Myocardial infarct size for predicting improvements in cardiac function in patients with ischemic cardiomyopathy following coronary artery bypass grafting
Introduction
Coronary artery bypass grafting (CABG) is currently the standard therapeutic strategy for patients with ischemic cardiomyopathy (ICM) (1). However, this is a major surgical procedure associated with a high risk of death or adverse outcomes, and some patients fail to achieve any postoperative improvement in cardiac function (2,3). In one study, about 40% of patients with ICM did not exhibit improved cardiac function following CABG, with this lack of cardiac functional improvement being associated with an increased risk of all-cause mortality (4).
At present, late gadolinium enhancement-cardiac magnetic resonance (LGE-CMR) imaging is considered to be the most significant and commonly used technique for myocardial infarct examination (5,6). In previous reports, LGE-CMR was used to assess myocardial infarct segments (LGE penetration degree >50%) and to predict improvements in cardiac function based on these findings, but some reports have suggested that there is some chance of improvement following revascularization in roughly one-third of myocardial infarct segments (7-10).
As such, evaluating myocardial infarct segments alone is insufficient as a predictive strategy when seeking to gauge the outcomes of patients with ICM. Accordingly, this study was designed based on the hypothesis that the left ventricle myocardial infarct size can better predict improvements in cardiac function in patients with ICM following CABG. We present this article in accordance with the STROBE reporting checklist (available at https://qims.amegroups.com/article/view/10.21037/qims-23-159/rc).
Methods
Patients
This was a single-center, retrospective, observational cohort study of 169 consecutive patients with ICM who underwent LGE-CMR imaging in Beijing Anzhen Hospital between January 2017 and December 2021. To be eligible for inclusion, patients had to meet the following criteria: (I) resting transthoracic echocardiography demonstrating a left ventricular ejection fraction (LVEF) ≤40%, (II) diagnosed with coronary heart disease via cutaneous coronary angiography and treated with CABG, (III) LGE-CMR performed presurgery and echocardiography performed at 6 months postsurgery, and (IV) graft patency assessed at 6 months postsurgery. The individuals were not allowed to participate if they (I) had any history of acute myocardial infarction within the last 3 months, (II) underwent any other cardiac surgeries in combination with CABG, (III) exhibited preoperative atrial fibrillation or any other arrhythmias, (IV) were preoperatively diagnosed with malignant tumors, or (V) underwent emergency operative treatment for cardiogenic shock.
Of the 169 patients potentially eligible for this study, 50 underwent mitral valve surgery, 8 underwent drug therapy, 4 underwent tricuspid valve surgery, and 19 underwent simultaneous ventricular aneurysm resection. In total, 88 patients underwent CABG alone, of whom 2 died due to low cardiac output syndrome and 1 died within 6 months after surgery. Two patients exhibited graft occlusion at 6 months post-CABG, two were lost to follow-up, and three exhibited poor image quality. The remaining 78 patients were included in subsequent analyses because they fulfilled the study inclusion requirements (Figure 1).
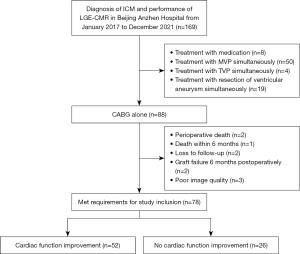
Study protocol
Both baseline clinical data and preoperative LGE-CMR findings were retrospectively collected for all patients in this study. Patient follow-up outcomes were obtained through telephone, WeChat, or outpatient services. Resting transthoracic echocardiography results at 6 months post-CABG were used to stratify patients into 2 groups based on whether or not they exhibited improvement in cardiac function. Improved cardiac function was defined as a minimum 5% increase in absolute LVEF (ΔLVEF ≥5%), with patients failing to meet this benchmark being considered to exhibit nonimproved cardiac function (7,8). Preoperative myocardial infarct size and myocardial infarct segments were compared between these 2 groups to gauge their relative performance as predictors of cardiac function improvement following CABG. The study was approved by the Ethics Committee of Beijing Anzhen Hospital (No. 2021104X). The study was conducted in accordance with the Declaration of Helsinki (as revised in 2013). The requirement for informed consent was waived due to the retrospective and observational nature of this study, which did not obtain specific information about patients.
