Three-dimensional magnetic resonance neurography aids in detection of brachial plexus nerve root signal and size alterations in patients with amyotrophic lateral sclerosis: a case-control study
Introduction
Amyotrophic lateral sclerosis (ALS) is a fatal neurodegenerative disorder characterized by upper and lower motor neuron loss of the human nervous system, leading to progressive weakness of the bulbar, limb, thoracic, and abdominal muscles (1). Progressive motor neuron degeneration is the most predominant neurophysiologic change of ALS (2). Growing evidence indicates that the peripheral nervous system is affected in ALS pathogenetic cascade, especially at an early stage, including axonal degeneration, neuroinflammation, and Wallerian degeneration of fibers (3). Increasing evidence indicates that ALS is a distal axonopathy, with a distal to proximal gradient of damage in peripheral nervous system (4).
Detecting imaging abnormality of peripheral nerves may help to assess pathophysiological changes of ALS and to eventually improve diagnosis in ALS. Magnetic resonance neurography (MRN) has been widely used in the assessment of peripheral neuropathy (5-7), providing structural information to complement the functional information of neurophysiologic tests. There is limited literature on using MRN for ALS with heterogeneous results (8,9). Although Staff et al. qualitatively showed ALS lesions of the brachial plexus, which appeared similar to multifocal motor neuropathy lesions, no quantitative measurements were obtained in their work (10). They also did not use 3-dimensional (3D) MRN and it is common for the peripheral nerves and unsuppressed adjoining veins to exhibit isointense signal alterations on 2-dimensional (2D) short tau inversion recovery and T2-weighted images (T2WI) limiting the assessment of abnormal signal alterations of the obliquely coursing nerves of the plexi.
3D nerve-sheath signal increased with inked rest-tissue rapid acquisition of relaxation imaging (3D SHINKEI) is an MRN sequence that includes a combination of two preparation pulses and 3D turbo spin-echo sequence with T2-weighting. It enables vascular signal suppression and superior 3D visualization of detailed anatomy of the brachial plexus in high spatial resolution (11,12). However, patients with ALS have not been assessed quantitatively on imaging or using 3D SHINKEI.
Since previous histopathological studies have shown a distal to proximal gradient of axonal damage in peripheral nerves of patients with ALS (4), it would be worthwhile to evaluate consequence of such changes on magnetic resonance imaging (MRI). The aim of this study was to assess the proximal-distal longitudinal signal and size alterations of the brachial plexus roots using nerve-selective sequence, 3D SHINKEI. We present this article in accordance with the MDAR reporting checklist (available at https://qims.amegroups.com/article/view/10.21037/qims-23-833/rc).
Methods
Study participants
The study was conducted in accordance with the Declaration of Helsinki (as revised in 2013). The study was approved by the institutional ethics committee of Shandong Provincial Hospital and informed consent was provided by all individual participants. A total of 22 patients with sporadic ALS and 19 healthy controls (HCs) were recruited consecutively from December 2017 to July 2019 at Shandong Provincial Hospital. All participants with ALS had received a neurologic and electrophysiological examination by a neurologist specializing in peripheral nerve disorders with 23 years of experience in neurology. The inclusion criterion was a diagnosis of ALS in adult patients of all genders [clinically definite or probable by El Escorial criteria (13)]. All patients with ALS had clinical signs of lower motor neuron involvement (i.e., weakness, atrophy and/or fasciculations) in the forearm or hand. HC cases with no history of neurologic, psychiatric, or other major medical illnesses were recruited from friendship groups of patients with ALS and by word of mouth and were matched with regard to age.
MRN protocol
The scans were acquired on a 3 Tesla MR system (Ingenia, Philips Healthcare, Best, Netherlands) with a 16-channel neurovascular coil. Bilateral imaging of the brachial plexus was performed using 3D SHINKEI. The remaining protocol was used to exclude other diseases, including coronal T1-weighted spin-echo images [time to repeat (TR) 564 ms; time to echo (TE), 7.3 ms; flip angle, 90°; number of signal averages (NSA), 2; slice thickness, 3 mm; gap, 0.3 mm], fat-suppressed coronal short tau inversion recovery images [TR, 2,826 ms; TE, 90 ms; inversion time (TI), 160 ms; flip angle, 90°; NSA,1; slice thickness, 3 mm; gap, 0.3 mm]. 3D SHINKEI was performed with the following parameters: TR, 2,200 ms; TE, 90 ms; TI, 250 ms, field of view (FOV) = 280 mm × 400 mm, echo-train length (ETL) =100, matrix =232×321, the reconstructed voxel size =0.45×0.45×1.0 mm3, improved motion-sensitized driven equilibrium (iMSDE) duration =35 ms, phase encoding direction; right to left, percentage of phase oversampling =50%, acquisition time =6 min 06 s.
