New evidence for fractional pressure ratio prediction by pulsatility index from transcranial Doppler in patients with symptomatic cerebrovascular stenosis disease
Introduction
Fractional flow reserve (FFR) serves as a pressure wire–derived index that quantifies the maximum blood flow to perfused tissue and is presented as a ratio between the pressures beyond the stenosis and the aortic pressure. FFR has been widely applied in a number of multicenter, randomized trials (1-3), guiding revascularization decisions within the cardiovascular field. Its endorsement within guidelines underscores its utility (4). Extending FFR’s utilization holds the potential to bring about substantial benefits for stroke survivors. In the field of cardiovascular research, assessing FFR requires inducing cerebral hyperemia in patients. However, due to the uncertain risks associated with inducing hyperemia in the cerebral arteries, this study refrained from using this method during the assessment. Instead, we opted to measure the pressure both distal and proximal to the stenotic lesion, leading us to introducing the term invasive fractional pressure ratio (FPR). However, the adoption of FPR alongside the pressure wire remains limited (5-7), which is likely attributable to technical intricacies, potential procedural risks linked to navigating the pressure wire within cerebral arteries, increased time and expenses, and the prospect of exacerbating reduced perfusion. Developing an approach capable of accurately representing the FPR within the context of cerebrovascular stenosis disease would yield notable advantages.
Transcranial Doppler (TCD) ultrasonography is a valuable and noninvasive screening technique with the capacity to swiftly identify hemodynamic alterations within a stenotic lesion (8-10). By providing further insights into distal downstream flow and reflecting localized shifts in blood flow at the site of a vascular lesion, TCD expands our comprehension of cerebral hemodynamics. The pulsatility index (PI), a frequently employed hemodynamic metric, serves to characterize the pulsatile nature of TCD waveforms (10). A variety of studies have been conducted that examine the clinical significance of the PI for appraising distal cerebrovascular resistance (CVR). These studies have collectively established that PI reliably signifies distal CVR, which is supported by an array of experimental and clinical studies (8,9,11-13). Moreover, the alterations in CVR are believed to serve as an indicator of cerebral perfusion status, a notion corroborated by other research (14,15).
Therefore, we intended to explore the relationship and determine the agreement between the PI derived from TCD and the FPR quantified through a pressure wire in patients with cerebrovascular stenosis disease. The fundamental aim of this investigation was to ascertain the feasibility of employing TCD PI as a potential indicator of the invasive FPR measurement.
Methods
Study design and population
This study enrolled patients with symptomatic atherosclerotic lesions and luminal stenosis ranging from 50% to 70% who underwent cerebrovascular angiography and FPR assessment at Beijing Tiantan Hospital. The demographic data, presenting symptoms at the time of admission, and vascular risk factors were retrospectively collected from the patients’ medical records. The study was approved by the ethics committee of Beijing Tiantan Hospital, which sanctioned the off-label use of the pressure guidewire, and was conducted in compliance with the principles outlined in the Declaration of Helsinki (as revised in 2013). The pressure guidewire was used as an auxiliary investigation tool in 2 clinical trials: Wingspan Stenting for Symptomatic Intracranial Artery Stenosis Registry in China (No. KY2013-013-02) and Apollo Stenting Balloon-Mounted Stent for Symptomatic Intracranial Artery Stenosis Registry in China (No. QX2012-012-02). Patients were selected from a database at Beijing Tiantan Hospital in Beijing, China, and informed consent was obtained from these patients or their legal guardians.
Angiographic evaluation, TCD examination, and invasive measurement of the FPR
Diagnostic digital subtraction angiography (DSA) was performed at the beginning of the procedure to confirm the stenotic degree based on North America Symptomatic Carotid Endarterectomy Trial (NASCET) criteria.
