Regional assessment of aortic valve calcification using topographic maps in contrast-enhanced CT: in-vivo sex and severity-based differences in calcific presentation
Introduction
Aortic valve calcification (AVC) strongly influences native and artificial valve behavior and is a key feature involved in the development and progression of aortic stenosis (AS) (1). AVC is presented in aortic sclerosis, a disease in which the valve leaflets begin to thicken and develop regions of focal calcification, and over time can progress to AS (1,2). AS is characterized as a gradual decrease of the valve orifice area leading to left ventricular outflow tract (LVOT) obstruction and left ventricular (LV) hypertrophy due to an increased afterload (3). Prior to the recent introduction of the minimally invasive transcatheter aortic valve replacement (TAVR), surgical aortic valve (AV) replacement was the only possible intervention for severe AS. As TAVR is becoming more frequent (4), the procedure is continuously advancing with improvements in valve and delivery system designs, as well as increased clinician experience (4). It is therefore critical that during procedural planning, valuable prognostic information is collected to help plan and optimize patient-specific interventions (5). Primarily, detailed calcification assessment must be included in this process (5,6) as AVC is associated with a variety of peri-procedural complications (5,7). In the context of TAVR, the new valve may be restricted from expanding completely due to native calcification. The presence of leaflet and/or LVOT calcification is a risk factor to paravalvular leakage surrounding the implant (8), annular rupture, aortic root (AR) injury or conduction abnormalities which may increase the risk of mortality (9,10).
Currently the only established method for assessing AVC quantitatively is via the Agatston method through non-contrast computed tomography (CT) images (11,12). Which was originally applied for quantifying coronary artery calcium (CAC) but has since been used to quantify cardiovascular calcification in various regions. In the case of the AV, it is measured at the cusp/leaflet region avoiding extra valvular calcium in the LVOT or the ascending aorta (11). Pixels above 130 Hounsfield unit (HU) are segmented and within each lesion in a 2D cross-section, the maximum HU is calculated and used to obtain a density weighting factor (DWF) which is multiplied by the 2D area of the lesion. The sum of this areas multiplied by DWF is then calculated to obtain the final Agatston score for the region of interest (11,12). It is primarily used as secondary diagnostic test for AS, when echocardiographic measures are discordant (6,11). However, this score has key limitations, it requires low resolution 2.5–3 mm axial views, which severely limits regional quantification of calcium burden (11,12). Furthermore, the lack of contrast precludes qualitative assessment of AV anatomy or valve planimetry (6). Both limitations motivate the design of new scoring method based on high resolution contrast CT images (13,14), especially in the context of TAVR, where accurate annular assessment using contrast CT is a necessity for procedural planning (6).
Previous studies (14-19) investigated simpler modifications to the standard Agatston technique when using contrast-enhanced CT images. These approaches were mainly motivated by the idea of using a new cut-off thresholding value, either fixed or dynamically determined based on luminal attenuation. In that regard, we previously (20) developed a method that does not explicitly rely on luminal attenuation. Instead, initial leaflet calcification regions are determined based on the intensity characteristics of a bounding AR segmentation. Using those initial conditions, an iterative region growing method adjusts the Hounsfield band for calcific detection gradually, until satisfying certain overlap criteria between calcific and non-calcific segments in the image. We were able to show strong correlation with gold standard non-contrast and echocardiographic based indices of AS severity (20). In this study we focused the study cohort on the tricuspid valve morphology and introduced a new anatomically based regional mapping scheme to provide a quantitative description of location, quantity, and average intensity of calcific deposition. The new topographic maps may provide a unique perspective for interpretation of calcific progression in terms of sex- and severity-based differences. To the best of our knowledge, this is the first study on in-vivo quantitative description of AV leaflet calcification using high resolution CT imaging.
Methods
We evaluated the proposed method on a cohort of patients diagnosed with AS. We compared the scores using the proposed method on the contrast-enhanced images to the standard scores produced for non-contrast (16,18,21) images that were scored using commercially available software (Calcium scoring application, Syngo.via; Siemens Healtheneers, Forchheim, Germany).
Study population
We retrospectively selected 178 patients diagnosed with AS from Hamilton General Hospital (Hamilton, Canada; between 2020 and 2022). Non-consecutive data collection included patients evaluated for TAVR with severe or non-severe AS diagnosed by 2D doppler echocardiography and who underwent both gated contrast and non-contrast CT within 3 months of the echocardiogram. Severity was determined by recommended thresholds for AV area, peak aortic jet velocity and mean valve pressure gradient (22). The study was conducted in accordance with the Declaration of Helsinki (as revised in 2013). The study was approved by the Hamilton integrated research ethics board (HiREB) and individual consent for this retrospective analysis was waived. The selections were done by operators blinded to the objectives and contents of this study. Clinical measurements were performed per relevant guidelines and regulations including guidelines of the American College of Cardiology and American Heart Association. Demographic and procedural data were collected from the patients’ medical records (see Table 1 for patient characteristics).
Table 1
Category | Value |
---|---|
Age (years), mean ± SD | 80.5±7.3 |
Sex, n (%) | |
Female | 80 (44.9) |
Male | 98 (55.1) |
AS severity, n (%) | |
Non severe | 49 (27.5) |
Severe | 129 (72.5) |
NYHA class, n (%) | |
I | 17 (9.6) |
II | 70 (39.3) |
III | 78 (43.8) |
IV | 13 (7.3) |
Coronary artery disease, n (%) | 85 (47.8) |
Hypertension, n (%) | 164 (92.1) |
Type 2 diabetes mellitus, n (%) | 74 (41.6) |
Dyslipidemia, n (%) | 132 (74.2) |
Atrial fibrillation, n (%) | 65 (36.5) |
SD, standard deviation; NYHA, New York Heart Association; AS, aortic stenosis.
CT acquisition
The patients underwent both contrast and non-contrast enhanced CT scans using GE Healthcare Discovery CT750 HD Scanner 64 slice 40 mm detector. Contrast CT images were acquired using retrospective gating without tube current modulation of the entire cardiac cycle using a slice thickness of 0.625 mm. Prospectively ECG gated non-contrast CT was performed in a sequential mode at 60–80% of the RR interval using a slice thickness of 3 mm (see Table S1 for CT acquisition parameters).
