Assessment of bone marrow fat by 3-Tesla magnetic resonance spectroscopy in patients with chronic kidney disease
Introduction
Bone marrow fat is the main tissue filling porous trabecular bone in adults and represents the third largest adipose depot after subcutaneous and visceral fat. For many years its exact role in the regulation and development of bone environment has been ignored. Several studies have recently revealed that bone marrow fat has important endocrine and paracrine functions, and influences hematopoiesis, osteogenesis and whole-body-energy metabolism (1-3). Adipogenesis in bone marrow cavities has been shown to be associated with aging, estrogen deficiency and various metabolic diseases (4-7). Moreover, it has been found that high bone marrow fat correlates with low bone volume and with vertebral fractures risk independently of bone volume, suggesting a possible role of marrow adipose tissue in the pathogenesis of bone deterioration (8,9). Consequently, quantification of bone marrow fat has recently gained an increasing interest. Proton magnetic resonance spectroscopy (1H MRS) is a commonly used imaging method for non-invasive quantification of bone marrow fat. It uses the signal of hydrogen atoms derived from different tissues to differentiate lipids from water peak, providing quantitative assessment of proton density fat fraction (PDFF) with a high accuracy and a strong correlation with fat content values from biopsy (8,10-13).
Comparing to other quantitative magnetic resonance (MR) techniques applied in bone marrow (e.g., Dixon imaging), MRS provides more detailed information on chemical shift dimension, enabling the measurement not only of PDFF but also of the distinct lipids contained in bone marrow. However, a robust MRS extraction of small components of lipids in bone marrow can be challenging due to the presence of broad water peaks. Other technical aspects of MRS, such as a relatively long acquisition time and the presence of potential chemical shift displacements and T2 decay effects, should be considered and adequately balanced (13,14).
MRS quantification of PDFF has been compared between 1.5 and 3.0 Tesla, demonstrating an improvement of spectral resolution and signal-to-noise ratio at the higher field strength (15,16).
Recently, chemical shift encoding-based water-fat imaging, also known as Dixon imaging, has been proposed for measuring PDFF in bone marrow (17,18). In contrast to MRS, it allows a spatially resolved quantification of PDFF, that is advantageous in presence of a heterogeneous distribution of bone marrow (e.g., proximal femur, spine), and has a short scanning time. However, the accuracy of PDFF assessment by Dixon imaging is limited by several confounding factors (e.g., multiple peaks in the fat spectrum, T1-bias effects, phase errors, T2 decay effects) that make necessary further technical improvements, especially concerning the signal model (13,14,19).
Hence, despite of its limitations, MRS is currently the preferable quantitative MR technique for bone marrow fat evaluation at various skeletal sites, with vertebral bone marrow representing the region most extensively investigated in MRS studies (8,12,15).
So far, researchers have focused on MRS evaluation of bone marrow changes in patients with chronic disorders such as osteoporosis or type 2 diabetes mellitus (8,16,20).
Few investigations exist regarding the assessment of bone marrow fat by MRS in patients with renal osteodystrophy (21,22).
Renal osteodystrophy is an abnormal bone histomorphometry associated with chronic kidney disease (CKD) and represents one component of the systemic symptoms of CKD-mineral bone disorder (23-25).
Patients with CKD have a wide spectrum of findings on bone biopsy from high-turnover forms associated with hyperparathyroidism to adynamic bone disorders or osteomalacia related to mineralization defects. Various paracrine and endocrine signals participate in bone remodeling, with parathyroid hormone (PTH) playing a central role. In CKD patients PTH metabolism is altered and secondary hyperparathyroidism is an almost universal complication of advanced stages of disease due to many factors such as hypocalcemia, retention of phosphorus, decreases in the levels of calcitriol, intrinsic alterations within the parathyroid gland and skeletal resistance to the actions of PTH (26).
Sustained high PTH levels in CKD cause a high turnover state in bone, where bone resorption and, in a lesser degree, bone formation are stimulated. The disturbed osteoblast activity results in disorganized bone formation, with an excessive amount of non-mineralized component of bone, leading to skeletal fragility and subsequent increased fracture risk, particularly at the peripheral skeleton (27).
In fact, fractures are two to four times more prevalent in patients with CKD compared to the general population (24,28).
Fracture risk is determined by bone strength, that depends on bone mass and bone quality. Bone mass is traditionally measured by bone mineral density using dual energy X-ray absorptiometry (DXA) but in the last years other bone indices such as volumetric bone mineral density (vBMD) using quantitative computed tomography (QCT) have been shown to be more accurate than DXA bone measurements for prediction of future fracture in CKD patients as well as in general population (29-31). Bone quality is determined by some bone tissue properties such as bone turnover, mineralization, microarchitecture, matrix composition. In renal osteodystrophy bone abnormalities imply defects of both bone mass and bone quality, that are mainly related to PTH excess. However, the evidence of an increased fracture risk in CKD patients even at low levels of PTH suggests that there are others factors dependent on kidney function that impair bone health (32,33). Since the characteristics of CKD are similar to those of aging process, but with an accelerated deterioration of tissues and cellular functions (34), it is possible to hypothesize that, as observed in aging population (4,9), also in patients with impaired kidney function bone marrow adipogenesis may play an important role in bone metabolism.