CMR image acquisition
All patients underwent LGE-CMR imaging within 1 week before undergoing CABG. Imaging was performed with a Verio 3.0T superconducting magnetic resonance system (Siemens Healthineers, Erlangen, Germany) and a 32-channel dedicated heart-phased front ring, with electrocardiogram gating being applied. LGE-CMR imaging was conducted by injecting patients with gadolinium gluconate contrast (0.1 mmol/kg, i.v.) for about 10 min, after which a phase-sensitive inversion recovery (PSIR) sequence was conducted under the following settings: repeat time/echo time, 4.1 ms/1.56 ms; field of view, 350 mm2; matrix, 2.1 mm × 1.4 mm × 5.0 mm; turn angle, 35°; and acceleration factor, 2. The LV short-axis imaging layer was 8-mm thick, with an interval of 0 mm. The LV imaging layers for cardiac chambers were 5-mm thick without any separation.
LGE-CMR image postprocessing
Commercially available software, CVI 42 (Circle Cardiovascular Imaging Inc., Calgary, AB, Canada). was used to assess myocardial infarction and survival. The CMR data were independently evaluated by image analysts with 5 years of experience and above the title of associate senior in the department of radiology who were blinded to patient clinical data and group assignments.
The heart partitioning method proposed by the American Heart Association and the College of Cardiology was used to divide the left ventricle into 17 segments (12). Given that the apical segment was too thin for reliable evaluation, the remaining 16 segments were analyzed after omission of the apical segment.
Furthermore, after exclusion of the papillary muscles, the epicardium and endocardium muscles were evaluated at the short-axis level of the PSIR sequence, which was followed by the delineation of a normal myocardial area of interest that was free of LGE and away from the LGE area, with the LGE area, in turn being used to define the infarcted myocardium. The underlined LGE area was considered on the basis of the myocardial area with a gray threshold level 5 SD above the mean value for the healthy myocardial tissue. The subtraction of the end-diastolic pericardium volume followed by its multiplication by 1.05 g/cm3 enabled the software to compute the LV myocardial mass and LGE mass, with these values then being used to calculate myocardial infarct size (LGE mass/LV mass 100%). The left ventricle was analyzed in segments, with an LGE penetration degree of 0–50% being indicative of a viable segment and an LGE penetration degree of 51–100% being indicative of a myocardial infarct segment (13,14).
Surgical techniques
A medial sternotomy was performed for all patients. Moreover, the left internal mammary artery (LIMA) was considered for transplantation to the left anterior descending (LAD) branch, and additional coronary arteries were anastomosed to the great saphenous vein. Each patient who participated in the current study underwent anatomically complete revascularization (15). Transit time flow measurements were used to examine the quality of graft anastomosis, with nonfunctional anastomosis being defined by a pulse index >5 and/or a mean graft flow <10 mL/min. Reanastomosis was performed for nonfunctional grafts until satisfactory anastomosis had been achieved. After surgery, all patients underwent standard anti-heart failure drug therapy (16).
All patients received guideline-directed drug therapy (GDMT) that included antiplatelet agents, angiotensin-converting enzyme inhibitors (ACEIs), angiotensin type II receptor blockers (ARBs), or angiotensin receptor and neprilysin inhibitors, beta blockers, and mineral corticosteroid receptor antagonists (1).
Patient follow-up
All patients underwent routine follow-up for 6 months following surgical treatment to gauge major adverse cardiovascular and cerebrovascular events (MACCE) incidence, changes in cardiac function, and patient New York Heart Association (NYHA) grading. Cardiac function was considered improved if patients exhibited ΔLVEF ≥5% on postoperative resting transthoracic echocardiography at 6 months post-CABG (7,8). MACCEs included all-cause mortality, cerebral infarction, myocardial infarction, and hospital readmission due to heart failure. Graft patency was also analyzed via coronary computed tomography angiography (17). Patient data were collected from the online database maintained by our institution by trained staff blinded to the goals of this study using standardized data collection forms.
