Native myocardial T1 mapping: influence of spatial resolution on quantitative results and reproducibility
Introduction
Magnetic resonance imaging (MRI) is an indispensable diagnostic tool for diagnosis and follow-up of numerous cardiac diseases. Myocardial mapping techniques can be used to quantitatively assess alterations in myocardial tissue properties (1,2). An increase in native T1 values is typically observed in cardiac amyloidosis and pathologies associated with pathologies associated with myocardial fibrosis, scarring, or edema, including myocarditis, ischemic heart disease, as well as dilated and hypertrophic cardiomyopathies (3-8). Reduced native T1 is characteristic of Fabry disease and iron overload.
Previous studies demonstrated high test-retest reproducibility of T1 mapping as long as identical acquisition parameters are used (9,10). Despite high importance of acquisition settings, optimal spatial resolution for myocardial mapping, however, is not clearly defined. Current guidelines recommend “adequate” spatial resolution and slice thickness to avoid partial volume effects between left ventricular (LV) blood pool and myocardium (11,12). Most published studies on myocardial T1 mapping used in-plane resolutions of 1.4–2.0 mm with 8 mm being the predominantly used slice thickness (13).
Myocardial mapping is most commonly acquired in end-diastole to minimize cardiac motion. The acquisition window for T1 mapping within the cardiac cycle is about 200–250 ms for most standard sequences (14). With increasing heart rate, the diastolic phase decreases making rendering the techniques more susceptible to cardiac motion artifacts such as blurring. For this reason, T1 mapping techniques with lower spatial resolution were designed for patients with higher heart rate. The lower spatial resolution of these sequences allows for shorter acquisition window within the brief end-diastolic phase in these patients. Following this rationale many centers use higher spatial resolution as standard in patients heart rates ≤90 bpm and lower spatial resolution for heart rates >90 bpm (12).
However, it is still unclear how spatial resolution affects relaxation times and their reproducibility in native myocardial T1 mapping. Therefore, this study aims to evaluate the influence of spatial resolution on quantitative results and reproducibility of native myocardial T1 mapping. We present this article in accordance with the STROBE reporting checklist (available at https://qims.amegroups.com/article/view/10.21037/qims-23-943/rc).
Methods
Ethical approval, study design and patient selection
This cross-sectional study with prospective data was approved by the responsible Institutional Review Board of the University Medical Center Rostock and was conducted in accordance with the Declaration of Helsinki (as revised in 2013). Participants were recruited within the staff and students of the University Medical Center. Prior to enrollment, written informed consent was obtained from all participants. A sample size of 50 individuals was chosen following recommendations for reference value acquisitions in native mapping (15). We included healthy adult volunteers. Exclusion criteria were coronary artery disease, cardiomyopathies, myocarditis, arrhythmias or any other pathology of the heart, arterial hypertension, diabetes mellitus, stroke, respiratory or pulmonary disease (chronic respiratory disease, pulmonary embolism, pulmonary hypertension) chronic kidney disease, any malignant or rheumatic disease, possible or known pregnancy, MR-incompatible implants, and claustrophobia.
Four predefined age groups were chosen (≤34, 35–44, 45–54, and ≥55 years and at least three individuals of each gender was recruited for every group) to assure a broad age distribution among the participants. Information about age, weight and height and cardiovascular risk factors were collected. The questionnaire for risk factors included: dyslipidemia (at least one abnormal value of blood lipid markers, n=6), smoking (current or past, n=20), and family history of ischemic heart disease (n=3). The MRI recorded heart rate ranged from 55 to 99 bpm.
MRI technique
Both MRI investigations were done on the same day. After the first scan, the volunteer was taken out of the scanner and returned back into the scanner with at least 20-minute break in between. All MRI examinations were performed on a 1.5 T MRI system (Avantofit, Siemens Healthineers, Erlangen, Germany). 4- and 2-chamber views in steady-state free precession were included in the MRI protocols. For T1 mapping in high and low spatial resolution, three short-axis slices (basal, midventricular, and apical position) were acquired in a of the left ventricle. Prospective electrocardiography (ECG)-triggering was used with diastolic image acquisition.