Only two previous studies investigated vertebral bone marrow fat content in CKD, demonstrating an increased bone marrow fat deposition in patients with CKD compared to healthy controls (21,22). However, neither of them evaluated the association between bone marrow fat and severity of CKD.
In the present study, we used MRS to assess PDFF at lumbar spine in patients with CKD. In this population, we correlated PDFF measurements with vBMD obtained by QCT and investigated if PDFF is associated with severity of renal function impairment.
Methods
Study population
CKD subjects were recruited consecutively for participation in the study, and the inclusion criteria were age ≥45 years old, estimated GFR ≤45 mL/min/1.732 m2 (MDRD formula) in the last 6 months, elevated PTH above the normal range (65 pg/mL) in the past year and QCT examination as part of diagnostic workup. Females who were pregnant or nursing, a prior history of lumbar fracture, general contraindications to MRI/MRS exam (e.g., IUD, pacemaker), history of haematological malignancy, chemotherapy or radiation therapy, and participants who were unable to consent were also excluded.
The healthy control volunteers were asymptomatic, had no history of spinal or other disease, and had not received any medicine that could have affected their bone marrow. They were matched to CKD patients based on race, gender, and age by 10 years. Between January 2019 and February 2020, 42 people (34 CKD patients and 8 healthy controls) were enrolled. Four CKD patients were removed due to technical issues with MRS scans, leaving 38 people in the analysis.
The study was conducted in accordance with the Declaration of Helsinki (as revised in 2013). The study was approved by the Ethics Committee of IRCCS “Casa Sollievo della Sofferenza” Hospital (No. 12/CE). Written informed consent was obtained from all participants.
Study procedures
To evaluate the repeatability of the MRS analysis, eight subjects (all healthy controls) were scanned twice at lumbar vertebrae on the same day with repositioning between the two scans. The repositioning of the subjects was done by the same technologist. Each lumbar vertebral level L1, L2 and L3 were selected for MRS analysis.
In CKD subjects, QCT and MR examinations of the lumbar spine were performed on the same day. For each patient, a blood sample for PTH measurement was collected at the time of MRS scan. GFR values were taken from the last clinically available assessment, within the last 6 months. CKD patients were stratified in three groups according to GFR levels in order to examine the influence of increasing impairment of renal function on bone marrow fat content. The group 1 included patients with moderate chronic renal insufficiency (GFR from 45 to 30 mL/min), the group 2 patients with severe chronic renal insufficiency (GFR from 29 to 15 mL/min) and the group 3 patients with the most advanced stage of renal failure (GFR <15 mL/min).
QCT examination
vBMD of the lumbar spine was assessed by QCT, a three-dimensional method which measures trabecular BMD in milligrams per cubic centimeter by indirectly quantifying hydroxyapatite in comparison to a reference phantom.
All examinations were performed by using a 16-row multidetector CT scanner (Aquilion, Toshiba Medical System, Tokyo, Japan). A non-simultaneous calibration system was used (Lumbar Reference Simulator, CIRS, Norfolk, VA, USA). The scan protocol was standardized and consisted of a tube voltage of 120 kVp and a tube current of 200 mAs. Slice thickness was 3 mm. QCT scans were analyzed on a workstation using the dedicated software (Mindways QCT Pro, Austin, USA). Elliptical regions of interest (ROIs) were manually placed by an experienced operator in the trabecular part of vertebral bodies from L1 to L3, avoiding cortical bone, basivertebral veins and sclerotic foci (Figure 1). The ROIs size was kept the same for all QCT analyses.
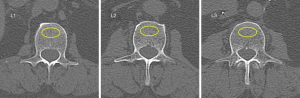
For each patient, vBMD values derived from the three lumbar vertebrae were annotated and the mean lumbar vBMD was computed by averaging vBMD measurements.
The precision error of vBMD measurements by QCT was previously reported (CV =1.8%) (35).
MR spectroscopy
All MR examinations were performed on a 3T MRI scanner (Philips Ingenia 3.0) using a phased array spine coil.
Subjects were in supine position with knees supported by a form wedge to reduce spine curvature and motion by improving patient comfort. The imaging protocol included T1-weighted TSE sequence [sagittal plane, repetition time (TR) 450–700 ms, echo time (TE) 9 ms, turbo factor 5, field of view (FOV) 32 cm, slice thickness 3 mm, spacing 0.3 mm, acquisition time 3 min and 21 s] and T2-weighted mDIXON TSE sequences (sagittal and coronal planes, TR 2,500–4,000 ms, TE 80 ms, turbo factor 19, FOV 32 cm, slice thickness 3 mm, spacing 0.3 mm, acquisition time 3 min and 5 s).