Statistical analysis
Normally distributed continuous data are presented as the mean ± SD and were compared with the Student’s t-test; meanwhile, they are presented as the median and interquartile range when nonnormally distributed and were compared with Mann-Whitney tests. Categorical data are expressed as frequency (rate) and were compared with chi-squared or Fisher exact tests. Area under the receiver operating characteristic (ROC) curve (AUC) values were calculated, and the maximum Youden index value (sensitivity + specificity – 1) was selected as a cutoff threshold for predicting whether or not patients would experience improved cardiac function. Independent predictors of improved cardiac function were selected using a binary logistic regression analysis approach. MACCE-free survival rates and all-cause mortality-free survival rates were compared between the 2 patient groups using Kaplan-Meier curves and the log-rank test. Pearson correlation was used to analyze the correlation between myocardial scar size and the degree of LVEF improvement. Additionally, Stata version16 (StataCorp, College Station, TX, USA) and SPSS version 23.0 (IBM Corp., Armonk, NY, USA) were used for all statistical analyses. P<0.05 was the significance threshold.
Results
Baseline patient characteristics
This study enrolled 78 patients with ICM who underwent CABG. At 6 months post-CABG, 52 patients (66.7%) exhibited improved cardiac function while 26 (33.3%) did not. The mean age of the overall patient cohort was 61.2±9.5 years (range, 39–84 years), and 79.5% (62/78) were male, with a larger proportion of males in the nonimproved cardiac function group (73.1% vs. 92.3%; P=0.047). No significant differences were observed between these 2 groups with respect to age, body mass index (BMI), body surface area (BSA), past medical history, or NYHA cardiac function classification (all P values >0.05) (Table 1).
Table 1
Variable | Improved (n=52) | Nonimproved (n=26) | P value |
---|---|---|---|
Age (years) | 61.8±10.1 | 60.0±8.2 | 0.428 |
Male | 38 (73.1) | 24 (92.3) | 0.047 |
BMI (kg/m2) | 25.3±2.8 | 25.0±2.5 | 0.558 |
BSA (m2) | 1.78±0.2 | 1.78±0.2 | 0.923 |
History | |||
Hypertension | 23 (44.2) | 12 (46.2) | 0.872 |
Diabetes | 19 (36.5) | 6 (23.1) | 0.230 |
Hyperlipemia | 21 (40.4) | 12 (46.2) | 0.627 |
Smoking | 21 (40.4) | 16 (61.5) | 0.078 |
Drinking | 14 (26.9) | 10 (38.5) | 0.298 |
Renal insufficiency | 2 (3.8) | 1 (3.8) | 1.000 |
Cerebral infarction | 6 (11.5) | 3 (11.5) | 1.000 |
COPD | 1 (1.9) | 1 (3.8) | 1.000 |
NYHA grading | 0.524 | ||
I | 3 (5.8) | 1 (3.8) | |
II | 22 (42.3) | 10 (38.5) | |
III | 18 (34.6) | 9 (34.6) | |
IV | 9 (17.3) | 6 (23.1) |
Data are presented as mean ± standard deviation or number (%). BMI, body mass index; BSA, body surface area; COPD, chronic obstructive pulmonary disease; NYHA, New York Heart Association.
There was no difference found in LVEF between the groups when preoperative imaging data from patients who underwent CABG and those who did not were compared (36.2%±4.5% vs. 35.4%±4.5%; P=0.500). Although left ventricular end-diastolic diameter (LVEDD) and left ventricular end-systolic diameter (LVESD) of patients in the nonimproved group were larger than those of the improved group, there was no statistical difference between the 2 groups in this regard (P>0.05). Coronary angiography failed to reveal any differences in Synergy Between Percutaneous Coronary Intervention With T axus and Cardiac Surgery (SYNTAX) scores or numbers of coronary lesions between the 2 groups (P>0.05). LGE-CMR imaging data revealed that compared to improved group, the group had significantly more myocardial infarct segments [improved group: median 1.0, interquartile range (IQR) 0–3; nonimproved group: median 4.0, IQR 3.0–6.0; P<0.001] and significantly greater myocardial infarct size (improved group: 22.4%±8.2%; nonimproved group: 34.7%±5.9%; P<0.001) (Table 2). Representative LGE-CMR images from patients that did and did not exhibit improvements in cardiac function at 6 months after CABG are respectively shown in Figures 2,3.