A 5(3)3 MOLLI technique was used for native T1 mapping. We used the default settings as recommended by the manufacturer for heart rates ≤90 bpm (high-resolution) and >90 bpm (low-resolution). For the low-resolution acquisitions, in-plane resolution was 1.875 mm × 1.875 mm, field of view (FOV) 360 mm × 307 mm, image pixel matrix 192×164 and slice thickness 5 mm. Echo time (TE) was 1.21 ms and the flip angle 35°. For the high-resolution acquisition, the in-plane resolution was 1.406 mm × 1.406 mm, FOV 360 mm × 306 mm, image pixel matrix 256×218, and slice thickness 8 mm. TE was 1.13 ms and the flip angle was 35°. To avoid wrap around artefacts the FOV was occasionally adjusted to 380 mm for larger patients.
Image and T1 mapping analysis
Cvi42 (Version 5.11, Circle Cardiovascular Imaging Inc., Calgary, Canada) was used for the post-processing of the scanner generated T1 maps. The assessment of native T1 values was performed by one trained observer (initials blinded) using scanner-generated T1 maps of the three short-axis slices mentioned before. The observer was blinded to demographic parameters. Blinding to spatial resolution was not possible due to the difference in image impression. When assessing the retest images, the observer was blinded to the results of the first round.
Image quality of scanner generated maps was confirmed as sufficient for quantitative analysis in all participants. T1 curve fits were not assessed, since we used scanner generated maps. The executed steps for the analysis followed recommendations for mapping analysis (15) and were already described previously (10). Global T1 relaxation times were assessed as mean (± standard deviation) by taking the average over the entire circular regions of interest of all 3 slices. Furthermore T1 relaxation times were evaluated for each myocardial segment [1–16] following the standard AHA 16-segment model (16) as well as per-slice values (basal, mid-ventricular and apical).
A part of our data (T1 in high-resolution) has already been published to compare reproducibility in combination with T2 values (10). However, the differences and reproducibility within T1 time in high and low-resolution were not compared.
Statistical analysis
Global quantitative results of T1 mapping were compared between high- and low-resolution acquisitions using paired t-test, after data were checked for normal distribution visually and by Shapiro-Francia W’ test. Sub-analyses were performed for women and men, as well as normal-weight [body mass index (BMI) <25 kg/m2] and overweight (BMI ≥25 kg/m2) individuals. Additionally, participants were divided into three groups according to heart rate (<67, 67–77, and >77 bpm).
Intra-class correlation coefficient (ICC) and Bland-Altman statistics (absolute differences as means ± SD) were used for assessing test-retest reproducibility. ICCs were interpreted with: <0.50: poor, 0.50–0.75: moderate, 0.75–0.90: good, >0.90: excellent (95% confidence intervals calculated from two-way random-effects models) (17).
AHA segments were grouped by slice position (basal segments: 1–6, midventricular: segments 7–12, apical: segments 13–16) and anatomical region (interventricular septum: segments 2, 3, 8, 9, 14; anterior wall: segments 1, 7, 13; lateral wall: segments: 5, 6, 11, 12, 16 and inferior wall: segments 4 ,10, 15) Reproducibility was calculated for individual AHA segments and every group. ICCs were compared between segments or groups using the F-test. A two-sided P value of <0.05 was considered statistically significant. For the comparison of T1 values for each of the 16 myocardial segments, Bonferroni correction was performed to account for multiple testing. Thus, a P value of <0.05/16=0.003 was considered to indicate statistical significance for the per-segment analysis. Statistical analyses were performed using Stata 16.1 (Stata Corporation, College Station, TX, USA). The Python libraries Matplotlib version 3.5.3 as well as Numpy version 1.23.5 (for polar map figures).
Results
Study participants
The study cohort consisted of 50 adult individuals (29 women) with mean age of 39.4±13.7 years. Participants were predominantly of normal weight (76%) with a mean BMI of 23.9±4.0 kg/m2. The mean heart rate was 72.2±10.5 beats per minute. Demographic parameters are summarized in Table 1.