Computational analysis
Calcification detection
We developed a framework which consists of pipelines designed for the 3D visualization and quantification of calcific lesions using contrast-enhanced thoracic CT images (Figures 1-6; Tables 1-4). The proposed calcium detection method uses a novel segmentation scheme that is based on automatically detected local image features (Figure 2). The proposed method obviates the need for manual annotation of a region of interest in the ascending aorta or a predetermined minimum HU cutoff value. Our developed computational framework requires the field of view to be initially localized to the AV based on the standard assessment guidelines for TAVR planning (Figure 1A). A partial AR model is then automatically segmented (Figure 1B). Using the AR segmentation, principal directions and landmark points are generated semi-automatically (Figure 1A,1B). Using this initial set of parameters, a fully automatic detection scheme is designed to detect the maximal amount of calcific content regardless of the effect of the contrast agent on luminal attenuation (Figure 2). This is accomplished by measuring the false positive rate (FPR) between iterative decrements of HU threshold reconstructions of calcific segments and the partial AR model (Figure 2).
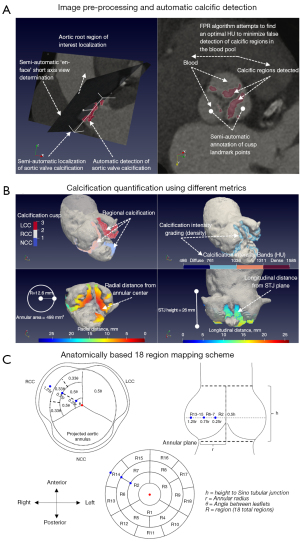
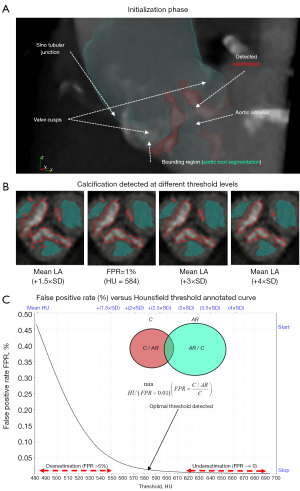
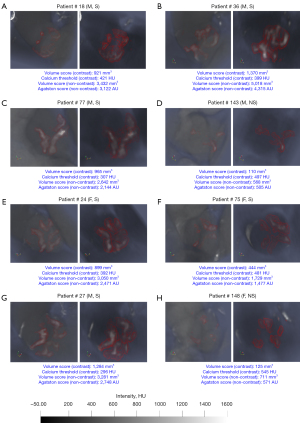
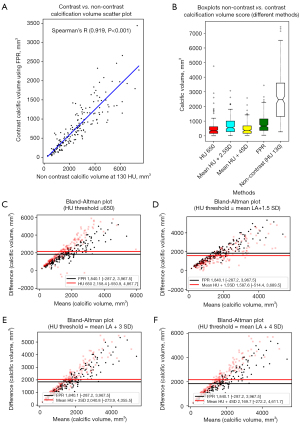
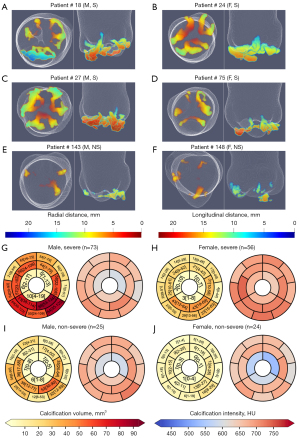
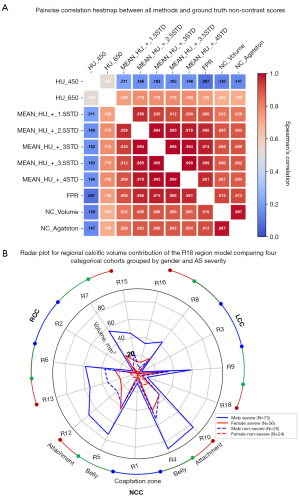
Table 2
Parameter | Female | Male | |||
---|---|---|---|---|---|
Non (n=24) | Severe (n=56) | Non (n=25) | Severe (n=73) | ||
Contrast CT calcific volume (mm3) | 148 [115–209] | 473 [374–759] | 538 [302–737] | 1,133 [780–1,483] | |
Indexed contrast CT calcific volume (mm3/cm2) | 39 [26–57] | 125 [84–215] | 114 [61–143] | 215 [172–302] | |
FPR derived calcific threshold (HU) | 462 [423–533] | 443 [391–591] | 424 [348–491] | 375 [307–415] | |
Non-contrast calcific volume (mm3) | 834 [615–1,094] | 2,042 [1,434–2,878] | 1,716 [1,374–2,227] | 3,281 [2,672–4,363] | |
Non-contrast Agatston score (AU) | 707 [516–867] | 1,570 [1,197–2,205] | 1,445 [1,145–1,820] | 2,645 [2,094–3,460] | |
Contrast CT calcific volume | |||||
NCC (mm3) | 64 [26–102] | 209 [136–353] | 246 [123–312] | 449 [304–571] | |
RCC (mm3) | 36 [24–65] | 122 [80–253] | 114 [85–151] | 343 [210–483] | |
LCC (mm3) | 44 [29–96] | 153 [76–222] | 167 [113–239] | 300 [209–494] | |
Annular area (cm2) | 4.0 [3.3–4.5] | 4.1 [3.5–4.5] | 5.2 [4.6–5.6] | 5.1 [4.5–5.6] | |
Annular radius (mm) | 11.3 [10.2–12] | 11.4 [10.6–12] | 12.9 [12.1–13.3] | 12.7 [12.0–13.4] | |
STJ height (mm) | 19.7 [18–21] | 20.5 [19.1–22.5] | 23.6 [21–25.6] | 23.4 [21.1–25.5] |
All continuous parameters presented as median [interquartile range]. CT, computed tomography; FPR, false positive rate; HU, Hounsfield unit; NCC, non-coronary cusp; RCC, right coronary cusp; LCC, left coronary cusp; STJ, sino-tubular junction.