Proton MR spectroscopy was performed using a single voxel spectroscopy technique (voxel size 15×15×15 mm3). The T2-weighted sequences were used to guide the optimal positioning of volumes of interest (VOI) within the selected lumbar vertebrae (L1–L3). Unless spine fractures, degenerative alterations or focal lesions (e.g., hemangiomas) were identified, the VOI was placed in the center of the vertebral bodies, avoiding the posterior venous plexuses. The voxel size was maintained the same for each vertebral level and for each subject.
The Point Resolved Spectroscopy (PRESS) method was used, and the spectroscopy sequence parameters were: TR 2,000 ms, TE 40 ms, spectral bandwidth 2,000 Hz, samples 1,024, voxel volume 3.4 cm3, acquisition time 5 min and 24 s).
Neither pre-saturation bands nor water suppression was used.
A second order shimming method was applied to optimize tissue homogeneity in MRS voxel. A mean FWHM <30 Hz was obtained.
MR image analysis
All spectroscopy data were transferred to a research workstation and analyzed with Spectroview software (Philips Medical Systems, Best, The Netherlands) using Gaussian line shapes and frequency-based methods. After reconstruction, corrections for phase, frequency shift, and baseline distortion were performed on spectral data. The spectrum acquired included a water peak at 4.77 ppm and four lipid peaks, consisting of olefinic protons CH=CH- at 5.35 ppm, methylene protons -CH=CHCH2- at 2.06 ppm, bulk methylene protons -[CH2]n- at 1.3 ppm and terminal methyl protons -CH3 at 0.9 ppm (Figure 2).
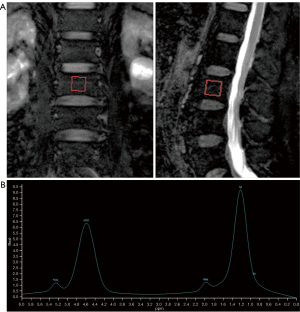
All the peaks were fitted to obtain their line widths and areas.
After fitting, PDFF was calculated as the ratio of all the fat peak areas to the sum of all the fat peak and water peak areas and expressed as percentage. The measurement of PDFF was obtained at each vertebral level. Moreover, for each participant, the average PDFF value derived from all three vertebral levels was calculated.
Statistical analysis
The repeatability of MRS for PDFF measurements in healthy controls was evaluated using coefficients of variation (CV) and Bland-Altman plots; the variability or precision of PDFF measurements was expressed as coefficient of repeatability (CR).
Paired t-tests were used to compare PDFF values at each lumbar site between CKD patients and healthy controls matched according to race, gender and age ±10 years.
Spectroscopic measurements of PDFF were correlated with vBMD values using Pearson’s correlation coefficients and multiple linear regression analysis was applied to estimate the effects of age on vBMD and PDFF measurements. Pearson’s correlation was also calculated to determine the association of PDFF and vBMD with GFR and PTH values.
One-way analysis of variation (ANOVA) was used to identify significant differences in PDFF and vBMD among the three groups of patients formed according to the severity of CKD. If a statistical significance was present, Tukey post hoc test was applied in order to find out which groups differed. Results were expressed as mean ± standard deviation. All statistical analysis was performed using SPSS software (version 29.0) and MedCalc software (version 22.005). Statistical significance was defined as P<0.05.
Results
Study population
Demographics characteristics of study population are presented in Table 1. In CKD patients, mean GFR value was 21.5±8.8 mL/min and mean PTH value was 149.2±53.1 pg/mL. When stratified by GFR, 23.3% (7/30) of patients had a GFR from 45 to 30 mL/min (group 1), 56.7% (17/30) had a GFR from 29 to 15 mL/min (group 2) and 20% (6/30) had a GFR <15 mL/min (group 3).
Table 1
Values | Controls | CKD patients |
---|---|---|
Sample size (n) | 8 | 30 |
Age (years) | 56.3±12.4 | 59.6±11.5 |
Males (%) | 75 | 70 |
Caucasian (%) | 100 | 100 |
BMI (kg/m2) | 26.9±4.5 | 27.1±5.1 |
Data are expressed as mean ± standard deviation. CKD, chronic kidney disease; BMI, body mass index.
Repeatability of PDFF spectroscopic measurements
Bone marrow spectrum was dominated by water peak (4.7 ppm) and an intense lipid peak (1.3 ppm) deriving from bulk-methylene protons. Olefinic (5.35 ppm) and methylenic (2.06 ppm) protons peaks were also well identified, though with smaller tips compared to the two main peaks. Terminal methyl protons peak (0.9 ppm) was more difficult to resolve due to the overlapping with the broad lipid peak from bulk-methylene protons.
The mean bone marrow fat, measured in the eight subjects was 53.1%±8.6% at L1, 56.3%±7% at L2 and 57.1%±7.3% at L3.