Table 1
Characteristics | All subjects (N=50) | Women (N=29) | Men (N=21) |
---|---|---|---|
Age (years) | 39.4±13.7 | 40.9±14.0 | 37.3±13.2 |
Height (cm) | 175.4±9.5 | 169.3±5.8 | 183.8±6.8 |
Weight (kg) | 73.8±15.7 | 66.7±12.6 | 83.6±14.5 |
BMI (kg/m2) | 23.9±4.0 | 23.3±4.2 | 24.7±3.7 |
Heart rate (bpm) | 72.2±10.5 | 74.1±9.4 | 69.4±11.5 |
Data is shown as mean ± standard deviation. BMI, body mass index.
Influence of spatial resolution on global quantitative results
Mean global native T1 values for high- and low-resolution are shown in Table 2. There was no significant difference between global quantitative results acquired with high- vs. low-resolution T1 mapping (1,027±29 vs. 1,026±27 ms, P=0.646). This was observed for both women (1,039±25 vs. 1,036±23 ms, P=0.185) and men (1,009±25 vs. 1,011±27 ms, P=0.502). Physiological gender differences with higher T1 mapping values in women compared to men were consistently found in both high- and low-resolution acquisitions. In BMI sub-analysis, spatial resolution had no significant impact on native myocardial T1 relaxation times for normal weight and overweight individuals. In heart rate sub-analysis, T1 values were significantly higher in high-resolution acquisitions compared to low-resolution in intermediate heart rates between 67–77 bpm (P<0.004) while no differences were found for lower or higher heart rates. Global native T1 relaxation times stratified by gender and heart rate in low- and high-resolution are displayed in Figure 1.
Table 2
Subgroup | N | Global T1 values high-resolution (ms) | Global T1 values low-resolution (ms) | P value |
---|---|---|---|---|
All subjects | 50 | 1,027±29 | 1,026±27 | 0.646 |
Gender | ||||
Women | 29 | 1,039±25 | 1,036±23 | 0.185 |
Men | 21 | 1,009±25 | 1,011±27 | 0.502 |
BMI, kg/m2 | ||||
<25 | 38 | 1,027±29 | 1,025±27 | 0.159 |
≥25 | 12 | 1,025±28 | 1,029±29 | 0.553 |
Heart rate, bpm | ||||
<67 | 18 | 1,023±35 | 1,025±32 | 0.523 |
67–77 | 16 | 1,029±29 | 1,023±30 | 0.004 |
>77 | 16 | 1,028±21 | 1,030±19 | 0.669 |
Data is shown as mean ± standard deviation. BMI, body mass index.

Influence of spatial resolution on the reproducibility of global quantitative results
Results of ICC analyses for high- and low-resolution global T1 values stratified by gender, BMI, and heart rate are summarized in Table 3. Overall reproducibility of global T1 values was significantly better with low-resolution compared to high-resolution (ICC: 0.95 vs. 0.88 respectively, P=0.003). In Figure 2, the Bland-Altman plots of native myocardial T1 relaxation times shows a wider limit of agreement (LoA, 95%) for high-resolution than for low-resolution. The LoA were −34 to 39 ms for high-resolution vs. −24.4 to 23 ms absolute for low-resolution.
Table 3
Subgroup | N | Reproducibility of global T1 high-resolution | Reproducibility of global T1 low-resolution | P value |
---|---|---|---|---|
All subjects | 50 | 0.88 (0.80–0.93) | 0.95 (0.90–0.97) | 0.003 |
Gender | ||||
Women | 29 | 0.71 (0.39–0.86) | 0.91 (0.82–0.96) | 0.001 |
Men | 21 | 0.96 (0.90–0.98) | 0.95 (0.88–0.98) | 0.666 |
BMI, kg/m2 | ||||
<25 | 38 | 0.92 (0.85–0.96) | 0.96 (0.92–0.98) | 0.029 |
≥25 | 12 | 0.77 (0.19–0.93) | 0.91 (0.69–0.97) | 0.064 |
Heart rate, bpm | ||||
<67 | 18 | 0.98 (0.94–0.99) | 0.97 (0.92–0.99) | 0.758 |
67–77 | 16 | 0.88 (0.65–0.96) | 0.94 (0.83–0.98) | 0.087 |
>77 | 16 | 0.53 (0.00–0.83) | 0.88 (0.68–0.96) | 0.004 |
Data are shown as ICC (95% CI) from two-way random-effects models. P value from F-test whether low-resolution ICC equals high-resolution ICC value. ICC, intraclass correlation coefficients; BMI, body mass index.