Table 3
Region | One-way ANOVA (non-parametric) (Dwass-Steel-Critchlow-Fligner pairwise comparisons) | ||||||
---|---|---|---|---|---|---|---|
F-NS vs. F-S | M-NS vs. M-S | F-S vs. M-S | F-NS vs. M-S | F-S vs. M-NS | F-NS vs. M-NS | ||
R1 | 0.197 | 0.316 | 0.002 | <0.001 | 0.836 | 0.102 | |
R2 | 0.242 | 0.025 | 0.046 | <0.001 | 0.886 | 0.757 | |
R3 | 0.172 | 0.363 | 0.054 | <0.001 | 0.976 | 0.105 | |
R4 | 0.006 | 0.036 | <0.001 | <0.001 | 0.681 | 0.003 | |
R5 | <0.001 | 0.126 | 0.006 | <0.001 | 0.982 | <0.001 | |
R6 | 0.003 | 0.136 | 0.006 | <0.001 | 0.887 | <0.001 | |
R7 | 0.01 | <0.001 | 0.003 | <0.001 | 0.121 | 0.912 | |
R8 | 0.008 | 0.173 | 0.113 | <0.001 | 0.991 | 0.203 | |
R9 | 0.096 | 0.261 | 0.023 | <0.001 | 0.947 | 0.113 | |
R10 | 0.052 | 0.002 | <0.001 | <0.001 | 0.949 | 0.264 | |
R11 | 0.002 | 0.03 | 0.041 | <0.001 | 0.603 | 0.356 | |
R12 | 0.003 | 0.008 | <0.001 | <0.001 | 0.874 | <0.001 | |
R13 | 0.007 | 0.027 | 0.005 | <0.001 | 1 | 0.068 | |
R14 | 0.057 | 0.099 | 0.161 | 0.001 | 0.826 | 0.447 | |
R15 | 0.002 | 0.023 | 0.001 | <0.001 | 0.979 | 0.026 | |
R16 | 0.271 | 0.054 | 0.107 | 0.003 | 0.816 | 0.855 | |
R17 | 0.392 | 0.298 | 0.969 | 0.179 | 0.515 | 0.994 | |
R18 | 0.242 | 0.987 | 0.997 | 0.345 | 0.955 | 0.688 |
Results of regional volume post-hoc pairwise comparisons between categories comparing sex and severity effect on regional calcific distribution, P values reported to three significant figures. R, region; F, female; M, male; S, severe; NS, non-severe.
Table 4
Paired samples t-test (Wilcoxon W) | Wilcoxon test statistic | P value | Mean difference | Standard error difference |
---|---|---|---|---|
NCC | ||||
Coaptation zone—R1 | ||||
Belly left—R4 | 184 | <0.001 | −52.182 | 4.33 |
Belly right—R5 | 339 | <0.001 | −42.766 | 4.77 |
Belly left—R4 | ||||
Attachment left—R10 | 8,985 | 0.056 | 6.946 | 4.04 |
Attachment mid—R11 | 10,466 | <0.001 | 15.868 | 4.85 |
Belly right—R5 | ||||
Attachment mid—R11 | 9,659 | 0.009 | 9.758 | 4.68 |
Attachment right—R12 | 9,077 | 0.079 | 4.172 | 3.99 |
RCC | ||||
Coaptation zone—R2 | ||||
Belly posterior—R6 | 734 | <0.001 | −33.118 | 3.57 |
Belly anterior—R7 | 739 | <0.001 | −30.991 | 3.43 |
Belly posterior—R6 | ||||
Attachment posterior—R13 | 8,640 | 0.264 | 2.969 | 4.58 |
Attachment mid—R14 | 12,006 | <0.001 | 16.889 | 3.84 |
Belly anterior—R7 | ||||
Attachment mid—R14 | 11,386 | <0.001 | 13.096 | 3.79 |
Attachment anterior—R15 | 9,823 | 0.007 | 7.308 | 3.59 |
LCC | ||||
Coaptation zone—R3 | ||||
Belly anterior—R8 | 1,400 | <0.001 | −24.08 | 3.15 |
Belly posterior—R9 | 830 | <0.001 | −37.433 | 4.08 |
Belly anterior—R8 | ||||
Attachment anterior—R16 | 7,829 | 0.848 | 0.464 | 3.87 |
Attachment mid—R17 | 8,967 | 0.082 | 4.597 | 4.17 |
Belly posterior—R9 | ||||
Attachment mid—R17 | 10,931 | <0.001 | 17.933 | 4.97 |
Attachment posterior—R18 | 11,546 | <0.001 | 20.779 | 4.77 |
Entire cohort was used in the analysis, pairs of adjacent regions in each leaflet cusp were used to evaluate statistical differences in regional volume across the valve surface. NCC, non-coronary cusp; RCC, right coronary cusp; LCC, left coronary cusp; R, region.
Assessment of the calcification detection method
Appropriate statistical tests were performed using Jamovi v.1.8. Summaries of the variables and tests performed in (Figures 4,6; Tables 2-4) are outlined as follows. Correlations and comparisons between the variables were performed using Spearman’s rank correlation test, Wilcoxon rank paired t-test, non-parametric One-way ANOVA (Kruskal-Wallis) followed by post-hoc Dwass-Steel-Critchlow-Fligner pairwise comparisons where applicable. Bland-Altman analysis of difference was used to compare the developed FPR method alongside the conventional methods based on a fixed HU threshold [650] or a mean HU attenuation at the ascending aorta (+1.5SD, +2.5SD, +3SD, +3.5SD, +4SD) (Figures 4,6A). Values were reported as median [25th–75th percentile], mean ± standard deviation or mean difference [lower limit of agreement, upper limit of agreement] where applicable. Finally, interobserver variability was measured using the intraclass correlation coefficient (ICC; two-way random agreement) for a sub cohort of 49 patients. Statistical significance was considered when the P value was less than 0.05. Detailed information regarding derivation and normalization of relevant parameters is outlined in Appendix 1 (section Normalization of the metrics).
Geometrical and structural map of detected calcification
Once the calcified voxels are detected with FPR, we generate density and topographic maps of both radial and longitudinal measurements for the detected calcific regions (Figure 1B). We developed an anatomical, 18 region mapping scheme (Figure 1C) based on the following standardized measurements: height to sino-tubular-junction, annular area derived radius and angles of the interleaflet triangle (Figure 1A). These measurements were used as distance thresholds (18 regions from coaptation zone to attachment) in order to quantify regional calcific volume and average Hounsfield intensity across the cohort (Figures 5,6B). Detailed information regarding methodology and theory is presented in Appendix 1, Figure S1 and Table S2.
Results
This study consisted of patients diagnosed with non-severe (49/178; 27.5%) and severe (129/178; 72.5%) AS. The cohort had an average age of 80.5±7.3 years and included males (98/178; 55.1%) and females (80/178; 44.9%). Baseline characteristics are presented in Table 1. Interobserver variability was assessed on a random sub-group of 49 patients using ICC (two-way random agreement) showing excellent reproducibility for the contrast CT calcific volume parameter (ICC: 0.95; 95% CI: 0.94–0.96; raters =2).