Data processing
Nerve root assessment of ALS patients and HCs was performed on 3D SHINKEI by two experienced neuroradiologists (SW and GW with 20 and 5 years of experience in neuroradiology, respectively) who were blinded to the diagnosis and electrophysiological examination findings. The nerve root segment was defined from a level immediately inferior to the spinal ganglia to the level of junction of brachial plexus root and trunk. The lengths of C5–C8 nerve root segments were standardized respectively using the mean length of all participants’ roots as a standard. On this basis, the slope values of diameter and signal-to-noise (SNR) ratio value in C5–C8 roots were measured respectively. For each of the C5–C8 nerve roots, curved surface reconstruction of the whole root was performed and then divided into 5 equal segments. Regions of interest (ROIs) were placed in the center of each segment on the axial reformatted images that were perpendicular to the course of each root (in turn, named 1 through 5 from proximal to distal points), as illustrated in Figure 1. The diameter was calculated by the mean of longest diameter and shortest diameter at each point. The SNR was calculated as follows: SNR = SI (root)/SD (noise), where SI (root) is the signal intensity of the roots of brachial plexus, and SD (noise) is the standard deviation of the background noise measured in an ROI drawn outside of the image.
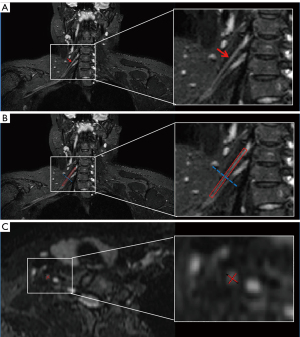
Statistical analyses
Statistical analysis was performed using the software GraphPad Prism Version 8 (GraphPad Software, San Diego, CA, USA). Chi-square test was used to determine differences in gender between ALS patients and HCs. Student’s t-test was used to determine differences in age, height, and weight between ALS patients and HCs and differences between left and right sides. The inter-observer agreement of the diameters and SNR values was assessed using intraclass correlation coefficient (ICC) analysis. The ICC ranges of reliability values provided by Landis and Koch were used as the reference standard (14).
The average data from both observers was used to calculate the case-control differences. Student’s t-test was performed to compare the differences at each point of C5–C8 nerve roots between ALS patients and HCs. Linear regression was performed for the diameters and SNR values of each root, and the differences in linear regression slopes between the two groups were analyzed by chi-square test. Receiver operating characteristic (ROC) curve analysis was obtained to evaluate the diagnostic performance of the diameter ratio and the SNR value ratio of the distal point (point 5) to the proximal point (point 1) in C5–C8 roots. The area under the ROC curve (AUC), cut-off values, sensitivity, and specificity were calculated. Statistical significance was denoted by P<0.05.
Results
Finally, 19 HCs and 21 patients with ALS were included as one patient was excluded due to the unclear nerve roots from chronic diffuse atrophy on 3D SHINKEI limiting the nerve root assessment. There were no significant differences in age, sex, weight, or height between the two groups. Table 1 shows the demographics of the ALS patients and HCs.
Table 1
Demographic or clinical feature | ALS patients | HCs | P value |
---|---|---|---|
No. of cases | 21 | 19 | |
Sex | 0.67 | ||
Female | 8 | 9 | |
Male | 13 | 10 | |
Age at MRI (years) | 61.68±7.17 | 62.16±8.42 | 0.85 |
Body weight (kg) | 72.41±12.12 | 67.63±11.88 | 0.43 |
Body height (cm) | 168.1±7.83 | 166.1±8.35 | 0.21 |
Duration of symptom onset before MRI (month) | 20.19±20.04 | – | – |
The values are presented as number or mean ± SD. ALS, amyotrophic lateral sclerosis; HC, healthy control; MRI, magnetic resonance imaging; SD, standard deviation.