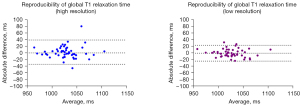
For women, reproducibility of T1 results was significantly better with low-resolution than with high-resolution (ICC: 0.91 vs. 0.71, P=0.001). No significant differences in reproducibility were observed in men. Normal-weight individuals (BMI <25 kg/m2) had significantly better T1 reproducibility with low-resolution compared to high-resolution (ICC: 0.96 vs. 0.92 respectively, P=0.029). For low heart rates <67 bpm, both high- and low-resolution acquisitions showed excellent reproducibility with no significant differences. A trend for better reproducibility was observed in intermediate heart rates (ICC: 0.88 vs. 0.94, P=0.087) and a clear benefit of lower resolution scanning was observed in faster heart rates (>77 bpm, 0.88 vs. 0.53, P=0.004).
Regional quantitative results by AHA segments
Mean values for T1 mapping results for high- and low-resolution separated by AHA segments are displayed in Figure 1. Apical segments (13-16) had slightly higher values and variability compared to other segments. This effect could be observed for both high- and low-resolution T1 acquisitions. Mean segmental T1 relaxation times ranged from 986 ms (±33 ms) to 1,095 ms (±84 ms) for high-resolution and from 991 ms (±39 ms) to 1,081 ms (±41 ms) for low-resolution imaging. For segments 8, 9, 13 and 16 the difference between high- and low-resolution results was statistically significant (P<0.001, Table 4). Figure 3 shows an example of native T1 myocardial maps for high- and low-resolution and both cMR examination of one volunteer.
Table 4
Segment No. | T1 low-resolution | T1 high-resolution | P value |
---|---|---|---|
1 | 1,008±34 | 1,000±37 | 0.179 |
2 | 1,035±25 | 1,026±30 | 0.004 |
3 | 1,025±26 | 1,025±28 | 0.850 |
4 | 1,020±39 | 1,023±35 | 0.613 |
5 | 997±35 | 987±33 | 0.034 |
6 | 1,005±33 | 994±31 | 0.027 |
7 | 1,025±36 | 1,028±46 | 0.509 |
8 | 1,031±27 | 1,019±25 | <0.001 |
9 | 1,035±30 | 1,024±30 | <0.001 |
10 | 1,021±30 | 1,019±35 | 0.569 |
11 | 1,013±39 | 1,001±44 | 0.012 |
12 | 1,032±38 | 1,026±44 | 0.255 |
13 | 1,041±42 | 1,076±67 | <0.001 |
14 | 1,078±75 | 1,075±65 | 0.680 |
15 | 1,033±49 | 1,050±80 | 0.047 |
16 | 1,042±56 | 1,094±85 | <0.001 |
T1 high and T1 low values are shown as mean ± standard deviation for each AHA segment. P values are from two-sided paired t-test. AHA, American Heart Association.
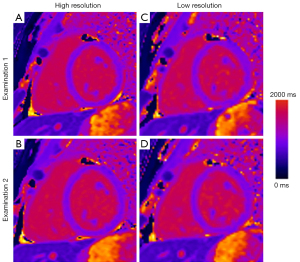
Reproducibility of regional quantitative results by AHA segments
Figure 4 visualizes ICC values of AHA segments for T1 mapping results in high- and low-resolution. T1 values in high-resolution showed good to excellent ICCs (ICC ≥0.75) in all segments except for moderate ICCs in segments 1,13 and 4 and poor ICCs in segments 5 and 6. Low-resolution T1 mapping results showed good to excellent ICCs in all segments except for segment 3, 4, 6 and 11 (moderate ICC: 0.50–0.75).