FPR method assessment
The contrast and non-contrast segmented calcium volumes were compared for the 8 representative patient samples in Figure 3. By focusing the field of view on the AV in both contrast and non-contrast images, we can qualitatively compare the patterns of calcification detected between the contrast (FPR method) and non-contrast (standard 130 HU). The detected calcification is identified with a red outline around the region of interest in each subplot (Figure 3). In general, we can observe a strong agreement of detected calcification between both images in terms of the shape and location. Additionally, calcification volume using the FPR method showed the best correlation against non-contrast volume and Agatston scores (r=0.919, P<0.001; r=0.913, P<0.001) in contrast-enhanced images (Figures 4A,6A). With regards to absolute volume of detected calcification, a large difference is observed. Contrast images consistently produces a lower total volume of detected calcification compared to the non-contrast images. Paired comparisons (Figures 3,4B; Table 2) showed significantly lower volume scores for the contrast images compared to non-contrast volume scores. This underestimation was observed for all methods evaluated against non-contrast (Figure 4B). Visual differences between detected calcific volumes using the proposed method and non-contrast images (130 HU threshold) are presented in (Figure 3). Given the systemic bias with non-contrast, we compared FPR with methods based on fixed HU or dynamic HU thresholds using luminal attenuation with Bland-Altman agreement plots (Figure 4C-4F), all against the same reference non-contrast volume scores. All evaluated methods showed a proportional bias that increases at higher calcium volumes when compared to non-contrast (Figure 4C,4D) with mean luminal attenuation +3SD being the closest method to the FPR (r=0.974; P<0.001) (Figure 6A). Moreover, comparative analysis showed the FPR method had the best overall performance in terms of both low and high calcification volumes with a mean bias (1,840.1) and an error at the low and high levels of agreement [−1.96SD: −287.2, +1.96SD: 3,967.5]. Compared to the fixed SD methods, we can clearly observe that at higher SD, bias increases at high level of agreement as the threshold becomes over-conservative in capturing calcific segments. Alternatively, at lower SD, bias increases at the low level of agreement as the determined threshold overestimates calcific segments.
Finally, we can also observe the behavior of the different methods on a representative severe AS patient in Figure 2B,2C with high thresholds (+3SD, +4SD) missing small calcific nodules and low thresholds (+1.5SD) merging large calcific regions between the leaflets.
We highlight that luminal attenuation derived methods can over or underestimate calcific deposition, especially in situations of high variance in the blood pool HU intensity distribution or low calcific densities in non-severe cases (16,19). Our FPR method can demonstrably compensate for these limitations, by iterative refinement of HU threshold based on an image specific FPR. This has implications for both a diagnostic use case of contrast-enhanced calcification score and accurate regional assessment of calcific nodules. Precise regional assessment cannot be performed in non-contrast CT due to low contrast and spatial resolution. This is critical as it may provide an incremental improvement over current qualitative criteria of calcification assessment in TAVR planning (6).
Geometry and structure of leaflet calcification in AS
Due to the complex morphology and structure of calcific presentation, the single value scores developed for CAC scoring (11,12) precludes determining the progression of calcific deposition in AS (23,24). The high contrast and spatial resolution in contrast images combined with a precise calcific detection method, provides a unique opportunity to examine the regional presentation of calcific deposition in AS. In that respect, we generated topographic distance maps for both radial and longitudinal distance relative to each patient’s AV dimensions (annular radius and STJ height) as presented in Figure 5A-5E. We arranged the results of 6 representative patients grouped by sex and AS severity. Furthermore, using the anatomical 18 region mapping scheme described in Figure 1C, we evaluated this regional presentation across the entire cohort (Figure 5G-5J) both in terms of calcific volume and average Hounsfield intensity in each region. Furthermore, we compared calcific volume contribution between the different groups as well as between the regions for the entire cohort (Figure 6B; Tables 3,4).
In terms of the regional presentation of calcification for each cusp (Figure 5), we observed four primary patterns of calcific distribution organized by severity and sex as follows:
- Severe: maximal calcific deposition in near the belly region of all leaflets followed by root attachment edges with significantly lower calcification near the coaptation zones (Figure 5A-5D,5G,5H; Table 4). In males the amount of calcification is significantly higher across most regions (Figure 6B, Table 3). While in females (Figure 5B,5D,5H), a lower calcific progression rate presents calcific arcs primarily near free edges of the leaflets that have not yet developed in rings at the fixed edge (Figure 6B; Table 3).
- Non-severe: maximal calcific deposition near sites of root attachment and belly, prominent near the non and left coronary cusps (Figure 5E,5F,5I,5J). At this stage calcification primarily presents as disconnected deposits with males presenting significantly higher calcific deposition near leaflet attachment edges between the non and right coronary leaflets than females (Figure 5F,5J,6BTable 3).
Overall, inter-region comparison (Figure 6B; Table 4) shows a radial spoke pattern of calcific progression that is clustered around the belly region of the leaflets, followed by the root attachment at both sides of the leaflets and significantly decreasing near the coaptation zone. In terms of scale, the non-coronary leaflet experiences the most calcific thickening followed by the right and then left coronary leaflet.
We remark that current in-vivo quantitative description of regional calcification is limited (7) and most previous studies relied on excised tissue analysis (23) or biomechanical simulated models (25,26). Our patient specific geometric model was designed to capture the local pattern of calcific deposition. Our results indicate that although calcific progression in each leaflet follows a particular pattern that agrees with previous literature, the rate of calcific progression is clearly asymmetric across the different leaflets. Furthermore, females experience significantly less calcific deposition within those patterns despite experiencing similar degrees of valve obstruction. This observation concurs with recent data (16,27,28), which may add further evidence that calcific thickening alone is not enough to cause significant valve obstruction and subsequent clinical symptoms of AS.
Discussion
AS is generally characterized as a gradual decrease of the valve orifice area leading to LVOT obstruction and later, LV hypertrophy due to an increased afterload. There are two primary pathways for AS development, calcific deposition, and rheumatic heart disease. Globally, calcific AS accounts for the majority of AS pathology particularly in developed countries with prevalence increasing with age. Accurate quantification of calcific burden is therefore critical in the AS diagnostic process, particularly in situations when standard diagnostic measures (e.g., echocardiography) are inconclusive (11).