Table 2
Variable | Improved (n=52) | Nonimproved (n=26) | P value |
---|---|---|---|
Echocardiogram | |||
LVEF (%) | 36.2±4.5 | 35.4±4.5 | 0.500 |
LVEDD (mm) | 57.2±6.5 | 60.2±6.1 | 0.058 |
LVESD (mm) | 44.2±7.6 | 47.8±6.8 | 0.055 |
Ventricular septal thickness (mm) | 9.9±2.0 | 9.0±2.0 | 0.078 |
LVPWT (mm) | 8.5±1.9 | 8.5±1.2 | 0.958 |
Coronary angiogram | 0.320 | ||
One lesion | 1 (1.9) | 2 (7.7) | |
Two lesions | 7 (13.5) | 5 (19.2) | |
Three lesions | 44 (84.6) | 19 (73.1) | |
SYNTAX score | 41.5±7.9 | 42.0±6.9 | 0.772 |
LGE-CMR | |||
Myocardial infarct segments | 1.0 (0, 3.0) | 4.0 (3.0, 6.0) | <0.001 |
Myocardial infarct size (%) | 22.4±8.2 | 34.7±5.9 | <0.001 |
Data are presented as the mean ± standard deviation, median (interquartile range), or number (%). LVEF, left ventricular ejection fraction; LVEDD, left ventricular end diastolic diameter; LVESD, left ventricular end-systolic diameter; LVPWT, left ventricular posterior wall thickness.
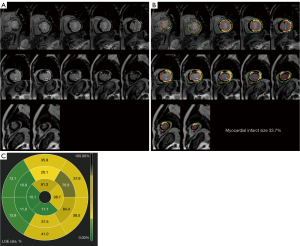
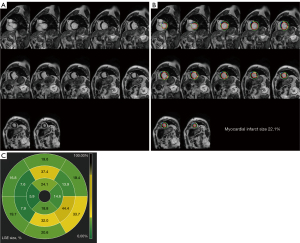
Surgery-related data
When operative data were compared between patients that did and did not experience improvements in cardiac function, no differences in extracorporeal circulation use, LIMA utilization rates, graft numbers, operative duration, or blood product usage were observed between these groups (P>0.05). A nonsignificant tendency toward longer intensive care unit admission, ventilator use, and postoperative hospitalization was evident in the nonimproved group relative to the improved group (P>0.05) (Table 3).
Table 3
Variable | Improved (n=52) | Nonimproved (n=26) | P value |
---|---|---|---|
Off-pump | 36 (69.2) | 21 (80.8) | 0.279 |
LIMA | 49 (94.2) | 24 (92.3) | 1.000 |
Number of bypass grafts | 3.4±0.7 | 3.5±0.8 | 0.914 |
Time of operation (h) | 4.2±0.8 | 4.3±0.9 | 0.547 |
Erythrocyte (U) | 0 (0, 4) | 0 (0, 2.5) | 0.807 |
Plasma (mL) | 0 (0, 0) | 0 (0, 0) | 0.559 |
Platelet (U) | 0 (0, 0) | 0 (0, 0) | 0.521 |
ICU stay (h) | 47.0 (23.5, 73.2) | 47.8 (26.4, 94.0) | 0.574 |
Ventilator use time (h) | 26.0 (19.6, 55.8) | 26.5 (22.3, 62.5) | 0.369 |
Postoperative hospital stay (d) | 7.0 (6.0, 9.8) | 9.0 (7.0, 12.0) | 0.135 |
Data are presented as the mean ± standard deviation, median (interquartile range), or number (%). LIMA, left internal mammary artery; ICU, intensive care unit.