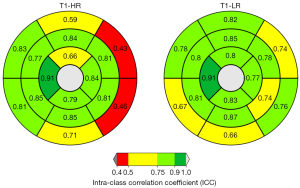
Reproducibility of regional quantitative results by slice position and anatomical region
Intraclass correlation analysis shows good to excellent results in T1 high and T1 low-resolution mapping by slice position and anatomical region (Table 5). T1 low-resolution data showed an overall higher ICCs, ranging from 0.87 to 0.92, compared to the high- resolution data (ICC: 0.81–0.91). Regional T1 values for basal (ICC: 0.81 vs. 0.89, P=0.023) and apical slices (ICC: 0.86 vs. 0.92, P=0.024) were significantly more reproducible with low-resolution compared to high-resolution acquisitions with no differences for midventricular slices (ICC: 0.91 vs. 0.92, P=0.402). Regarding the anatomical region, the anterior wall showed a non-significant trend for more reproducibility with lower-resolution mode compared to high-resolution mode (ICC: 0.81 vs. 0.88, P=0.050) with no difference in other regions of the myocardium (Table 5).
Table 5
Subgroup | Reproducibility of regional T1 high-resolution | Reproducibility of regional T1 low-resolution | P value |
---|---|---|---|
Slice position | |||
Basal slice | 0.81 (0.65–0.89) | 0.89 (0.81–0.94) | 0.023 |
Midventricular slice | 0.91 (0.84–0.95) | 0.92 (0.85–0.95) | 0.402 |
Apical slice | 0.86 (0.76–0.92) | 0.92 (0.86–0.96) | 0.024 |
Anatomical region | |||
Anterior wall | 0.81 (0.67–0.89) | 0.88 (0.79–0.93) | 0.050 |
Septum | 0.90 (0.82–0.94) | 0.92 (0.86–0.95) | 0.238 |
Inferior wall | 0.86 (0.76–0.92) | 0.86 (0.75–0.92) | 0.488 |
Lateral wall | 0.86 (0.76–0.92) | 0.87 (0.78–0.93) | 0.348 |
Data are shown as ICC (95% CI) from two-way random-effects models. P value from F-test whether low-resolution ICC equals high-resolution ICC value. ICC, intraclass correlation coefficients.
Discussion
In this prospective study including 50 healthy individuals, we evaluated the influence of spatial resolution on native myocardial T1 mapping results and their reproducibility performed at 1.5 T.
There were no systematic differences between spatial resolution on global native T1 values. No reliable trends could be observed in sub-group analyses by demographic data. A statistically significant difference between data from high- and low-resolution acquisitions was only found for individuals with intermediate heart rates (67–77 bpm). Since no differences were found overall and for lower and higher heart rates, the results for the sub-group with intermediate heart rate might be caused by the small number of individuals in each subgroup. The difference was also too small to be biologically meaningful (1,029 vs. 1,023 ms, relative difference 0.6%).
Previous investigations found good reproducibility of native global myocardial T1 relaxation times (9,10,18). Our present study goes beyond previously published data as we compare native T1 mapping acquired with lower and higher resolution. The overall reproducibility of global native T1 values was significantly better for the low-resolution acquisition. On subgroup analysis, low-resolution mode showed significantly improved reproducibility for heart rates >77 bpm. The lower spatial resolution allows for a shorter acquisition window in the end-diastolic phase. In higher heart rates, this becomes increasingly important to avoid blurring from cardiac motion as diastole shortens. Supporting this explanation, we found no difference in reproducibility for lower heart rates (<67 bpm) but a trend favoring lower resolution in intermediate (67–77 bpm) heart rates. Before performing this study, it was our institutional practice to use lower resolution only in very high heart rates (>90 bpm) as it was also the recommendation by the manufacturer. However, based on our findings, we believe it is prudent to use lower spatial resolution for heart rates >67 bpm if native T1 mapping is performed using a MOLLI 5(3)3 sequence on a 1.5 T system.
Interestingly, our subgroup analysis showed superior reproducibility when using lower spatial resolution for women but not for men, and for normal weight but not for overweight individuals. The latter results are probably caused by an uneven distribution of participants. Due to the small number of overweight individuals in our study, the difference in reproducibility did not reach statistical significance. To verify our findings, studies in larger cohorts are needed.