All patients underwent a series of carotid and vertebral artery (VA) ultrasound examinations with the Doppler-BOX (DWL Elektronische Systeme GmbH, Singen, Germany) in accordance with a standard insonation protocol prior to surgery. The TCD flow was measured distally in each lesion. For internal carotid artery (ICA) C6–C7 segment stenosis, the distal part included the middle cerebral artery (MCA) M1 segment. For MCA M1 segment stenosis, the distal part included the MCA distal to the stenotic lesion. For basilar artery (BA) stenosis, the distal part included both the left and right posterior cerebral arteries (PCAs). For VA stenosis, the distal part included the BA. A handheld 2-MHz transducer was used to obtain Doppler signals from the main stem of the MCA through a transtemporal window at a depth of 56 to 60 mm. Additionally, we obtained Doppler signals of the ICA C6–C7 at a depth of 60 to 70 mm. Signals from the BA were collected at a depth of 80 to 90 mm using a handheld 2-MHz probe, while signals from the PCAs were collected at a depth of 50 to 70 mm through a transtemporal window. The systolic, diastolic, and mean velocities were measured and recorded for each artery, and the PI was calculated as follows: (systolic velocity – diastolic velocity)/mean velocity.
The endovascular procedures were performed under monitored anesthesia, with a pressure transducer attached to a 6-Fr guiding catheter that was first calibrated. An 0.014-inch pressure wire (PrimeWire Prestige Plus, San Diego, CA, USA) was then advanced through the guiding catheter to the tip of the catheter. The pressure-sensing microwire was used to measure the mean values of the arterial pressure of the proximal and distal to the stenosis lesion. The FPR was then calculated as the mean distal value divided by the mean proximal value.
Statistical analysis
Categorical variables are expressed as counts and percentages, while continuous variables are reported as means with the corresponding standard deviation (SD). The Pearson correlation coefficient was employed to determine the degree of correlation between the PI measured with TCD and the FPR at the distal end per stenosis segment and per TCD location. Subsequently, ordinal least square (OLS) linear regression was used to establish the linear relationship between PI and FPR, and the fitted OLS lines for the distal TCD PI at all stenosis lesions were generated and superimposed onto the scatter plot. The average difference between the TCD PI and FPR was then computed and depicted in a Bland-Altman plot with the mean and +1.96 SD of the differences provided to evaluate the deviation of the mean from zero and to compare the 95% confidence interval (CI) with the clinically acceptable limit of agreement (LOA). We conducted the statistical analysis using SAS software version 9.4 (SAS Institute, Inc., Cary, NC, USA), and all reported P values were 2-tailed, with a significance threshold of P<0.05.
Data availability
All data are available upon a reasonable request to the corresponding author.
Results
Our analysis included a cohort of 33 patients, 75.8% of whom were male, with an average age of 58.1 years. The scope of the study encompassed individuals afflicted with stenotic lesions who underwent TCD assessment and pressure wire-based FPR measurements at distal sites. Among the entire patient pool, 20 exhibited anterior circulation lesions. Specifically, there were 8 patients with stenosis in the ICA C1 segment, 6 with lesions in the ICA C6–C7 segment, and another 6 with stenosis occurring in the MCA M1 segment. Additionally, 13 patients displayed posterior circulation lesions, consisting of 4 cases with stenosis in the VA segment and 9 with lesions in the BA segment. A comprehensive summary of the baseline characteristics of all patients with stenosis across these 5 segments is presented in Table 1. The average translesional FPR registered at 0.65, with values ranging between 0.34 and 0.88.
Table 1
Characteristic | N (%)/mean ± SD |
---|---|
Number of patients | 33 |
Location of stenosis | |
Anterior circulation | 20 (60.6) |
ICA C1 | 8 (24.2) |
ICA C6–7 | 6 (18.2) |
MCA M1 | 6 (18.2) |
Posterior circulation | 13 (39.4) |
BA | 9 (27.3) |
VA V4 | 4 (12.1) |
Age, years | 58.1±9.3 |
Male | 25 (75.8) |
Risk factor | |
Hypertension | 22 (66.7) |
Diabetes | 12 (36.4) |
Hyperlipidemia | 14 (42.4) |
Previous TIA/stroke | 8 (24.2) |
Treatment | |
Stenting | 18 (54.5) |
Angioplasty | 11 (33.3) |
Angiography | 4 (12.1) |
SD, standard deviation; ICA C1, internal carotid artery C1 segment; ICA C6–7, internal carotid artery C6–C7 segment; MCA M1, middle cerebral artery M1 segment; BA, basilar artery; VA V4, vertebral artery V4 segment; TIA, transient ischemic attack.