ICCs for inter-observer agreement of diameter measurements were 0.895 [95% confidence interval (CI): 0.824–0.936] for C5 root, 0.852 (95% CI: 0.719–0.910) for C6 root, 0.912 (95% CI: 0.801–0.958) for C7 root, and 0.913 (95% CI: 0.822–0.973) for C8 root, respectively. ICCs for inter-observer agreement of SNR value were 0.821 (95% CI: 0.787–0.941) for C5 root, 0.840 (95% CI: 0.713–0.910) for C6 root, 0.802 (95% CI: 0.709–0.848) for C7 root, and 0.814 (95% CI: 0.742–0.853) for C8 root. No differences were found between the right and left nerve root values (P>0.05).
The 3D SHINKEI coronal images of brachial plexus of HCs and ALS patients are shown in Figure 2. The statistical results are presented in Figure 3, Figure 4, and Table 2. For each point, the diameters at point 1 and point 2 of C6–C8 roots were increased in patients with ALS compared with HCs and the diameters at point 4 and point 5 of C7 roots were decreased in patients with ALS compared with HCs. The SNR values at point 5 of C6–C8 roots and point 4 of C7 root were decreased in patients with ALS compared with HCs.
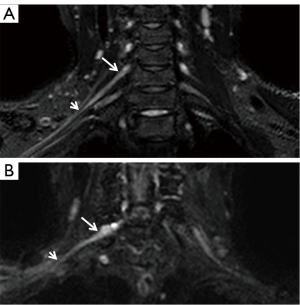
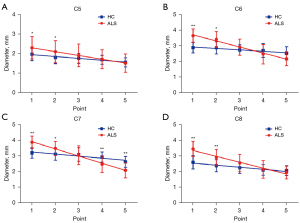
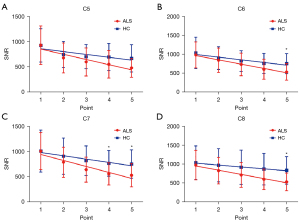
Table 2
Parameter | ALS patients | HCs | P | |||||
---|---|---|---|---|---|---|---|---|
Slope value | P value | Equation | Slope value | P value | Equation | |||
C5 diameter | −0.01924 | <0.001* | Y = −0.01924X + 2.500 | −0.009422 | 0.003* | Y = −0.009422X + 2.049 | <0.001* | |
C5 SNR value | −10.14 | 0.02* | Y = −10.14X + 958.2 | −5.552 | 0.06 | Y = −5.552X + 918.5 | 0.17 | |
C6 diameter | −0.04404 | <0.001* | Y = −0.04404X + 4.100 | −0.01039 | 0.01* | Y = −0.01039X + 3.023 | <0.001* | |
C6 SNR value | −12.86 | <0.001* | Y = −12.86X + 1081 | −8.009 | 0.01* | Y = −8.009X + 1068 | 0.03 | |
C7 diameter | −0.06228 | <0.001* | Y = −0.06228X + 4.374 | −0.01799 | 0.03* | Y = −0.01799X + 3.382 | <0.001* | |
C7 SNR value | −15.99 | 0.01* | Y = −15.99X + 1059 | −8.900 | 0.008* | Y = −8.900X + 1046 | 0.07 | |
C8 diameter | −0.06464 | 0.001* | Y = −0.06464X + 3.738 | −0.02294 | 0.004* | Y = −0.02294X + 2.684 | <0.001* | |
C8 SNR value | −19.06 | <0.001* | Y = −19.06X + 1060 | −8.631 | <0.001* | Y = −8.631X + 1074 | <0.001* |
*, P<0.05 was considered statistically significant. ALS, amyotrophic lateral sclerosis; HC, healthy control; SNR, signal-to-noise ratio.
Nerve diameters and SNR values tended towards a statistically significant decrease from proximal to distal except for the SNR value of C5 in HCs. The nerve diameters for ALS patients were more negatively sloped than HCs for all nerve roots (P<0.001 for C5, C6, C7, C8). The nerve SNR values for patients with ALS were more negatively sloped than those for HCs in C6 and C8 roots (P=0.03 for C6, P<0.001 for C8) yet there were no significant statistical differences in C5 and C7 roots. The variation tendency in each gender is presented in Figure S1, Figure S2, and Table S1.
The ROC curves for the diameter and SNR value ratio (cutoff values, sensitivity, specificity, and the AUC) are shown in Figure 5 and Table 3. The AUCs of the C6, C7, and C8 diameter ratios were higher than that of the C5 diameter ratio.