In contrast, the difference by gender is significant and thus should considered meaningful. For men, both lower and higher resolution had excellent reproducibility (ICC: 0.96 vs. 0.95). For women, reproducibility was excellent (0.91) for lower resolution but significantly worse (0.71, P=0.001) for higher spatial resolution. Myocardial volumes, mass, and thickness are lower in women than in men. Therefore, we expected better results in women with high-resolution mapping because partial volume effects can be reduced by more detailed delineation of left ventricle myocardium. However, this was not the case as the results of our analysis point in the opposite direction with a benefit of lower spatial resolution specifically in women. There was a difference in heart rates between women and men with a higher mean heart rate in women (74 bpm) compared to men (69 bpm). This might explain the selective benefit of lower-resolution scanning in women as lower-resolution acquisition is beneficial in heart rates >67 bpm. Certainly, this aspect warrants further investigation as different acquisition parameters may be optimal for women and men.
It is noticeable that variability of native T1 mapping values was higher in apical segments and T1 time in these segments was elevated compared to other areas. This was previously observed by us (10) and others (19,20) and can now also be transferred to the T1 time in low-resolution. Thus, this deviation might be not sequence-related and more likely caused by biological factors or partial volume effects in thinned wall structures.
Reproducibility of regional native T1 values in basal and apical slices was significantly better with low-resolution than with high-resolution. No significant differences were found for mid-ventricular slices. These findings might be explained by the longitudinal shortening of the left ventricle during the cardiac cycle, which leads to more movement in the apical and basal compared to the midventricular regions (20,21). Faster image acquisition in low-resolution mode therefore provides better reproducibility in these areas, since they are more susceptible to cardiac motion artefacts than mid-ventricular slices. Additionally, increased image noise with higher resolution may play a role for the inferior reproducibility of T1 values in some subgroups.
Several limitations of our study should be noted. We examined a cohort of healthy volunteers without any cardiovascular diseases. Consequently, cardiac morphology, mass, and volumes were within physiological range without pathological changes. Therefore, the reproducibility of native T1 mapping in pathological conditions cannot be deduced with certainty. In particular, we cannot exclude that there may be a benefit for higher spatial resolution in pathologies with thinned myocardium such as dilated cardiomyopathy. Our data was examined by one observer with good qualification and good experience using specific commercial software. A previous study has shown relevant inter-observer variability for T1 mapping (22). Our study was performed at 1.5T since this is the predominant field strength used in routine clinical cardiac MRI. The results may not apply to cardiac MRI performed at higher field strengths. Only native studies were performed, so we cannot make any statements about post-contrast T1 maps. Further studies are necessary whether post-contrast maps show similar results. We compared a 5 mm slice with a 8 mm slice and did not exhaust the range for high and low-resolution. However, these are the usual differences and relevant for clinical routine.
Conclusions
In conclusion, global quantitative results of native myocardial T1 mapping in 1.5 T do not differ systematically between lower (1.9 mm × 1.9 mm) and higher (1.4 mm × 1.4 mm) in-plane resolution in healthy volunteers. Test-retest reproducibility of regional T1 in basal and apical slices is superior for lower compared to higher in-plane resolution. Superior reproducibility of global T1 values was found for lower resolution especially in women and higher heart rates. Based on our data, we recommend a spatial resolution on the order of 1.9 mm × 1.9 mm for native myocardial T1 mapping using a MOLLI 5(3)3 sequence at 1.5 T especially in these groups. Since the reproducibility does not differ significantly between high and low-resolution in healthy volunteers, it can be evaluated to choose low-resolution as the default setting for all patients.
Acknowledgments
Funding: None.
Footnote
Reporting Checklist: The authors have completed the STROBE reporting checklist. Available at https://qims.amegroups.com/article/view/10.21037/qims-23-943/rc
Conflicts of Interest: All authors have completed the ICMJE uniform disclosure form (available at https://qims.amegroups.com/article/view/10.21037/qims-23-943/coif). F.G.M. received a research grant from GE HealthCare and speaker honoraria from GE HealthCare, Circle Cardiovascular Imaging and Bayer Vital. The other authors have no conflicts of interest to declare.