MRS measurements of PDFF at all vertebral levels were highly repeatable, with CV values ranging from 2.1% to 4.6% (Table 2). The Bland-Altman plots, used to visually assess the differences between repeated MRS measurements at each vertebral level, showed a low variability with CR values of 0.041 for L1, 0.090 for L2 and 0.079 for L3 (Figure 3).
Table 2
Vertebral level | Mean ± SD (%) | CV (%) |
---|---|---|
L1 | 53.1±8.6 | 2.1 |
L2 | 56.3±7 | 4.6 |
L3 | 57.1±7.3 | 4.3 |
SD, standard deviation; CV, coefficient of variation.
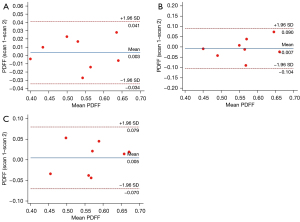
Differences in PDFF between CKD patients and controls
At each lumbar spine site, the mean marrow fat content was significantly elevated in patients compared to controls (Table 3). The mean fat content at L1 was 70.5%±8.3% in subjects with CKD versus 53.1%±8.6% in controls (P<0.001). At L2, the bone marrow fat was 71.5%±11.9% in CKD versus 56.3%±7% in controls (P<0.001). Similarly, at L3, the bone marrow fat was 72.4%±9.5% versus 57.1%±7.3% (P=0.02).
Table 3
Vertebral level | CKD patients (n=30) | Controls (n=8) | P value |
---|---|---|---|
L1 | 70.5±8.3 | 53.1±8.6 | <0.001 |
L2 | 71.5±11.9 | 56.3±7 | <0.001 |
L3 | 72.4±9.5 | 57.1±7.3 | 0.02 |
All levels (L1–L3) | 71.4±8.7 | 55.5±7.6 | <0.001 |
Data are expressed as mean ± standard deviation. CKD, chronic kidney disease.
Correlation between PDFF and vBMD
The average values of vBMD, measured in all CKD patients, were 104.1±32.6 mg/cm3 at L1, 97.1±26.4 mg/cm3 at L2 and 93.0±34.7 mg/cm3 at L3. The mean value of vBMD at all lumbar sites was 98.1±27.9 mg/cm3.
Mean PDFF showed a strong negative correlation with vBMD (r=−0.71; P<0.001) as represented on Figure 4. In multiple linear regression analysis, age was a significant predictor of vBMD (P=0.001; R2=0.33); conversely, no significant interaction was found between age and PDFF.
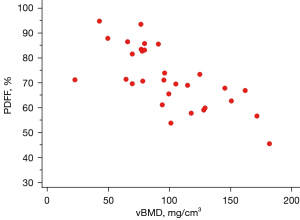
Association of PDFF and vBMD with CKD severity and PTH values
Results of Pearson’s correlation test revealed a good negative correlation between PDFF and GFR (r=−0.53; P=0.003). Moreover, one-way ANOVA showed statistically significant differences of fat fraction in the three groups of CKD patients stratified by GFR (P=0.002). Specifically, PDFF was lower in group 1 (65.4%±0.8%) compared to group 2 (69.2%±1.2%) and higher in group 3 (82.1%±1.9%) compared to the other two groups (Tukey: P<0.05) (Figure 5).
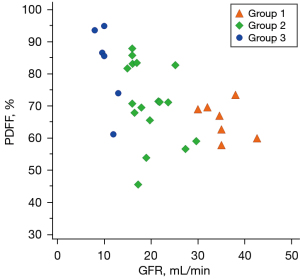
There was no significant correlation between mean PDFF and PTH values (P>0.05). The mean vBMD was 105±37.9 mg/cm3 in group 1, 96.1±47.8 mg/cm3 in group 2 and 93.2±28.4 mg/cm3 in group 3, however its values were no significantly different among the three CKD groups and no significantly correlated with GFR and PTH (P>0.05).
Discussion
In this study, we used MRS at 3T for evaluating bone marrow fat at lumbar spine. The repeatability of bone marrow fat measurements by MRS was optimal at all lumbar vertebral levels. Recent studies have documented the reliability of MRS in marrow fat quantification and the advantages of acquiring spectral data with a high field strength (13,15,16,18). In particular, 3T MR technique has a high signal-to-noise ratio that allows the acquisition of higher quality and more easily quantifiable spectra in smaller voxels and/or in shorter times. Moreover, the high spectral resolution at 3T helps to better detect even lipid peaks with low signal intensity. As known, MRS quantification of PDFF may be challenging for the presence of broad water and bulk-methylene lipid peaks due to susceptibility differences between bone and marrow. This results in a poor identification of the remaining lipid peaks that, although small, contribute to the total lipid signal (13,14,17). The use of MRS at high field strength allows to accurately measure signals derived from olefinic protons (5.31 ppm), even if partially overlapped with the water peak, and from methylene protons (2.06 ppm), that have a weak intensity. Fitting MRS spectra in either frequency-domain or time-domain may also improve the differentiation between adjacent peaks in the spectrum, and the accuracy of PDFF quantification (13,17). In our study, we obtained a good spectral quality, as shown in Figure 2; olefinic and methylene protons peaks were definitely or partially resolved in all spectra. However, MRS measurements of the individual lipid components were less repeatable than measurements of the total fat fraction, with CV values ranged between 13.1% and 15.4% (data not shown). Despite the great advantages of the high field MR spectroscopy, further developments in both acquisition and post processing methods are needed to better overcome potential limitations of 1H-MRS and facilitate its application in the clinical routine. In our research, we focused on bone marrow fat quantification by MRS in patients with CKD and compared them with healthy subjects. Our results demonstrated that MRS measurements of PDFF were significantly elevated in patients with chronic renal impairment. So far, few studies have investigated bone marrow composition by MR imaging in patient with CKD (21,22). Moorthi et al. found that bone marrow fat by MRS was significantly higher in CKD patients compared to controls (21). Woods et al. also observed an association between CKD and spectroscopic measurements of bone marrow fat, with higher values of in subjects with GFR <45 mL/min compared to those with GFR >60 mL/min (22). Our data are consistent with these findings.