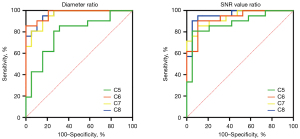
Table 3
Parameter | Sensitivity | Specificity | AUC | Cutoff value |
---|---|---|---|---|
C5 diameter | 80.95% | 73.68% | 0.7895 | 0.7418 |
C6 diameter | 85.71% | 100.00% | 0.9749 | 0.6952 |
C7 diameter | 80.95% | 94.74% | 0.9549 | 0.6431 |
C8 diameter | 90.48% | 89.47% | 0.9674 | 0.7147 |
C5 SNR value | 80.95% | 94.74% | 0.8822 | 0.5989 |
C6 SNR value | 90.48% | 89.47% | 0.9298 | 0.6516 |
C7 SNR value | 85.71% | 89.47% | 0.9373 | 0.6065 |
C8 SNR value | 90.48% | 94.74% | 0.9574 | 0.6758 |
ROC, receiver operating characteristic; SNR, signal-to-noise; AUC, area under the ROC curve.
Discussion
In this study, the results of 3D MRN imaging reveal that the diameters and SNR values of C5–C8 roots from proximal to distal decreased significantly and in ALS more than HCs. In addition, larger-size diameter changes of the proximal points were observed in C6–C8 roots of the ALS patients. In addition, the distal points demonstrated smaller diameters of C7 roots and smaller SNR values of C5–C8 roots. These results indicated that root alterations were different between proximal and distal points, as suggested in pathophysiological change. The ROC results further revealed the diameter and SNR value ratio of distal point to proximal point could distinguish ALS from HCs with high sensitivity and specificity.
In this study, the proximal nerve enlargement is consistent with the previous report of increased volume in the brachial plexus of ALS patients by Gerevini et al. (15). Potential etiologies for these findings could include neuroinflammation, endothelial dysfunction, and nerve edema (16) occurring during the course of ALS. Neuroinflammation is a primary pathologic feature in the ALS pathogenic cascade, characterized by activation and infiltration of monocytes/macrophages surrounding the degenerating peripheral nerve fibers, which occurs even before the onset of clinical signs of motor weakness (17-19). The proximal nerve enlargement might be related to this neuroinflammation and consequent nerve edema and swelling. Interestingly, the results further indicated that the diameters and SNR values of nerve roots at the distal points were decreased compared to controls, which could be a result of reduced myelinated fibers and chronic axonal degeneration (3). Given that ALS is a motor neuronopathy, progressive motor axonal degeneration and nerve fiber loss is considered a major cause of signs and symptoms in ALS (20,21). Furthermore, the changes of nerve diameters and SNR values from proximal to distal are consistent with the pathophysiological changes of ALS in peripheral nerves, indicating that ALS is a distal axonopathy, a pattern typically seen in peripheral neuropathies with a distal to proximal gradient of damage (4).
The differences from proximal to distal may be related to different disease stages. In this study, one ALS patient imaged 72.3 months after the onset of symptoms demonstrated entire brachial plexus atrophy including the proximal roots, reflecting chronicity of disease process and the late stage of the ALS pathogenetic cascade. This patient was excluded from our study as the markedly atrophic nerve roots were unclear on 3D MRN. Further prospective study with a larger number of patients is needed to ascertain whether this change observed in 3D MRN images is systematically associated with disease course and severity.
There has been contradiction in the literature regarding the diameters of nerve roots of patients with ALS. Most ultrasound studies have suggested that the roots are thinner in patients with ALS (22), whereas increased diameters have been found on the MRI studies (10). This study systematically evaluated the nerve roots at different segments from proximal to distal parts, and found that the signal and size alterations were different, which may account for the controversies above. 3D SHINKEI allowed excellent nerve selective imaging and depiction of the above-described lesions. This also contributed to excellent inter-reader reliability in both signal and size measurements despite the small size of the nerves.
To capture the decrease in nerve root diameters and SNR values from proximal to distal and to facilitate clinical application, the diameter ratio and SNR value ratio of the distal point (point 5) to the proximal point (point 1) in C5–C8 roots was chosen to evaluate the diagnostic performance. The high sensitivity and specificity of the ratio indicated that it may be helpful to evaluate the peripheral neuropathy of ALS. Moreover, the AUCs of C6–C8 diameters were higher than that of C5 diameter, which probably showed that C6–C8 diameters were more sensitive to the assessment of lesions of the peripheral nerves.