Ethical Statement: The authors are accountable for all aspects of the work in ensuring that questions related to the accuracy or integrity of any part of the work are appropriately investigated and resolved. The study was designed as a prospective, single-center cross-sectional study and approved by the responsible Institutional Review Board of the University Medical Center Rostock and was conducted in accordance with the Declaration of Helsinki (as revised in 2013). Prior to enrollment, written informed consent was obtained from all participants.
Open Access Statement: This is an Open Access article distributed in accordance with the Creative Commons Attribution-NonCommercial-NoDerivs 4.0 International License (CC BY-NC-ND 4.0), which permits the non-commercial replication and distribution of the article with the strict proviso that no changes or edits are made and the original work is properly cited (including links to both the formal publication through the relevant DOI and the license). See: https://creativecommons.org/licenses/by-nc-nd/4.0/.
References
- Jellis CL, Kwon DH. Myocardial T1 mapping: modalities and clinical applications. Cardiovasc Diagn Ther 2014;4:126-37. [Crossref] [PubMed]
- Hamdy A, Kitagawa K, Ishida M, Sakuma H. Native Myocardial T1 Mapping, Are We There Yet? Int Heart J 2016;57:400-7. [Crossref] [PubMed]
- Mewton N, Liu CY, Croisille P, Bluemke D, Lima JA. Assessment of myocardial fibrosis with cardiovascular magnetic resonance. J Am Coll Cardiol 2011;57:891-903. [Crossref] [PubMed]
- Dall'Armellina E, Piechnik SK, Ferreira VM, Si QL, Robson MD, Francis JM, Cuculi F, Kharbanda RK, Banning AP, Choudhury RP, Karamitsos TD, Neubauer S. Cardiovascular magnetic resonance by non contrast T1-mapping allows assessment of severity of injury in acute myocardial infarction. J Cardiovasc Magn Reson 2012;14:15. [Crossref] [PubMed]
- Messroghli DR, Walters K, Plein S, Sparrow P, Friedrich MG, Ridgway JP, Sivananthan MU. Myocardial T1 mapping: application to patients with acute and chronic myocardial infarction. Magn Reson Med 2007;58:34-40. [Crossref] [PubMed]
- Ferreira VM, Piechnik SK, Dall'Armellina E, Karamitsos TD, Francis JM, Choudhury RP, Friedrich MG, Robson MD, Neubauer S. Non-contrast T1-mapping detects acute myocardial edema with high diagnostic accuracy: a comparison to T2-weighted cardiovascular magnetic resonance. J Cardiovasc Magn Reson 2012;14:42. [Crossref] [PubMed]
- Kedzierski M. Influenza: causes and incidence. Nurs Stand 1991;5:35-8. [Crossref] [PubMed]
- Taylor AJ, Salerno M, Dharmakumar R, Jerosch-Herold M. T1 Mapping: Basic Techniques and Clinical Applications. JACC Cardiovasc Imaging 2016;9:67-81. [Crossref] [PubMed]
- Piechnik SK, Ferreira VM, Lewandowski AJ, Ntusi NA, Banerjee R, Holloway C, Hofman MB, Sado DM, Maestrini V, White SK, Lazdam M, Karamitsos T, Moon JC, Neubauer S, Leeson P, Robson MD. Normal variation of magnetic resonance T1 relaxation times in the human population at 1.5 T using ShMOLLI. J Cardiovasc Magn Reson 2013;15:13. [Crossref] [PubMed]
- Böttcher B, Lorbeer R, Stöcklein S, Beller E, Lang CI, Weber MA, Meinel FG. Global and Regional Test-Retest Reproducibility of Native T1 and T2 Mapping in Cardiac Magnetic Resonance Imaging. J Magn Reson Imaging 2021;54:1763-72. [Crossref] [PubMed]