Current techniques using contrast-enhanced imaging, which are based on luminal attenuation or a fixed HU threshold, have shown promise in terms of ease of use and reproducibility. Despite the benefits, these methods may underestimate calcification in the leaflets, especially if image noise or calcific deposits in the sino-tubular junction (STJ) plane are present. Additionally, these methods may be sensitive to interscan, inter-device and cohort selection, which may explain the wide variation of values used for calcium thresholding (14-19). Thus, we propose an approach that can dynamically adjust the threshold based on local image features. Finally, using high detail contrast imaging, presents a potential to move beyond the bulk volume or Agatston scores (13,16). New markers based on the regional patterns of calcification could be a valuable add-on to existing CT-TAVR guidance protocols, given that in current CT-TAVR (6) assessment, AS severity is already confirmed and valvular calcification is only described qualitatively to plan interventions. Another motivation for developing new markers for measuring calcific burden is the strong evidence that shape, position, and density distribution of calcification are all closely related to peri-procedural outcomes (6,7,29).
FPR method provides an attenuation stable calcific detection threshold
The key advantage of the FPR method is that by considering a shape overlap measure in guiding the optimization for calcium threshold detection, the method can better adapt to general fluctuations in the intraluminal contrast. Therefore, it can work equally well in both high and low calcific densities. The FPR method (Figure 2) relies on the automatic estimation of the FPR of detected contrast material (19) to guide the detection of an optimal threshold of calcific regions. This is based on the idea that the detection of scattered calcific lesions can be guided by the more reliable estimation of the larger AR model with well-defined boundaries. Indeed, the direct evaluation of AS scoring is currently based on non-contrast images (6,11). We showed that the volume scores derived using the proposed method had the strongest correlation with standardized non-contrast calcific scores (Figure 6A) and further demonstrated the limitations of fixed and luminal attenuation methods, particularly in either under or overestimation of calcific content at high or low calcific concentrations, respectively (Figure 4C-4F). This could be particularly important in improving the reproducibility of calcification assessment using contrast images in large, randomized cohorts, especially in females. Despite exhibiting significantly lower calcific deposition compared to males (Figure 5), females still present with symptoms of severe AS (27). In addition, a more precise calcific detection method can have added prognostic use for TAVR planning (6). By determining the regional effect of calcific deposition in periprocedural scenarios (6,7,29) (e.g., paravalvular leakage, left-bundle branch block).
A precise calcium detection method is necessary to accurately evaluate regional calcific distribution and hence understand the more likely pattern of progression. Furthermore, once a calcific threshold is identified, we can proceed to evaluate the HU intensity distribution specific to calcific regions (Figure 1B; Figure 5G-5I). With higher intensity corresponding to denser calcific concentrations, this may also correspond to earlier vs later calcific deposition (26). From one aspect, diffuse regions may be attributed to late-stage leaflet fibrosis (16). Another aspect of calcification intensity grading is the possibility of predicting leaflet mobility, given that the pattern and rate of calcific progression affects the tissue material properties inducing impairment to physiological function (23-26).
Volume scores for patients with AS in contrast-enhanced images are significantly lower than those using non-contrast images
Previously reported volume scores using contrast images (14,17-19) were considerably lower compared to expected volume scores in AS (21,30). This apparent underestimation, even when using different techniques, was not sufficiently discussed in the literature. Quantifying this bias has important implications for both the diagnostic and prognostic use cases of contrast CT imaging. Indeed, quantifying this relative error in measurement would decrease uncertainty in deriving sex-specific thresholds for AS severity determination. In terms of the comparing contrast and non-contrast calcific estimation, we can observe close qualitative agreement with detected calcification, both in terms of location relative to aortic leaflet surface and pattern of distribution (Figure 3). Additionally, we showed significant correlation in volume between both non-contrast and contrast images (Figure 6A). Despite these agreements, the total volume scores for contrast images seem to be significantly underestimated compared to those for non-contrast images (Figures 3,4B; Table 2). Potential reasons for this disagreement are as follows (31,32). The non-contrast Agatston protocol uses a lower axial resolution (3 vs. 0.625 mm), which may cause an overestimation of large calcific nodules and underestimation of smaller deposits. This is due to the increased slice thickness via the partial volume effect (33). This error would be nonlinear and sensitive to local calcium densities and orientation, which we can observe in our experiments (Figure 3B,3H; Figure 4B-4F). And this also can explain observed underestimation of calcium scores when using the “en-face” reconstructed view (34). Secondly, dense calcific regions with large HU values may produce blooming artifacts (32) which could further compound the volume error with a higher slice thickness. Both factors may imply a more accurate volume measurement using contrast images with lower slice thickness and contrast differentiation between the tissues.
Varied patterns of calcific distribution can be detected and quantified using contrast CT
In order to better assess calcification in various regions of the cardiovascular system, new quantitative criteria (13) were suggested to go beyond the existing calcification assessment methods (12) which only rely on the quantity of calcification. These markers could be especially important in the context of TAVR planning since different factors can influence the severity of various complications during and post-operation such as the location, quantity, and type of the calcific deposits (6,7,35,36). We have, therefore, developed a geometrical mapping framework that quantifies the topology of the calcification in the AV based on the common anatomical definitions of a tricuspid AV (37,38) and focused on the leaflet region of the valve to provide an in-vivo quantitative description of the pattern of calcific progression in AS.
We showed qualitative and quantitative patterns of calcific distribution that seem to closely match those demonstrated in ex-vivo studies by Thubrikar et al. (23), and those posited in biomechanical simulated models (24-26,38). These experiments chiefly entail the following: calcification advances primarily from the high stress regions of the valve leaflets which form arcs and eventually rings covering the leaflet’s surface. Among the four distinctive patterns described previously (24-26), we observed that the primary pattern of calcific arcs forming between the two attachment edges of each leaflet along the belly seems to dominate across sex and severity categories (Figure 5G-5J, Figure 6B). In severe tricuspid cases, calcific nodules form arcs connecting the root attachment points and eventually form fully connected rings that conform to the free and fixed edge of the leaflets from both sides (Figure 5). In contrast, non-severe AS cases have calcification that is less developed and concentrated near the coaptation between the non and left coronary leaflets (Figure 5). We also demonstrated that this pattern is presented in both males and females with females experiencing a significantly lower amount across all regions (Figures 5,6). Differences in AV size do not sufficiently explain this wide discrepancy (Table 2). This finding provides further evidence that biomechanics of functional impairment in females are not dominated by calcific leaflet thickening and it seems that leaflet fibrosis could play a much bigger role in worsening clinical symptoms for females with AS (16,27,28,39). In addition, we further show that asymmetry in cusp sizes and shapes (40) leads to analogous asymmetry in quantity and pattern of calcific distribution across the valve. Regarding regional volume scores, we show a relative increase in non-coronary cusp (NCC) calcification which agrees with previous experiments using similar patient cohorts and image modalities (7) (Table 2). The relatively longer length of the NCC (40) along with the direction of calcific progression can explain the discrepancy in volume. This observation could have important implications for post-procedural complications due to the proximity of NCC calcification to the ventricular conduction system (6,7,29). In summary, accurate quantification of the asymmetry in calcific deposits may be important to avoid various procedural complications if related to the positioning and expansion of the implant (7,10,18,41).