The novel finding of our study was that bone marrow fat and vBMD were inversely related in patients with CKD. In this population, we found elevated values of PDFF and low levels of vBMD, with a significant negative correlation between the two parameters, both measured at lumbar spine respectively by MRS and QCT. We chose QCT instead of DXA because this three-dimensional technique allows obtaining volumetric BMD measurements, that are more consistent with those of MR spectroscopy than bi-dimensional BMD measurements of DXA.
In addition, we explored the possible connection between bone marrow fat and severity of CKD. To our knowledge, this investigation has not been performed so far.
In aging population, MRS measurements of bone marrow fat correlate with osteopenia and osteoporosis assessed on the basis of BMD and vertebral fractures, as demonstrated in several reports (8,9,36).
The similarities among these studies and our results suggest that in both CKD and aging there is an increased marrow fat deposition, whose cause is still unknown. CKD represents a model of premature aging, characterized by an accelerated deterioration of physiological cellular and tissue functions. In this condition, the common cellular precursors of adipocytes and osteoblasts (i.e., mesenchymal stem cells) are functionally altered due to the accumulation of oxidative stress, leading to a preferential differentiation towards adipocyte lineage in bone marrow (34,37). This observation suggests that an impaired kidney function affects bone marrow environment. Accordingly, the evidence of a reciprocal relationship between marrow fat and bone mineral density supports the observation that bone weakening depends not only on trabecular bone loss but also on increased adipogenesis. In fact, excessive amounts of marrow fat determine the reduction of bone mass, and consequently of bone strength. In addition, a disproportionate adipogenesis may inhibit normal osteoblast activity, resulting in bone loss and alteration of bone quality. These mechanisms could be responsible for the higher fracture rates in CKD patients compared to general population (24,28).
Bone abnormalities affect almost all patients with CKD requiring dialysis and the majority of patients with CKD stages 3–5 (27,38). So, we hypothesized an increased marrow fat in patients with late stages of chronic renal insufficiency. In effect, our results demonstrated that PDFF increased significantly with the decline of GFR, suggesting that the normal mineral and endocrine functions disrupted in CKD patients play an important role in modulation of marrow adipogenesis. In our study, changes in bone marrow fat related to CKD were not affected by age. This finding, though unexpected, was consistent with the results of Woods et al. (22). A possible explanation may be the relatively young age of our cohort of patients (mean age 59.6±11.5 years).
We also investigated the relationship between marrow fat content and blood levels of PTH, that is considered a valuable biomarker of bone remodeling in CKD. Our results did not show a significant association between these two parameters. A small portion of CKD patients received treatment for secondary hyperparathyroidism and the effects of drugs may have influenced blood levels of PTH. In the future, we will correlate bone marrow fat with biochemical markers of bone turnover in patients with chronic renal insufficiency, at baseline and after specific treatment to correct secondary hyperparathyroidism. These observations may help to understand if bone marrow fat composition is a “reversible” condition that changes with clinical improvement of mineral disorder related to CKD.
An interesting finding of our analysis was the evidence of no association between vBMD and severity of CKD. Moreover, vBMD did not show a significant correlation with PTH values. In accordance with our results, previous reports conducted on CKD patients demonstrated that while at hip hyperparathyroidism was associated with low vBMD, resulting in high fracture risk, at spine this association was less strong (39,40). Whether in CKD patients the increased marrow fat adipogenesis may explain the high bone fragility and fracture risk at lumbar spine, independently of PTH, it deserves further studies.
The current study has some limitations. The population size is relatively small, in particular healthy controls, and measurements of vBMD by QCT were not available in healthy subjects. It should be emphasized that several studies have already examined association between marrow fat and bone density in healthy population (8,9,36,41). However, further investigations are needed to recruit a larger sample of subjects for comparing their bone composition with those of CKD patients.