LGE-CMR parameters predicted postoperative improvement in cardiac function
ROC analyses revealed that the number of myocardial infarct segments could predict cardiac function improvement (ΔLVEF ≥5%) at 6 months post-CABG with an AUC of 0.81 [95% confidence interval (CI): 0.71–0.91]. The AUC for myocardial infarct size for predicting improved cardiac function was 0.88 (95% CI: 0.80–0.95), and the optimal cutoff threshold value for myocardial infarct size was ≥26.4%, enabling the prediction of improved cardiac function with respective sensitivity and specificity values of 92.3% and 71.2%. Based on a comparison of these AUC values, myocardial infarct size was determined to be a more effective predictor of improvement in cardiac function following CABG as compared to myocardial infarct segments (P=0.041; Figure 4). Univariate analyses indicated that myocardial infarct size was associated with a lack of improvement in cardiac function following CABG. Following correction for gender, LVEDD, LVESD, and ventricular septal thickness via a binary logistics regression analysis, myocardial infarct size was identified as an independent predictor of nonimprovement in cardiac function [odds ratio (OR) =1.244, 95% CI: 1.114–1.389; P<0.001] (Table 4).
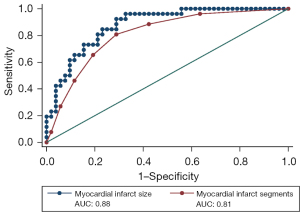
Table 4
Variables | Univariate analysis | Multivariate analysis | |||
---|---|---|---|---|---|
OR (95% CI) | P value | OR (95% CI) | P value | ||
Gender | 0.226 (0.047–1.084) | 0.063 | 0.162 (0.010–2.511) | 0.193 | |
LVEDD | 1.079 (0.996–1.168) | 0.062 | 1.127 (0.882–1.441) | 0.338 | |
LVESD | 1.070 (0.997–1.147) | 0.059 | 0.889 (0.712–1.110) | 0.299 | |
Ventricular septal thickness | 0.795 (0.615–1.026) | 0.078 | 0.842 (0.600–1.181) | 0.318 | |
Myocardial infarct size | 1.244 (1.128–1.372) | <0.001 | 1.244 (1.114–1.389) | <0.001 |
OR, odds ratio; CI, confidence interval; LVEDD, left ventricular end-diastolic diameter; LVESD, left ventricular end-systolic diameter.
Follow-up results
Echocardiography results revealed significantly better LVEF and LVESD in the improved group relative to the nonimproved group at follow-up (45.9%±7.3% vs. 36.7%±4.4%, P<0.001; 39.4±4.7 vs. 44.3±6.7 mm, P<0.001). NYHA grading for patients in the improved group was lower than that for patients in the nonimproved group at 6 months following CABG, which was consistent with the significant alleviation of heart failure symptoms (P=0.019). MACCEs occurred in 3 and 7 patients in the improved and nonimproved groups, respectively, over a median 1.6-year follow-up interval (range, 0.5–4.1 years). Among patients in the improved group, 1 died of myocardial infarction, 1 developed cerebral infarction, and 1 was readmitted to the hospital due to heart disease. All 7 cases of MACCEs in the nonimproved group died due to heart failure. Kaplan-Meier survival analyses revealed significantly lower MACCE and lower all-cause mortality incidence in the nonimproved group relative to the improved group (P<0.001) (Table 5, Figures 5,6).