This study aims to shed further light on the relationship between calcific leaflet thickening, subsequent valve obstruction and presentation of clinical symptoms (42). A better understanding the biomechanical causes of impaired leaflet mobility may provide an opportunity to decide on optimal intervention times, new staging criteria (43) and personalized patient treatment (44,45).
Limitations
Preliminary findings from this study are based on an observational cohort of patients. Our non-consecutive cohort was chosen to evaluate and verify the proposed methods in a balanced distribution of sex and AS severity which may not reflect in a larger population study. Further investigation is needed in a multi-center setting to better judge the effectiveness of the method in more clinical contexts as well as interscan and inter-device variability. Finally, some of the proposed metrics for calcification assessment should be further analyzed in the context of peri-procedural outcomes in TAVR/TAVI. Future work is needed to develop stronger indices based on these descriptors, which can provide a prognostic impact in relation with implantable device size, type, and expansion behavior.
Conclusions
Calcific AS is the most common valvular disease, with high mortality rate once symptoms are presented. AVR intervention is currently the only treatment option in which AVC deposited in and around the valve is an important factor for procedural risk assessment and predicting complications in TAVR. The presented computational assessment tool can be used to characterize the complex patterns of calcification at different stages of the disease. We found that calcific progression appears to follow distinctive patterns for each leaflet, with differences emerging in the stage of calcific progression between the leaflets. This difference in rate of progression is also significantly affected by sex which cannot be explained by the difference in valve sizes. In addition, we provide a new method for calcific detection that could potentially overcome the inherent variability of contrast material effect on HU attenuation which could enable additional quantitative criteria for calcific assessment in routinely used contrast CT for TAVR planning. In summary, detailed quantitative description of the complex patterns of leaflet calcification, combined with accurate anatomical assessment of AV morphology, could be critical for simultaneously monitoring the progression of AS, and determining the best treatment options, especially for asymptomatic patients who might eventually need an AV replacement.
Acknowledgments
The authors would like to thank Dr. Tej Sheth (Hamilton Health Sciences and Niagara Health, McMaster University, Hamilton, ON, Canada) and their team for their valuable contributions to this work in terms of access to patient images, demographic information, and baseline characteristics.
Funding: This work was supported by
Footnote
Conflicts of Interest: All authors have completed the ICMJE uniform disclosure form (available at https://qims.amegroups.com/article/view/10.21037/qims-23-778/coif). The authors have no conflicts of interest to declare.
Ethical Statement: The authors are accountable for all aspects of the work in ensuring that questions related to the accuracy or integrity of any part of the work are appropriately investigated and resolved. The study was conducted in accordance with the Declaration of Helsinki (as revised in 2013). The study was approved by the Hamilton integrated research ethics board (HiREB) and individual consent for this retrospective analysis was waived.
Open Access Statement: This is an Open Access article distributed in accordance with the Creative Commons Attribution-NonCommercial-NoDerivs 4.0 International License (CC BY-NC-ND 4.0), which permits the non-commercial replication and distribution of the article with the strict proviso that no changes or edits are made and the original work is properly cited (including links to both the formal publication through the relevant DOI and the license). See: https://creativecommons.org/licenses/by-nc-nd/4.0/.
References
- Clavel MA, Pibarot P. A Decade of Revolutions in Calcific Aortic Stenosis. Cardiol Clin 2020;38:xiii-xiv. [Crossref] [PubMed]
- Lindman BR, Clavel MA, Mathieu P, Iung B, Lancellotti P, Otto CM, Pibarot P. Calcific aortic stenosis. Nat Rev Dis Primers 2016;2:16006. [Crossref] [PubMed]
- Généreux P, Stone GW, O'Gara PT, Marquis-Gravel G, Redfors B, Giustino G, Pibarot P, Bax JJ, Bonow RO, Leon MB. Natural History, Diagnostic Approaches, and Therapeutic Strategies for Patients With Asymptomatic Severe Aortic Stenosis. J Am Coll Cardiol 2016;67:2263-88. [Crossref] [PubMed]
- Siontis GCM, Overtchouk P, Cahill TJ, Modine T, Prendergast B, Praz F, Pilgrim T, Petrinic T, Nikolakopoulou A, Salanti G, Søndergaard L, Verma S, Jüni P, Windecker S. Transcatheter aortic valve implantation vs. surgical aortic valve replacement for treatment of symptomatic severe aortic stenosis: an updated meta-analysis. Eur Heart J 2019;40:3143-53. [Crossref] [PubMed]
- Pollari F, Hitzl W, Vogt F, Cuomo M, Schwab J, Söhn C, Kalisnik JM, Langhammer C, Bertsch T, Fischlein T, Pfeiffer S. Aortic valve calcification as a risk factor for major complications and reduced survival after transcatheter replacement. J Cardiovasc Comput Tomogr 2020;14:307-13. [Crossref] [PubMed]