In our research, we did not have histological data derived from bone biopsy to confirm MRS marrow fat measurements in CKD patients. Nevertheless, a recent study of Cohen et al. performed in osteoporotic and control subjects demonstrated a correlation between marrow fat content in trans-iliac crest biopsy and lumbar spine marrow fat content by MRS (42). Although related to a different study population, these results contribute to better validate MRS in bone marrow fat assessment.
Lastly, in a small number of our study population eventual drug therapies affecting bone and mineral metabolism may have had effects on blood PTH values measured in those patients, influencing our results in terms of correlation with marrow fat content. Regardless of that, there is a need to understand if CKD-induced disturbances of PTH metabolism may have a role in changes of bone marrow composition.
Conclusions
This study demonstrates that a decrease in bone density within lumbar vertebrae is associated with a significant increase of bone marrow fat in CKD patients. Moreover, bone marrow fat modifications correlate with the severity of renal function impairment.
Based on our results, we believe that 3T MRS quantification of bone marrow fat is reliable and highly repeatable, indicating its possible role as quantitative imaging biomarker for bone marrow changes in CKD patients.
Acknowledgments
Funding: None.
Footnote
Conflicts of Interest: All authors have completed the ICMJE uniform disclosure form (available at https://qims.amegroups.com/article/view/10.21037/qims-23-530/coif). GG serves as an unpaid editorial board member of Quantitative Imaging in Medicine and Surgery. The other authors have no conflicts of interest to declare
Ethical Statement: The authors are accountable for all aspects of the work in ensuring that questions related to the accuracy or integrity of any part of the work are appropriately investigated and resolved. The study was conducted in accordance with the Declaration of Helsinki (as revised in 2013). The study was approved by the Ethics Committee of IRCCS “Casa Sollievo della Sofferenza” Hospital (No. 12/CE). Written informed consent was obtained from all patients in this study.
Open Access Statement: This is an Open Access article distributed in accordance with the Creative Commons Attribution-NonCommercial-NoDerivs 4.0 International License (CC BY-NC-ND 4.0), which permits the non-commercial replication and distribution of the article with the strict proviso that no changes or edits are made and the original work is properly cited (including links to both the formal publication through the relevant DOI and the license). See: https://creativecommons.org/licenses/by-nc-nd/4.0/.
References
- Li Z, Hardij J, Bagchi DP, Scheller EL, MacDougald OA. Development, regulation, metabolism and function of bone marrow adipose tissues. Bone 2018;110:134-40. [Crossref] [PubMed]
- Wang H, Leng Y, Gong Y. Bone Marrow Fat and Hematopoiesis. Front Endocrinol (Lausanne) 2018;9:694. [Crossref] [PubMed]
- Rosen CJ, Klibanski A. Bone, fat, and body composition: evolving concepts in the pathogenesis of osteoporosis. Am J Med 2009;122:409-14. [Crossref] [PubMed]
- Justesen J, Stenderup K, Ebbesen EN, Mosekilde L, Steiniche T, Kassem M. Adipocyte tissue volume in bone marrow is increased with aging and in patients with osteoporosis. Biogerontology 2001;2:165-71. [Crossref] [PubMed]
- Yu EW, Greenblatt L, Eajazi A, Torriani M, Bredella MA. Marrow adipose tissue composition in adults with morbid obesity. Bone 2017;97:38-42. [Crossref] [PubMed]
- Shu JB, Kim TY. Bone marrow adiposity in diabetes and clinical interventions. Curr Opin Endocrinol Diabetes Obes 2022;29:303-9. [Crossref] [PubMed]
- Paccou J, Badr S, Lombardo D, Khizindar H, Deken V, Ruschke S, Karampinos DC, Cotten A, Cortet B. Bone Marrow Adiposity and Fragility Fractures in Postmenopausal Women: The ADIMOS Case-Control Study. J Clin Endocrinol Metab 2023;108:2526-36. [Crossref] [PubMed]