- Moon JC, Messroghli DR, Kellman P, Piechnik SK, Robson MD, Ugander M, Gatehouse PD, Arai AE, Friedrich MG, Neubauer S, Schulz-Menger J, Schelbert EB. Myocardial T1 mapping and extracellular volume quantification: a Society for Cardiovascular Magnetic Resonance (SCMR) and CMR Working Group of the European Society of Cardiology consensus statement. J Cardiovasc Magn Reson 2013;15:92. [Crossref] [PubMed]
- Kellman P, Hansen MS. T1-mapping in the heart: accuracy and precision. J Cardiovasc Magn Reson 2014;16:2. [Crossref] [PubMed]
- Kim PK, Hong YJ. Im DJ, Suh YJ, Park CH, Kim JY, Chang S, Lee HJ, Hur J, Kim YJ, Choi BW. Myocardial T1 and T2 Mapping: Techniques and Clinical Applications. Korean J Radiol 2017;18:113-31. [Crossref] [PubMed]
- Rumac S, Pavon AG, Hamilton JI, Rodrigues D, Seiberlich N, Schwitter J, van Heeswijk RB. Cardiac MR fingerprinting with a short acquisition window in consecutive patients referred for clinical CMR and healthy volunteers. Sci Rep 2022;12:18705. [Crossref] [PubMed]
- Messroghli DR, Moon JC, Ferreira VM, Grosse-Wortmann L, He T, Kellman P, Mascherbauer J, Nezafat R, Salerno M, Schelbert EB, Taylor AJ, Thompson R, Ugander M, van Heeswijk RB, Friedrich MG. Clinical recommendations for cardiovascular magnetic resonance mapping of T1, T2, T2* and extracellular volume: A consensus statement by the Society for Cardiovascular Magnetic Resonance (SCMR) endorsed by the European Association for Cardiovascular Imaging (EACVI). J Cardiovasc Magn Reson 2017;19:75. [Crossref] [PubMed]
- Cerqueira MD, Weissman NJ, Dilsizian V, Jacobs AK, Kaul S, Laskey WK, Pennell DJ, Rumberger JA, Ryan T, Verani MS. Standardized myocardial segmentation and nomenclature for tomographic imaging of the heart. A statement for healthcare professionals from the Cardiac Imaging Committee of the Council on Clinical Cardiology of the American Heart Association. Circulation 2002;105:539-42. [Crossref] [PubMed]
- Koo TK, Li MY. A Guideline of Selecting and Reporting Intraclass Correlation Coefficients for Reliability Research. J Chiropr Med 2016;15:155-63. [Crossref] [PubMed]
- Messroghli DR, Plein S, Higgins DM, Walters K, Jones TR, Ridgway JP, Sivananthan MU. Human myocardium: single-breath-hold MR T1 mapping with high spatial resolution--reproducibility study. Radiology 2006;238:1004-12. [Crossref] [PubMed]
- Granitz M, Motloch LJ, Granitz C, Meissnitzer M, Hitzl W, Hergan K, Schlattau A. Comparison of native myocardial T1 and T2 mapping at 1.5T and 3T in healthy volunteers: Reference values and clinical implications. Wien Klin Wochenschr 2019;131:143-55. [Crossref] [PubMed]
- von Knobelsdorff-Brenkenhoff F, Prothmann M, Dieringer MA, Wassmuth R, Greiser A, Schwenke C, Niendorf T, Schulz-Menger J. Myocardial T1 and T2 mapping at 3 T: reference values, influencing factors and implications. J Cardiovasc Magn Reson 2013;15:53. [Crossref] [PubMed]
- Støylen A, Dalen H, Molmen HE. Left ventricular longitudinal shortening: relation to stroke volume and ejection fraction in ageing, blood pressure, body size and gender in the HUNT3 study. Open Heart 2020;7:e001243. [Crossref] [PubMed]
- Roy C, Slimani A, de Meester C, Amzulescu M, Pasquet A, Vancraeynest D, Vanoverschelde JL, Pouleur AC, Gerber BL. Age and sex corrected normal reference values of T1, T2 T2* and ECV in healthy subjects at 3T CMR. J Cardiovasc Magn Reson 2017;19:72. [Crossref] [PubMed]