Table 5
Variable | Improved (n=52) | Nonimproved (n=26) | P value |
---|---|---|---|
Echocardiogram | |||
LVEF (%) | 45.9±7.3 | 36.7±4.3 | <0.001 |
LVEDD (mm) | 55.1±5.6 | 57.2±7.7 | 0.173 |
LVESD (mm) | 39.4±4.7 | 44.3±6.7 | <0.001 |
Ventricular septal thickness (mm) | 10.1±1.6 | 9.5±1.8 | 0.202 |
LVPWT (mm) | 8.9±1.5 | 8.1±1.7 | 0.030 |
NYHA grading | 0.019 | ||
I | 11 (21.2) | 3 (11.5) | |
II | 32 (61.5) | 12 (46.2) | |
III | 7 (13.5) | 6 (23.1) | |
IV | 2 (3.8) | 5 (19.2) | |
MACCE | 3 (5.8) | 7 (26.9) | <0.001 |
All-cause mortality | 1 (1.9) | 7 (26.9) | <0.001 |
Data are presented as mean ± standard deviation or number (%). LVEF, left ventricular ejection fraction; LVEDD, left ventricular end diastolic diameter; LVESD, left ventricular end-systolic diameter; LVPWT, left ventricular posterior wall thickness; NYHA, New York Heart Association; MACCE, major adverse cardiovascular and cerebrovascular event.
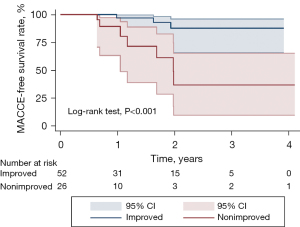
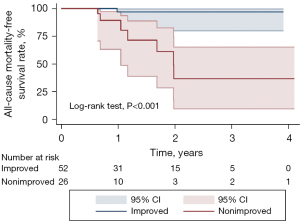
Additionally, we analyzed the correlation between myocardial scar size and the degree of improvement of LVEF (post-LVEF – pre-LVEF, ΔLVEF) and found that there was a negative correlation between myocardial scar size and the degree of improvement of LVEF (correlation coefficient =–0.487; P<0.001). The corresponding scatter plot is shown in Figure 7.
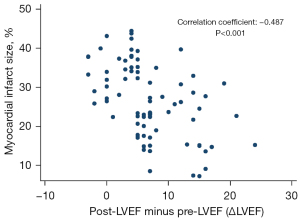
Discussion
There are several key findings to this study. First, cardiac function improved in 66.7% of the patients with ICM at 6 months post-CABG. Second, relative to myocardial infarct segments, preoperative myocardial infarct size was found to offer greater utility as a predictor of this post-CABG improvement in cardiac function. ROC curves were used to evaluate this predictive efficacy and ultimately demonstrated that a myocardial infarct size cutoff of 26.4% was the most effective threshold for predicting post-CABG cardiac function outcomes, yielding good sensitivity and specificity. Third, a lack of improvement in cardiac function in this patient cohort was found to be associated with a poor short- and medium-term prognosis, with patients who exhibited improved cardiac function also experiencing better outcomes. As such, these findings have the potential to guide efforts to better select patients likely to benefit from CABG treatment based on analyses of myocardial infarct size.
At present, CABG remains the primary treatment option for most patients with ICM (1). However, several prior reports have indicated varying rates of improvement in cardiac function following CABG (7-9,18-20). Nakae et al. further found that about 40% of patients with ICM did not exhibit any improvement in cardiac function after undergoing CABG, with an average follow-up interval of 64.5±45.5 months, with this lack of postoperative functional improvement being independently associated with higher postoperative all-cause mortality rates (4). In the present analysis, 66.7% of patients with ICM exhibited improved cardiac function at 6 months post-CABG whereas 33.3% did not, which is roughly in line with these previous results. In our study, the patient group that failed to exhibit any improvement in cardiac function also had a higher MACCE incidence as compared to the group that exhibited improved cardiac function.
Measurements of an infarcted myocardium have repeatedly been shown to outperform those of a viable myocardium in predicting improvements in cardiac function (5,21). Accordingly, we analyzed myocardial infarct size as a potential predictor of post-CABG cardiac functional outcome. The myocardium is composed of nonregenerating cells such that once myocardial fibrosis occurs, infarcted myocardial tissue cannot revert to viable tissue even following the restoration of blood flow. The infarcted myocardium can also impact the movement of the surrounding myocardial tissue, potentially counteracting appropriate contractile movements of the viable myocardium and thereby interfering with the potential for any improvement in myocardial function. These factors highlight the potential advantages of focusing on myocardial infarct size given that it may ultimately have a greater impact on cardiac functional outcomes.