- Griffith JF, Yeung DK, Antonio GE, Lee FK, Hong AW, Wong SY, Lau EM, Leung PC. Vertebral bone mineral density, marrow perfusion, and fat content in healthy men and men with osteoporosis: dynamic contrast-enhanced MR imaging and MR spectroscopy. Radiology 2005;236:945-51. [Crossref] [PubMed]
- Schwartz AV, Sigurdsson S, Hue TF, Lang TF, Harris TB, Rosen CJ, Vittinghoff E, Siggeirsdottir K, Sigurdsson G, Oskarsdottir D, Shet K, Palermo L, Gudnason V, Li X. Vertebral bone marrow fat associated with lower trabecular BMD and prevalent vertebral fracture in older adults. J Clin Endocrinol Metab 2013;98:2294-300. [Crossref] [PubMed]
- Machann J, Stefan N, Schick F. (1)H MR spectroscopy of skeletal muscle, liver and bone marrow. Eur J Radiol 2008;67:275-84. [Crossref] [PubMed]
- Pichardo JC, Milner RJ, Bolch WE. MRI measurement of bone marrow cellularity for radiation dosimetry. J Nucl Med 2011;52:1482-9. [Crossref] [PubMed]
- Griffith JF, Yeung DK, Chow SK, Leung JC, Leung PC. Reproducibility of MR perfusion and (1)H spectroscopy of bone marrow. J Magn Reson Imaging 2009;29:1438-42. [Crossref] [PubMed]
- Karampinos DC, Ruschke S, Dieckmeyer M, Diefenbach M, Franz D, Gersing AS, Krug R, Baum T. Quantitative MRI and spectroscopy of bone marrow. J Magn Reson Imaging 2018;47:332-53. [Crossref] [PubMed]
- Cordes C, Baum T, Dieckmeyer M, Ruschke S, Diefenbach MN, Hauner H, Kirschke JS, Karampinos DC. MR-Based Assessment of Bone Marrow Fat in Osteoporosis, Diabetes, and Obesity. Front Endocrinol (Lausanne) 2016;7:74. [Crossref] [PubMed]
- Pansini VM, Monnet A, Salleron J, Penel G, Migaud H, Cotten A. Reproducibility of 1H MR spectroscopy of hip bone marrow at 3 Tesla. J Magn Reson Imaging 2012;36:1445-9. [Crossref] [PubMed]
- Li X, Kuo D, Schafer AL, Porzig A, Link TM, Black D, Schwartz AV. Quantification of vertebral bone marrow fat content using 3 Tesla MR spectroscopy: reproducibility, vertebral variation, and applications in osteoporosis. J Magn Reson Imaging 2011;33:974-9. [Crossref] [PubMed]
- Karampinos DC, Melkus G, Baum T, Bauer JS, Rummeny EJ, Krug R. Bone marrow fat quantification in the presence of trabecular bone: initial comparison between water-fat imaging and single-voxel MRS. Magn Reson Med 2014;71:1158-65. [Crossref] [PubMed]
- Schmeel FC, Vomweg T, Träber F, Gerhards A, Enkirch SJ, Faron A, Sprinkart AM, Schmeel LC, Luetkens JA, Thomas D, Kukuk GM. Proton density fat fraction MRI of vertebral bone marrow: Accuracy, repeatability, and reproducibility among readers, field strengths, and imaging platforms. J Magn Reson Imaging 2019;50:1762-72. [Crossref] [PubMed]
- Peterson P, Trinh L, Månsson S. Quantitative (1) H MRI and MRS of fatty acid composition. Magn Reson Med 2021;85:49-67. [Crossref] [PubMed]
- Baum T, Yap SP, Karampinos DC, Nardo L, Kuo D, Burghardt AJ, Masharani UB, Schwartz AV, Li X, Link TM. Does vertebral bone marrow fat content correlate with abdominal adipose tissue, lumbar spine bone mineral density, and blood biomarkers in women with type 2 diabetes mellitus? J Magn Reson Imaging 2012;35:117-24. [Crossref] [PubMed]
- Moorthi RN, Fadel W, Eckert GJ, Ponsler-Sipes K, Moe SM, Lin C. Bone marrow fat is increased in chronic kidney disease by magnetic resonance spectroscopy. Osteoporos Int 2015;26:1801-7. [Crossref] [PubMed]
- Woods GN, Ewing SK, Sigurdsson S, Kado DM, Ix JH, Hue TF, Eiriksdottir G, Xu K, Gudnason V, Lang TF, Vittinghoff E, Harris TB, Rosen CJ, Li X, Schwartz AV. Chronic Kidney Disease Is Associated With Greater Bone Marrow Adiposity. J Bone Miner Res 2018;33:2158-64. [Crossref] [PubMed]
- Moe S, Drüeke T, Cunningham J, Goodman W, Martin K, Olgaard K, Ott S, Sprague S, Lameire N, Eknoyan G. Definition, evaluation, and classification of renal osteodystrophy: a position statement from Kidney Disease: Improving Global Outcomes (KDIGO). Kidney Int 2006;69:1945-53. [Crossref] [PubMed]
- Pimentel A, Ureña-Torres P, Bover J, Luis Fernandez-Martín J, Cohen-Solal M. Bone Fragility Fractures in CKD Patients. Calcif Tissue Int 2021;108:539-50. [Crossref] [PubMed]
- Gerakis A, Hadjidakis D, Kokkinakis E, Apostolou T, Raptis S, Billis A. Correlation of bone mineral density with the histological findings of renal osteodystrophy in patients on hemodialysis. J Nephrol 2000;13:437-43.