- Blanke P, Weir-McCall JR, Achenbach S, Delgado V, Hausleiter J, Jilaihawi H, Marwan M, Norgaard BL, Piazza N, Schoenhagen P, Leipsic JA. Computed tomography imaging in the context of transcatheter aortic valve implantation (TAVI) / transcatheter aortic valve replacement (TAVR): An expert consensus document of the Society of Cardiovascular Computed Tomography. J Cardiovasc Comput Tomogr 2019;13:1-20. [Crossref] [PubMed]
- Khalique OK, Hahn RT, Gada H, Nazif TM, Vahl TP, George I, Kalesan B, Forster M, Williams MB, Leon MB, Einstein AJ, Pulerwitz TC, Pearson GD, Kodali SK. Quantity and location of aortic valve complex calcification predicts severity and location of paravalvular regurgitation and frequency of post-dilation after balloon-expandable transcatheter aortic valve replacement. JACC Cardiovasc Interv 2014;7:885-94. [Crossref] [PubMed]
- Généreux P, Head SJ, Hahn R, Daneault B, Kodali S, Williams MR, van Mieghem NM, Alu MC, Serruys PW, Kappetein AP, Leon MB. Paravalvular leak after transcatheter aortic valve replacement: the new Achilles' heel? A comprehensive review of the literature. J Am Coll Cardiol 2013;61:1125-36. [Crossref] [PubMed]
- Pollari F, Dell'Aquila AM, Söhn C, Marianowicz J, Wiehofsky P, Schwab J, Pauschinger M, Hitzl W, Fischlein T, Pfeiffer S. Risk factors for paravalvular leak after transcatheter aortic valve replacement. J Thorac Cardiovasc Surg 2019;157:1406-1415.e3. [Crossref] [PubMed]
- Mauri V, Deuschl F, Frohn T, Schofer N, Linder M, Kuhn E, Schaefer A, Rudolph V, Madershahian N, Conradi L, Rudolph TK, Schäfer U. Predictors of paravalvular regurgitation and permanent pacemaker implantation after TAVR with a next-generation self-expanding device. Clin Res Cardiol 2018;107:688-97. [Crossref] [PubMed]
- Pawade T, Sheth T, Guzzetti E, Dweck MR, Clavel MA. Why and How to Measure Aortic Valve Calcification in Patients With Aortic Stenosis. JACC Cardiovasc Imaging 2019;12:1835-48. [Crossref] [PubMed]
- Agatston AS, Janowitz WR, Hildner FJ, Zusmer NR, Viamonte M Jr, Detrano R. Quantification of coronary artery calcium using ultrafast computed tomography. J Am Coll Cardiol 1990;15:827-32. [Crossref] [PubMed]
- Blaha MJ, Mortensen MB, Kianoush S, Tota-Maharaj R, Cainzos-Achirica M. Coronary Artery Calcium Scoring: Is It Time for a Change in Methodology? JACC Cardiovasc Imaging 2017;10:923-37. [Crossref] [PubMed]
- Jilaihawi H, Makkar RR, Kashif M, Okuyama K, Chakravarty T, Shiota T, Friede G, Nakamura M, Doctor N, Rafique A, Shibayama K, Mihara H, Trento A, Cheng W, Friedman J, Berman D, Fontana GP. A revised methodology for aortic-valvar complex calcium quantification for transcatheter aortic valve implantation. Eur Heart J Cardiovasc Imaging 2014;15:1324-32. [Crossref] [PubMed]
- Alqahtani AM, Boczar KE, Kansal V, Chan K, Dwivedi G, Chow BJ. Quantifying Aortic Valve Calcification using Coronary Computed Tomography Angiography. J Cardiovasc Comput Tomogr 2017;11:99-104. [Crossref] [PubMed]
- Cartlidge TR, Bing R, Kwiecinski J, Guzzetti E, Pawade TA, Doris MK, Adamson PD, Massera D, Lembo M, Peeters FECM, Couture C, Berman DS, Dey D, Slomka P, Pibarot P, Newby DE, Clavel MA, Dweck MR. Contrast-enhanced computed tomography assessment of aortic stenosis. Heart 2021;107:1905-11. [Crossref] [PubMed]
- Eberhard M, Mastalerz M, Frauenfelder T, Tanner FC, Maisano F, Nietlispach F, Seifert B, Alkadhi H, Nguyen-Kim TDL. Quantification of aortic valve calcification on contrast-enhanced CT of patients prior to transcatheter aortic valve implantation. EuroIntervention 2017;13:921-7. [Crossref] [PubMed]
- Bettinger N, Khalique OK, Krepp JM, Hamid NB, Bae DJ, Pulerwitz TC, Liao M, Hahn RT, Vahl TP, Nazif TM, George I, Leon MB, Einstein AJ, Kodali SK. Practical determination of aortic valve calcium volume score on contrast-enhanced computed tomography prior to transcatheter aortic valve replacement and impact on paravalvular regurgitation: Elucidating optimal threshold cutoffs. J Cardiovasc Comput Tomogr 2017;11:302-8. [Crossref] [PubMed]
- Kim WK, Renker M, Rolf A, Liebetrau C, Van Linden A, Arsalan M, Doss M, Rieck J, Opolski MP, Möllmann H, Walther T, Hamm CW. Accuracy of device landing zone calcium volume measurement with contrast-enhanced multidetector computed tomography. Int J Cardiol 2018;263:171-6. [Crossref] [PubMed]
- Abdelkhalek M, Daeian M, Chavarria J, Sellers S, Gulsin G, Leipsic J, Sheth T, Keshavarz-Motamed Z. Patterns and Structure of Calcification in Aortic Stenosis: An Approach on Contrast-Enhanced CT Images. JACC Cardiovasc Imaging 2023;16:1224-6. [Crossref] [PubMed]
- Pawade T, Clavel MA, Tribouilloy C, Dreyfus J, Mathieu T, Tastet L, et al. Computed Tomography Aortic Valve Calcium Scoring in Patients With Aortic Stenosis. Circ Cardiovasc Imaging 2018;11:e007146. [Crossref] [PubMed]
- Baumgartner H, Hung J, Bermejo J, Chambers JB, Edvardsen T, Goldstein S, Lancellotti P, LeFevre M, Miller F Jr, Otto CM. Recommendations on the Echocardiographic Assessment of Aortic Valve Stenosis: A Focused Update from the European Association of Cardiovascular Imaging and the American Society of Echocardiography. J Am Soc Echocardiogr 2017;30:372-92. [Crossref] [PubMed]
- Thubrikar MJ, Aouad J, Nolan SP. Patterns of calcific deposits in operatively excised stenotic or purely regurgitant aortic valves and their relation to mechanical stress. Am J Cardiol 1986;58:304-8. [Crossref] [PubMed]
- Bäck M, Gasser TC, Michel JB, Caligiuri G. Biomechanical factors in the biology of aortic wall and aortic valve diseases. Cardiovasc Res 2013;99:232-41. [Crossref] [PubMed]