The agreement analysis encompassed 42 TCD data points sourced from various sites distal to the lesion. Generally, the PI values closely parallel with the FPR values across the majority of TCD locations. Table 2 demonstrates the Pearson correlation coefficients between TCD PI and FPR concerning both lesion segments and TCD locations. Across most TCD locations situated distal to the lesions, correlation coefficients were less than 0.90% and had significant P values (P<0.001), thus signifying very robust positive correlations. The only exceptions were found in the distal TCD for MCA segment lesions (r=0.897; P=0.015) and VA V4 segment (r=0.964; P=0.036), which demonstrated slightly lower correlation coefficients.
Table 2
Circulation | Stenosis location | TCD location | Pearson correlation | Linear regression of TCD PI on FPR | |||
---|---|---|---|---|---|---|---|
r | P value | Intercept (95% CI) | Slope (95% CI) | ||||
Anterior | ICA C1 | C6–7 | 0.944 | <0.001 | −0.102 (−0.371 to 0.167) | 1.075 (0.699 to 1.451) | |
ICA C6–7 | M1 | 0.986 | <0.001 | −0.071 (−0.240 to 0.098) | 1.102 (0.848 to 1.357) | ||
MCA M1 | M1 | 0.897 | 0.015 | −0.120 (−0.565 to 0.325) | 1.112 (0.352 to 1.872) | ||
Posterior | BA | LPCA | 0.932 | 0.001 | 0.063 (−0.201 to 0.328) | 0.906 (0.554 to 1.257) | |
RPCA | 0.944 | <0.001 | −0.088 (−0.337 to 0.161) | 1.083 (0.746 to 1.420) | |||
V4 | BA | 0.964 | 0.036 | −0.093 (−0.823 to 0.637) | 1.119 (0.176 to 2.061) | ||
Anterior | All | All | 0.948 | <0.001 | −0.092 (−0.212 to 0.027) | 1.084 (0.904 to 1.265) | |
Posterior | All | All | 0.942 | <0.001 | −0.065 (−0.202 to 0.072) | 1.067 (0.885 to 1.250) | |
All | All | All | 0.954 | <0.001 | −0.097 (−0.177 to −0.018) | 1.102 (0.990 to 1.214) |
TCD, transcranial Doppler; PI, pulsatility index; FPR, fractional pressure ratio; CI, confidence interval; ICA C1, internal carotid artery C1 segment; ICA C6–7, internal carotid artery C6–C7 segment; MCA M1, middle cerebral artery M1 segment; BA, basilar artery; LPCA, left posterior cerebral artery; RPCA, right posterior cerebral artery; V4, vertebral artery V4 segment.
Table 2 displays the outcomes derived from ordinary least squares (OLS) regression analysis conducted across lesion segments and TCD locations. This analysis revealed a consistent positive linear relationship for each individual TCD location. Figure 1 graphically presents an OLS regression fitted line characterized by a slope of 0.832 (95% CI: 0.743–0.920) in relation to the nearest distal TCD assessment. This indicates a significant statistical deviation from a slope of 1 and a resulting distinct proportional variance between TCD PI and FPR. Additionally, the estimated intercept was 0.142 (95% CI: 0.083–0.202), signifying a constant disparity between the 2 variables. Figure 2 shows the scatterplot for TCD assessments positioned distally to the lesion within both the anterior and posterior circulation lesions, revealing strikingly similar slopes and intercepts between the regression lines for anterior and posterior circulation. These regression lines were determined using the OLS regression approach.