This study has some limitations. First, our patient population was small, which was attributed to the rarity of ALS. However, we measured five regions in eight nerve roots (bilateral C5–C8 roots), and the changes from proximal to distal were similar. Second, the diameters of nerve roots were evaluated, not the volumes. However, the diameter was calculated by the average of the longest diameter and shortest diameter at each point, which could produce little effect on the conclusion. In the future, nerve segmentation may help to measure the exact aggregate volumes of the nerves. Third, our study did not account for disease treatment; as there are no detailed studies of disease course and severity, further investigation may be necessary. Fourth, we did not evaluate the entirety of the brachial plexus. Due to its complex form, it is extremely difficult to measure all segments of the brachial plexus. Finally, we did not perform diffusion-weighted or diffusion tensor imaging (DTI). DTI can provide insight into the neuronal architecture. In the future, the DTI evaluation of brachial plexus in patients with ALS using DTI could be explored.
Conclusions
In conclusion, 3D MRN images reveal the proximal-distal longitudinal diameters and SNR values of brachial plexus nerve roots decreased and in ALS more than HCs. These results reflect pathophysiological changes of ALS and may be helpful in improving the diagnosis of ALS. Further prospective study is needed to ascertain whether this change is associated with disease course and severity.
Acknowledgments
Funding: This work was supported by
Footnote
Reporting Checklist: The authors have completed the MDAR reporting checklist. Available at https://qims.amegroups.com/article/view/10.21037/qims-23-833/rc
Conflicts of Interest: All authors have completed the ICMJE uniform disclosure form (available at https://qims.amegroups.com/article/view/10.21037/qims-23-833/coif). WC is an employee of Philips Healthcare in Shanghai. The other authors have no conflicts of interest to declare.
Ethical Statement: The authors are accountable for all aspects of the work in ensuring that questions related to the accuracy or integrity of any part of the work are appropriately investigated and resolved. The study was conducted in accordance with the Declaration of Helsinki (as revised in 2013). The study was approved by the institutional ethics committee of Shandong Provincial Hospital and informed consent was provided by all individual participants.
Open Access Statement: This is an Open Access article distributed in accordance with the Creative Commons Attribution-NonCommercial-NoDerivs 4.0 International License (CC BY-NC-ND 4.0), which permits the non-commercial replication and distribution of the article with the strict proviso that no changes or edits are made and the original work is properly cited (including links to both the formal publication through the relevant DOI and the license). See: https://creativecommons.org/licenses/by-nc-nd/4.0/.
References
- Turner MR, Swash M. The expanding syndrome of amyotrophic lateral sclerosis: a clinical and molecular odyssey. J Neurol Neurosurg Psychiatry 2015;86:667-73. [Crossref] [PubMed]
- Saberi S, Stauffer JE, Schulte DJ, Ravits J. Neuropathology of Amyotrophic Lateral Sclerosis and Its Variants. Neurol Clin 2015;33:855-76. [Crossref] [PubMed]
- Sobue G, Matsuoka Y, Mukai E, Takayanagi T, Sobue I. Pathology of myelinated fibers in cervical and lumbar ventral spinal roots in amyotrophic lateral sclerosis. J Neurol Sci 1981;50:413-21. [Crossref] [PubMed]
- Fischer LR, Culver DG, Tennant P, Davis AA, Wang M, Castellano-Sanchez A, Khan J, Polak MA, Glass JD. Amyotrophic lateral sclerosis is a distal axonopathy: evidence in mice and man. Exp Neurol 2004;185:232-40. [Crossref] [PubMed]
- Kronlage M, Bäumer P, Pitarokoili K, Schwarz D, Schwehr V, Godel T, Heiland S, Gold R, Bendszus M, Yoon MS. Large coverage MR neurography in CIDP: diagnostic accuracy and electrophysiological correlation. J Neurol 2017;264:1434-43. [Crossref] [PubMed]