- Arzani A, Mofrad MRK. A strain-based finite element model for calcification progression in aortic valves. J Biomech 2017;65:216-20. [Crossref] [PubMed]
- Halevi R, Hamdan A, Marom G, Mega M, Raanani E, Haj-Ali R. Progressive aortic valve calcification: three-dimensional visualization and biomechanical analysis. J Biomech 2015;48:489-97. [Crossref] [PubMed]
- Myasoedova VA, Di Minno A, Songia P, Massaiu I, Alfieri V, Valerio V, Moschetta D, Andreini D, Alamanni F, Pepi M, Trabattoni D, Poggio P. Sex-specific differences in age-related aortic valve calcium load: A systematic review and meta-analysis. Ageing Res Rev 2020;61:101077. [Crossref] [PubMed]
- Thaden JJ, Nkomo VT, Suri RM, Maleszewski JJ, Soderberg DJ, Clavel MA, Pislaru SV, Malouf JF, Foley TA, Oh JK, Miller JD, Edwards WD, Enriquez-Sarano M. Sex-related differences in calcific aortic stenosis: correlating clinical and echocardiographic characteristics and computed tomography aortic valve calcium score to excised aortic valve weight. Eur Heart J 2016;37:693-9. [Crossref] [PubMed]
- Hansson NC, Nørgaard BL, Barbanti M, Nielsen NE, Yang TH, Tamburino C, et al. The impact of calcium volume and distribution in aortic root injury related to balloon-expandable transcatheter aortic valve replacement. J Cardiovasc Comput Tomogr 2015;9:382-92. [Crossref] [PubMed]
- Angelillis M, Costa G, De Backer O, Mochi V, Christou A, Giannini C, Spontoni P, De Carlo M, Søndergaard L, Miccoli M, Petronio AS. Threshold for calcium volume evaluation in patients with aortic valve stenosis: correlation with Agatston score. J Cardiovasc Med (Hagerstown) 2021;22:496-502. [Crossref] [PubMed]
- van der Bijl N, de Bruin PW, Geleijns J, Bax JJ, Schuijf JD, de Roos A, Kroft LJ. Assessment of coronary artery calcium by using volumetric 320-row multi-detector computed tomography: comparison of 0.5 mm with 3.0 mm slice reconstructions. Int J Cardiovasc Imaging 2010;26:473-82. [Crossref] [PubMed]
- Kalisz K, Buethe J, Saboo SS, Abbara S, Halliburton S, Rajiah P. Artifacts at Cardiac CT: Physics and Solutions. Radiographics 2016;36:2064-83. [Crossref] [PubMed]
- Šprem J, de Vos BD, Lessmann N, van Hamersvelt RW, Greuter MJW, de Jong PA, Leiner T, Viergever MA, Išgum I. Coronary calcium scoring with partial volume correction in anthropomorphic thorax phantom and screening chest CT images. PLoS One 2018;13:e0209318. [Crossref] [PubMed]
- Guzzetti E, Simard L, Clisson M, Clavel MA. Multiplanar “En Face” Reconstruction of the Aortic Valve: Impact on Aortic Valve Calcium Scoring. JACC Cardiovasc Imaging 2020;13:2678-80. [Crossref] [PubMed]
- Keshavarz-Motamed Z, Khodaei S, Rikhtegar Nezami F, Amrute JM, Lee SJ, Brown J, Ben-Assa E, Garcia Camarero T, Ruano Calvo J, Sellers S, Blanke P, Leipsic J, de la Torre Hernandez JM, Edelman ER. Mixed Valvular Disease Following Transcatheter Aortic Valve Replacement: Quantification and Systematic Differentiation Using Clinical Measurements and Image-Based Patient-Specific In Silico Modeling. J Am Heart Assoc 2020;9:e015063. [Crossref] [PubMed]
- Khodaei S, Sadeghi R, Blanke P, Leipsic J, Emadi A, Keshavarz-Motamed Z. Towards a non-invasive computational diagnostic framework for personalized cardiology of transcatheter aortic valve replacement in interactions with complex valvular, ventricular and vascular disease. International Journal of Mechanical Sciences 2021;202–203:106506.
- Piazza N, de Jaegere P, Schultz C, Becker AE, Serruys PW, Anderson RH. Anatomy of the aortic valvar complex and its implications for transcatheter implantation of the aortic valve. Circ Cardiovasc Interv 2008;1:74-81. [Crossref] [PubMed]
- Bahadormanesh N, Tomka B, Kadem M, Khodaei S, Keshavarz-Motamed Z. An ultrasound-exclusive non-invasive computational diagnostic framework for personalized cardiology of aortic valve stenosis. Med Image Anal 2023;87:102795. [Crossref] [PubMed]
- Pantelidis P, Oikonomou E, Lampsas S, Zakynthinos GE, Lysandrou A, Kalogeras K, Katsianos E, Theofilis P, Siasos G, Vavuranakis MA, Antonopoulos AS, Tousoulis D, Vavouranakis M. Lipoprotein(a) and calcific aortic valve disease initiation and progression: a systematic review and meta-analysis. Cardiovasc Res 2023;119:1641-55. [Crossref] [PubMed]
- Schäfers HJ, Schmied W, Marom G, Aicher D. Cusp height in aortic valves. J Thorac Cardiovasc Surg 2013;146:269-74. [Crossref] [PubMed]
- Khodaei S, Garber L, Abdelkhalek M, Maftoon N, Emadi A, Keshavarz-Motamed Z. Reducing Long-Term Mortality Post Transcatheter Aortic Valve Replacement Requires Systemic Differentiation of Patient-Specific Coronary Hemodynamics. J Am Heart Assoc 2023;12:e029310. [Crossref] [PubMed]
- Pawade TA, Newby DE, Dweck MR. Calcification in Aortic Stenosis: The Skeleton Key. J Am Coll Cardiol 2015;66:561-77. [Crossref] [PubMed]
- Généreux P, Pibarot P, Redfors B, Mack MJ, Makkar RR, Jaber WA, et al. Staging classification of aortic stenosis based on the extent of cardiac damage. Eur Heart J 2017;38:3351-8. [Crossref] [PubMed]
- Kadem M, Garber L, Abdelkhalek M, Al-Khazraji BK, Keshavarz-Motamed Z. Hemodynamic Modeling, Medical Imaging, and Machine Learning and Their Applications to Cardiovascular Interventions. IEEE Rev Biomed Eng 2023;16:403-23. [Crossref] [PubMed]
- Mohamed Abdelkhalek, Nikrouz Bahadormanesh, Javier Ganame, Zahra Keshavarz-Motamed, Incremental prognostic value of intensity-weighted regional calcification scoring using contrast CT imaging in TAVR, European Heart Journal - Imaging Methods and Practice, Volume 1, Issue 2, September 2023, qyad027