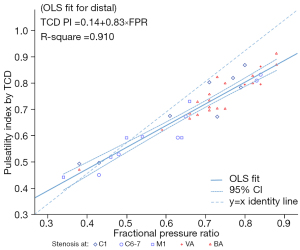
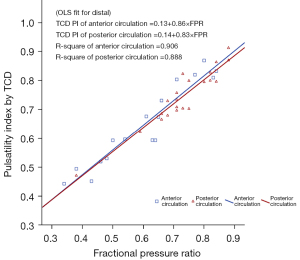
Figure 3 presents the Bland-Altman plots for the nearest distal TCD assessment. These plots reveal an insignificantly small disparity of 0.003 units between the 2 measures. The 95% confidence intervals (CIs) of the differences were confined within a range of 0.06 to 0.12, which aligns closely with the clinically acceptable LOA augmented by 0.01. The outcomes also indicate that the FPR values were slightly lower in comparison to the TCD PI values. Notably, this discrepancy was most prominent in cases with lower average values; specifically, the difference (PI – FPR) was more substantial when the FPR values were lower.
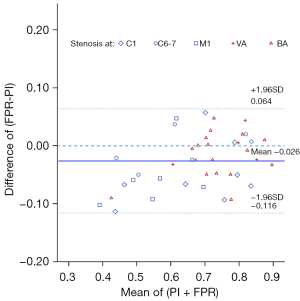
Discussion
The study’s most noteworthy discovery was that TCD PI distal to the stenosis showed a strong correlation and good agreement with FPR, as measured by a pressure wire. This result suggests that the PI obtained from noninvasive TCD at the nearest distal location might serve as a predictor of FPR in invasive pressure wire measurement and may also be used as a screening tool in making treatment decisions for cerebrovascular stenosis disease.
Several randomized trials conducted across multiple centers have employed pressure wire-based FFR to offer significant guidance for revascularization in patients diagnosed with coronary artery disease (CAD) (1-3). However, the invasive nature and technical intricacies of FFR-based pressure wire have hindered its widespread utilization in the treatment of cerebrovascular disease (6,16,17). Despite the pressing need for proper assessment tools that can provide logical and comprehensive parameters for cerebrovascular stenosis disease treatment decisions, such tools remain lacking. A parameter that incorporates multiple features of an arterial lesion by measuring its downstream flow effect, such as FPR, can potentially address this deficiency.
The TCD assessment tool stands out as the sole means of determining blood flow velocity and spectral waveform in real time (18,19). Currently, the most widely used hemodynamic index is PI, which has been studied by numerous researchers by virtue of its effectiveness in evaluating cerebral perfusion pressure (CPP) and distal CVR (8,11). There is a notable correlation between vascular resistance, intraluminal pressure, and FFR (20). Previously, the correlation among PI, CVR, and CPP have also been investigated (8,12,13,21,22). Despite these findings, the clinical implications of PI remain relatively unexplored, and its value in informing treatment decisions for cerebrovascular stenosis disease is underestimated due to inadequate scientific evidence.
As one of the parameters used for transit-time flow measurement, PI has been found to have a positive correlation with FFR in the field of cardiovascular medicine (23). Surprisingly, our investigation unveiled a robust association between TCD-derived PI and FPR within the context of cerebrovascular stenosis. Additionally, we observed a close concurrence between the PI downstream of the stenotic lesion and FPR. To our knowledge, our study represents a pioneering effort in identifying this connection in the domain of cerebrovascular disease. The notable efficacy of the PI in the distal position was within our expectations, given that distal the PI is anticipated to reflect the state of distal perfusion and vascular resistance. Nonetheless, further extensive research is imperative to ascertain the precise nature of this correlation.
The significant correlation observed between the FPR-based wire and PI from TCD assessments serves to highlight the advantages of using this approach. Despite the compensatory effects of collateral circulation, PI has the ability to accurately portray changes in intravascular hemodynamics within atherosclerotic stenotic lesions. As a result, it currently plays a pivotal role in predicting FPR values. The outcomes of the current study indicate that the TCD PI has the potential to provide a safer, more accessible, and potentially dependable means of conducting assessments guided by physiological factors, which closely correspond with the reference standard of pressure wire–based FPR. These findings hold considerable promise for facilitating treatment decisions based on physiological guidance in cases of cerebrovascular stenosis disease in the future. However, it is important to note that substantial prospective studies are needed to authenticate these findings.