- Fortanier E, Ogier AC, Delmont E, Lefebvre MN, Viout P, Guye M, Bendahan D, Attarian S. Quantitative assessment of sciatic nerve changes in Charcot-Marie-Tooth type 1A patients using magnetic resonance neurography. Eur J Neurol 2020;27:1382-9. [Crossref] [PubMed]
- Wu F, Wang W, Yang Y, Li C, Wu J, Liu H, Ren Y. MR neurography of lumbosacral nerve roots for differentiating chronic inflammatory demyelinating polyneuropathy from acquired axonal polyneuropathies: a cross-sectional study. Quant Imaging Med Surg 2022;12:4875-84. [Crossref] [PubMed]
- Kronlage M, Knop KC, Schwarz D, Godel T, Heiland S, Bendszus M, Bäumer P. Amyotrophic Lateral Sclerosis versus Multifocal Motor Neuropathy: Utility of MR Neurography. Radiology 2019;292:149-56. [Crossref] [PubMed]
- Rajabally YA, Jacob S. Chronic inflammatory demyelinating polyneuropathy-like disorder associated with amyotrophic lateral sclerosis. Muscle Nerve 2008;38:855-60. [Crossref] [PubMed]
- Staff NP, Amrami KK, Howe BM. Magnetic resonance imaging abnormalities of peripheral nerve and muscle are common in amyotrophic lateral sclerosis and share features with multifocal motor neuropathy. Muscle Nerve 2015;52:137-9. [Crossref] [PubMed]
- Kasper JM, Wadhwa V, Scott KM, Rozen S, Xi Y, Chhabra A. SHINKEI--a novel 3D isotropic MR neurography technique: technical advantages over 3DIRTSE-based imaging. Eur Radiol 2015;25:1672-7. [Crossref] [PubMed]
- Yoneyama M, Takahara T, Kwee TC, Nakamura M, Tabuchi T. Rapid high resolution MR neurography with a diffusion-weighted pre-pulse. Magn Reson Med Sci 2013;12:111-9. [Crossref] [PubMed]
- Brooks BR, Miller RG, Swash M, Munsat TLWorld Federation of Neurology Research Group on Motor Neuron Diseases. El Escorial revisited: revised criteria for the diagnosis of amyotrophic lateral sclerosis. Amyotroph Lateral Scler Other Motor Neuron Disord 2000;1:293-9. [Crossref] [PubMed]
- Kundel HL, Polansky M. Measurement of observer agreement. Radiology 2003;228:303-8. [Crossref] [PubMed]
- Gerevini S, Agosta F, Riva N, Spinelli EG, Pagani E, Caliendo G, Chaabane L, Copetti M, Quattrini A, Comi G, Falini A, Filippi M. MR Imaging of Brachial Plexus and Limb-Girdle Muscles in Patients with Amyotrophic Lateral Sclerosis. Radiology 2016;279:553-61. [Crossref] [PubMed]
- Stoll G, Müller HW. Nerve injury, axonal degeneration and neural regeneration: basic insights. Brain Pathol 1999;9:313-25. [Crossref] [PubMed]
- Evans MC, Couch Y, Sibson N, Turner MR. Inflammation and neurovascular changes in amyotrophic lateral sclerosis. Mol Cell Neurosci 2013;53:34-41. [Crossref] [PubMed]
- Philips T, Robberecht W. Neuroinflammation in amyotrophic lateral sclerosis: role of glial activation in motor neuron disease. Lancet Neurol 2011;10:253-63. [Crossref] [PubMed]
- Schreiber S, Schreiber F, Garz C, Debska-Vielhaber G, Assmann A, Perosa V, Petri S, Dengler R, Nestor P, Vielhaber S. Toward in vivo determination of peripheral nervous system immune activity in amyotrophic lateral sclerosis. Muscle Nerve 2019;59:567-76. [Crossref] [PubMed]
- Riva N, Chaabane L, Peviani M, Ungaro D, Domi T, Dina G, Bianchi F, Spano G, Cerri F, Podini P, Corbo M, Carro UD, Comi G, Bendotti C, Quattrini A. Defining peripheral nervous system dysfunction in the SOD-1G93A transgenic rat model of amyotrophic lateral sclerosis. J Neuropathol Exp Neurol 2014;73:658-70. [Crossref] [PubMed]
- Devigili G, Uçeyler N, Beck M, Reiners K, Stoll G, Toyka KV, Sommer C. Vasculitis-like neuropathy in amyotrophic lateral sclerosis unresponsive to treatment. Acta Neuropathol 2011;122:343-52. [Crossref] [PubMed]
- Mori A, Nodera H, Takamatsu N, Maruyama-Saladini K, Osaki Y, Shimatani Y, Oda M, Izumi Y, Kaji R. Sonographic evaluation of cervical nerve roots in ALS and its clinical subtypes. J Med Invest 2016;63:54-7. [Crossref] [PubMed]