- Evenepoel P, Bover J, Ureña Torres P. Parathyroid hormone metabolism and signaling in health and chronic kidney disease. Kidney Int 2016;90:1184-90. [Crossref] [PubMed]
- Aguilar A, Gifre L, Ureña-Torres P, Carrillo-López N, Rodriguez-García M, Massó E, da Silva I, López-Báez V, Sánchez-Bayá M, Prior-Español Á, Urrutia M, Paul J, Bustos MC, Vila A, Garnica-León I, Navarro-González JF, Mateo L, Bover J. Pathophysiology of bone disease in chronic kidney disease: from basics to renal osteodystrophy and osteoporosis. Front Physiol 2023;14:1177829. [Crossref] [PubMed]
- Jansz TT, Goto NA, van Ballegooijen AJ, Willems HC, Verhaar MC, van Jaarsveld BC. The prevalence and incidence of vertebral fractures in end-stage renal disease and the role of parathyroid hormone. Osteoporos Int 2020;31:515-24. [Crossref] [PubMed]
- Li N, Li XM, Xu L, Sun WJ, Cheng XG, Tian W. Comparison of QCT and DXA: Osteoporosis Detection Rates in Postmenopausal Women. Int J Endocrinol 2013;2013:895474. [Crossref] [PubMed]
- Mao SS, Li D, Syed YS, Gao Y, Luo Y, Flores F, Child J, Cervantes M, Kalantar-Zadeh K, Budoff MJ. Thoracic Quantitative Computed Tomography (QCT) Can Sensitively Monitor Bone Mineral Metabolism: Comparison of Thoracic QCT vs Lumbar QCT and Dual-energy X-ray Absorptiometry in Detection of Age-relative Change in Bone Mineral Density. Acad Radiol 2017;24:1582-7. [Crossref] [PubMed]
- Malluche HH, Davenport DL, Cantor T, Monier-Faugere MC. Bone mineral density and serum biochemical predictors of bone loss in patients with CKD on dialysis. Clin J Am Soc Nephrol 2014;9:1254-62. [Crossref] [PubMed]
- Danese MD, Kim J, Doan QV, Dylan M, Griffiths R, Chertow GM. PTH and the risks for hip, vertebral, and pelvic fractures among patients on dialysis. Am J Kidney Dis 2006;47:149-56.
- Coco M, Rush H. Increased incidence of hip fractures in dialysis patients with low serum parathyroid hormone. Am J Kidney Dis 2000;36:1115-21. [Crossref] [PubMed]
- Figuer A, Bodega G, Tato P, Valera G, Serroukh N, Ceprian N, de Sequera P, Morales E, Carracedo J, Ramírez R, Alique M. Premature Aging in Chronic Kidney Disease: The Outcome of Persistent Inflammation beyond the Bounds. Int J Environ Res Public Health 2021;18:8044. [Crossref] [PubMed]
- Chiodini I, Guglielmi G, Battista C, Carnevale V, Torlontano M, Cammisa M, Trischitta V, Scillitani A. Spinal volumetric bone mineral density and vertebral fractures in female patients with adrenal incidentalomas: the effects of subclinical hypercortisolism and gonadal status. J Clin Endocrinol Metab 2004;89:2237-41. [Crossref] [PubMed]
- Woods GN, Ewing SK, Sigurdsson S, Kado DM, Eiriksdottir G, Gudnason V, Hue TF, Lang TF, Vittinghoff E, Harris TB, Rosen C, Xu K, Li X, Schwartz AV. Greater Bone Marrow Adiposity Predicts Bone Loss in Older Women. J Bone Miner Res 2020;35:326-32. [Crossref] [PubMed]
- Li J, Lu L, Liu Y, Yu X. Bone marrow adiposity during pathologic bone loss: molecular mechanisms underlying the cellular events. J Mol Med (Berl) 2022;100:167-83. [Crossref] [PubMed]
- Hu L, Napoletano A, Provenzano M, Garofalo C, Bini C, Comai G, La Manna G. Mineral Bone Disorders in Kidney Disease Patients: The Ever-Current Topic. Int J Mol Sci 2022;23:12223. [Crossref] [PubMed]
- Bezerra de Carvalho KS, Vasco RFV, Custodio MR, Jorgetti V, Moysés RMA, Elias RM. Chronic kidney disease is associated with low BMD at the hip but not at the spine. Osteoporos Int 2019;30:1015-23. [Crossref] [PubMed]
- Huang JF, Zheng XQ, Sun XL, Zhou X, Liu J, Li YM, Wang XY, Zhang XL, Wu AM. Association between Bone Mineral Density and Severity of Chronic Kidney Disease. Int J Endocrinol 2020;2020:8852690. [Crossref] [PubMed]
- Yu A, Huang M, Wang L, Zhang Y, Li K, Lou L, Liang W, Blake GM, Deng W, Cheng X. Age and gender differences in vertebral bone marrow adipose tissue and bone mineral density, based on MRI and quantitative CT. Eur J Radiol 2023;159:110669. [Crossref] [PubMed]
- Cohen A, Shen W, Dempster DW, Zhou H, Recker RR, Lappe JM, Kepley A, Kamanda-Kosseh M, Bucovsky M, Stein EM, Nickolas TL, Shane E. Marrow adiposity assessed on transiliac crest biopsy samples correlates with noninvasive measurement of marrow adiposity by proton magnetic resonance spectroscopy ((1)H-MRS) at the spine but not the femur. Osteoporos Int 2015;26:2471-8. [Crossref] [PubMed]