A rationale for the correlation of PI and FPR based on 0-dimensional approximation
In general, the Navier-Stokes equations are considered suitable for modeling fluid mechanics systems. However, due to the intricate properties of blood and the challenges involved in establishing the vascular system, it is exceedingly difficult to calculate all blood parameters both before and after stenosis using this principle. As a solution to this issue, these complex electrodynamic equations are simplified into a relationship between flow and pressure, akin to the current and voltage in an electric system. This 0-dimensional hemodynamic approximation is employed to qualitatively describe the relationship between PI and FPR in this section.
As the direct current (DC) and alternating current (AC) in an electric system, the blood flow is comprised of steady and dynamic flow. According to Poiseuille’s law, the flow resistance of static components is primarily dependent on r−4, where r represents the diameter of the stenosis. Conversely, the behavior of dynamic components differs from that of static components. The resistance of dynamic flow is inversely proportional to the compliance of the blood vessels. Consequently, compliance (C) of a vessel can be defined as the changes in volume (V) over a given change in pressure (P) per unit of length, as follows:
Through the application of the stress formula and the assumption of a constant Young modulus throughout the stenosis, it is possible to ascertain that compliance is directly proportional to r. This indicates that the alteration in compliance during stenosis is notably smaller than is the change in steady flow resistance.
Hence, there is a distinct behavior between steady and dynamic flows in confined areas. The steady flow has a substantial decrease while the dynamic flow almost remains constant. Blood circulates through stenosis predominantly via dynamic flow. Consequently, the mathematical formulations can be represented as follows:
where a and b refer to distal and proximal vessel pressure, respectively, while s and d refer to static or dynamic pressure, respectively.
The PI value holds a significant physical significance, representing the ratio of the amplitude of the high-frequency component (dynamic flow) to the zero-frequency component (steady flow). When the dynamic flow undergoes minimal changes, the PI value for the distal end of a single stenosis is directly proportional to the pressure ratio between both ends.
In this context, the ultimate approximation suggests that in instances of mild to moderate stenosis, the compliance undergoes only a marginal alteration. Consequently, this leads to an approximate equilibrium in pressure between the distal and proximal vessel segments.
Limitations
It is important to note that this study has certain limitations that must be considered. First, the sample size was limited. Second, the TCD examination was operator-dependent, and the results were not cross-validated by an additional sonologist. Moreover, this study did not account for individual variations in the circle of Willis which may have an impact to the blood flow patterns. Third, although the present study showed good concordance between the TCD PI and the pressure wire FPR assessments, further research is necessary to standardize the parameter gauge. As per the earlier study (24), in asymptomatic carotid endarterectomy (CEA) patients, the PI is often reported to be ≥1.0, while in symptomatic CEA patients, it is typically <1.0. These values indicate reduced peripheral cerebral resistance and likely signify a degree of cerebral autoregulatory exhaustion. In symptomatic patients with a baseline PI <1.0 prior to CEA, there is often an observed increase in cerebral blood flow after plaque removal and flow restoration. Fourth, the correlation and agreement between TCD- and FPR-based pressure wires justify a large prospective study for verification purposes.
Conclusions
The TCD assessment-derived PI value shows promising agreement with the FPR value obtained from pressure wire measurements. This suggests that TCD PI could potentially serve as a dependable predictor for the actual FPR value in patients with cerebrovascular stenosis disease. However, it is essential to validate these findings through a large-scale prospective study to ensure their robustness and clinical applicability.
Acknowledgments
Funding: This work was supported by
Footnote
Conflicts of Interest: All authors have completed the ICMJE uniform disclosure form (available at https://qims.amegroups.com/article/view/10.21037/qims-23-701/coif). Xi Zhao is an employee of United Imaging Healthcare, Shanghai, China. The other authors have no conflicts of interest to declare.
Ethical Statement: The authors are accountable for all aspects of the work in ensuring that questions related to the accuracy or integrity of any part of the work are appropriately investigated and resolved. The study was approved by the ethics committee of Beijing Tiantan Hospital, which sanctioned the off-label use of the pressure guidewire, and was conducted in compliance with the principles outlined in the Declaration of Helsinki (as revised in 2013). The pressure guidewire was used as an auxiliary investigation tool in 2 clinical trials: Wingspan Stenting for Symptomatic Intracranial Artery Stenosis Registry in China (No. KY2013-013-02) and Apollo Stenting Balloon-Mounted Stent for Symptomatic Intracranial Artery Stenosis Registry in China (No. QX2012-012-02). Patients were selected from a database at Beijing Tiantan Hospital in Beijing, China, and informed consent was obtained from these patients or their legal guardians.
Open Access Statement: This is an Open Access article distributed in accordance with the Creative Commons Attribution-NonCommercial-NoDerivs 4.0 International License (CC BY-NC-ND 4.0), which permits the non-commercial replication and distribution of the article with the strict proviso that no changes or edits are made and the original work is properly cited (including links to both the formal publication through the relevant DOI and the license). See: https://creativecommons.org/licenses/by-nc-nd/4.0/.
References
- De Bruyne B, Pijls NH, Kalesan B, Barbato E, Tonino PA, Piroth Z, et al. Fractional flow reserve-guided PCI versus medical therapy in stable coronary disease. N Engl J Med 2012;367:991-1001. [Crossref] [PubMed]
- Pijls NH, van Schaardenburgh P, Manoharan G, Boersma E, Bech JW, van't Veer M, Bär F, Hoorntje J, Koolen J, Wijns W, de Bruyne B. Percutaneous coronary intervention of functionally nonsignificant stenosis: 5-year follow-up of the DEFER Study. J Am Coll Cardiol 2007;49:2105-11. [Crossref] [PubMed]
- Tonino PA, De Bruyne B, Pijls NH, Siebert U, Ikeno F. van' t Veer M, Klauss V, Manoharan G, Engstrøm T, Oldroyd KG, Ver Lee PN, MacCarthy PA, Fearon WF; . Fractional flow reserve versus angiography for guiding percutaneous coronary intervention. N Engl J Med 2009;360:213-24. [Crossref] [PubMed]
- Kolh P, Windecker S, Alfonso F, Collet JP, Cremer J, Falk V, et al. 2014 ESC/EACTS Guidelines on myocardial revascularization: the Task Force on Myocardial Revascularization of the European Society of Cardiology (ESC) and the European Association for Cardio-Thoracic Surgery (EACTS). Developed with the special contribution of the European Association of Percutaneous Cardiovascular Interventions (EAPCI). Eur J Cardiothorac Surg 2014;46:517-92. [Crossref] [PubMed]
- Han YF, Liu WH, Chen XL, Xiong YY, Yin Q, Xu GL, Zhu WS, Zhang RL, Ma MM, Li M, Dai QL, Sun W, Liu DZ, Duan LH, Liu XF. Severity assessment of intracranial large artery stenosis by pressure gradient measurements: A feasibility study. Catheter Cardiovasc Interv 2016;88:255-61. [Crossref] [PubMed]
- Miao Z, Liebeskind DS, Lo W, Liu L, Pu Y, Leng X, Song L, Xu X, Jia B, Gao F, Mo D, Sun X, Liu L, Ma N, Wang B, Wang Y, Wang Y. Fractional Flow Assessment for the Evaluation of Intracranial Atherosclerosis: A Feasibility Study. Interv Neurol 2016;5:65-75. [Crossref] [PubMed]
- Zanaty M, Rossen JD, Roa JA, Nakagawa D, Hudson JS, Kasab SA, Limaye K, Asi K, Dandapat S, Jabbour P, Samaniego EA, Hasan DM. Intracranial Atherosclerosis: A Disease of Functional, not Anatomic Stenosis? How Trans-Stenotic Pressure Gradients Can Help Guide Treatment. Oper Neurosurg (Hagerstown) 2020;18:599-605. [Crossref] [PubMed]
- de Riva N, Budohoski KP, Smielewski P, Kasprowicz M, Zweifel C, Steiner LA, Reinhard M, Fábregas N, Pickard JD, Czosnyka M. Transcranial Doppler pulsatility index: what it is and what it isn't. Neurocrit Care 2012;17:58-66. [Crossref] [PubMed]
- Lee KY, Sohn YH, Baik JS, Kim GW, Kim JS. Arterial pulsatility as an index of cerebral microangiopathy in diabetes. Stroke 2000;31:1111-5. [Crossref] [PubMed]
- Gosling RG, King DH. Arterial assessment by Doppler-shift ultrasound. Proc R Soc Med 1974;67:447-9.
- Bellner J, Romner B, Reinstrup P, Kristiansson KA, Ryding E, Brandt L. Transcranial Doppler sonography pulsatility index (PI) reflects intracranial pressure (ICP). Surg Neurol 2004;62:45-51; discussion 51. [Crossref] [PubMed]
- Giller CA, Hodges K, Batjer HH. Transcranial Doppler pulsatility in vasodilation and stenosis. J Neurosurg 1990;72:901-6. [Crossref] [PubMed]
- Lim MH, Cho YI, Jeong SK. Homocysteine and pulsatility index of cerebral arteries. Stroke 2009;40:3216-20. [Crossref] [PubMed]
- Nation DA, Wierenga CE, Clark LR, Dev SI, Stricker NH, Jak AJ, Salmon DP, Delano-Wood L, Bangen KJ, Rissman RA, Liu TT, Bondi MW. Cortical and subcortical cerebrovascular resistance index in mild cognitive impairment and Alzheimer's disease. J Alzheimers Dis 2013;36:689-98. [Crossref] [PubMed]
- Yew B, Nation DA. Cerebrovascular resistance: effects on cognitive decline, cortical atrophy, and progression to dementia. Brain 2017;140:1987-2001. [Crossref] [PubMed]
- Leng X, Lan L, Ip VHL, Liu H, Abrigo J, Liebeskind DS, Wong LKS, Leung TW. Noninvasive fractional flow in intracranial atherosclerotic stenosis: Reproducibility, limitations, and perspectives. J Neurol Sci 2017;381:150-2. [Crossref] [PubMed]
- Liu J, Yan Z, Pu Y, Shiu WS, Wu J, Chen R, Leng X, Qin H, Liu X, Jia B, Song L, Wang Y, Miao Z, Wang Y, Liu L, Cai XC. Functional assessment of cerebral artery stenosis: A pilot study based on computational fluid dynamics. J Cereb Blood Flow Metab 2017;37:2567-76. [Crossref] [PubMed]
- Feldmann E, Wilterdink JL, Kosinski A, Lynn M, Chimowitz MI, Sarafin J, Smith HH, Nichols F, Rogg J, Cloft HJ, Wechsler L, Saver J, Levine SR, Tegeler C, Adams R, Sloan M. The Stroke Outcomes and Neuroimaging of Intracranial Atherosclerosis (SONIA) trial. Neurology 2007;68:2099-106. [Crossref] [PubMed]
- Stroke outcome and neuroimaging of intracranial atherosclerosis (SONIA): design of a prospective, multicenter trial of diagnostic tests. Neuroepidemiology 2004;23:23-32. [Crossref] [PubMed]
- Siebes M, Chamuleau SA, Meuwissen M, Piek JJ, Spaan JA. Influence of hemodynamic conditions on fractional flow reserve: parametric analysis of underlying model. Am J Physiol Heart Circ Physiol 2002;283:H1462-70. [Crossref] [PubMed]
- Czosnyka M, Richards HK, Whitehouse HE, Pickard JD. Relationship between transcranial Doppler-determined pulsatility index and cerebrovascular resistance: an experimental study. J Neurosurg 1996;84:79-84. [Crossref] [PubMed]
- Hsu HY, Chern CM, Kuo JS, Kuo TB, Chen YT, Hu HH. Correlations among critical closing pressure, pulsatility index and cerebrovascular resistance. Ultrasound Med Biol 2004;30:1329-35. [Crossref] [PubMed]
- Honda K, Okamura Y, Nishimura Y, Uchita S, Yuzaki M, Kaneko M, Yamamoto N, Kubo T, Akasaka T. Graft flow assessment using a transit time flow meter in fractional flow reserve-guided coronary artery bypass surgery. J Thorac Cardiovasc Surg 2015;149:1622-8. [Crossref] [PubMed]
- Heyer EJ, Mergeche JL, Connolly ES Jr. Middle cerebral artery pulsatility index and cognitive improvement after carotid endarterectomy for symptomatic stenosis. J Neurosurg 2014;120:126-31. [Crossref] [PubMed]