Researchers have frequently used myocardial infarct size to gauge the extent of myocardial injury, with larger infarct size generally being indicative of more severe injury and lower likelihood of cardiac functional improvement. In prior studies (7-9), myocardial infarct segments were reported to offer value as a tool for predicting improvement in cardiac function in patients with ICM following CABG, which is in line with our results. However, more detailed comparisons of the predictive utility of myocardial infarct segments and infarct size revealed that the latter was a more reliable predictor. This may be attributable to the fact that while the degree of LGE penetration of the myocardial wall is inversely correlated with myocardial segmental function improvements following revascularization, roughly one-third of myocardial infarct segments exhibiting LGE >50% still have the potential to exhibit improvement when revascularization is complete (10). Myocardial infarct size may thus be a more accurate predictor of cardiac outcomes in patients with ICM as compared to myocardial infarct segments.
Evidence from research supports the ability of myocardial infarct size to predict poor prognosis in patients with ICM (12,21-24). Infarct size is also independently predictive of the risk of cardiogenic death, and patients with a similar myocardial infarct size ultimately encounter a similar mortality risk irrespective of whether they undergo revascularization therapy or pharmacological treatment alone (25,26). In these patients, drug therapy may be the most effective therapeutic option given that revascularization did not benefit the survival rates in patients with infarcted myocardial tissue. Yang et al. previously reported that myocardial infarct size was the most significant predictor of perioperative and mid- and long-term adverse cardiovascular event incidence following CABG (13). In line with the present results, patients that did not exhibit improved cardiac function tended to exhibit a larger myocardial infarct size, and a lack of improved cardiac function was related to a worse prognosis in both the short- and medium-term.
In conclusion, the present analysis was conducted to determine whether myocardial infarct size, as measured by LGE-CMR, could be used to predict improvement in cardiac function following CABG in patients with ICM. Given that an absence of any functional improvement has important negative implications for patient prognostic outcomes, these findings have the potential to guide the care of patients who are unlikely to benefit from CABG. Alternate therapeutic options for these patients may include continued medication, electrophysiological device placement [such as implantable cardioversion defibrillator (ICD) or cardiac resynchronization therapy (CRT)], surgical higher-order alternative therapy for advanced heart failure (such as left ventricular assist device or heart transplantation), or cell therapy (1,27).
Limitations
The sample size for this study was relatively small, and LGE-CMR imaging could not be performed in patients who had received CRT or ICD therapy or those with end-stage kidney disease or on hemodialysis. These patients were excluded, which might have introduced a degree of selection bias. Moreover, even small adjustments to endocardial and epicardial profiles can contribute to alterations in myocardial infarct size, and the CMR image analyst might have introduced subjective error when attempting to delineate these profiles. Finally, these patients did not undergo postoperative LGE-CMR imaging, and further research will be necessary to examine how these findings relate to patient outcomes.
Conclusions
Myocardial infarct size can be measured to reliably predict improvements in cardiac function in patients with ICM following CABG. These results can guide clinicians in their efforts to identify those patients most likely to achieve positive outcomes following CABG.
Acknowledgments
Funding: None.
Footnote
Reporting Checklist: The authors have completed the STROBE reporting checklist. Available at https://qims.amegroups.com/article/view/10.21037/qims-23-159/rc
Conflicts of Interest: All authors have completed the ICMJE uniform disclosure form (available at https://qims.amegroups.com/article/view/10.21037/qims-23-159/coif). Jumatay Biekan has been an employee of Circle Cardiovascular Imaging throughout his involvement in the study. The other authors have no conflicts of interest to declare.
Ethical Statement: The authors are accountable for all aspects of the work in ensuring that questions related to the accuracy or integrity of any part of the work are appropriately investigated and resolved. The study was approved by the Ethics Committee of Beijing Anzhen Hospital (No. 2021104X) and conducted in accordance with the Declaration of Helsinki (as revised in 2013). The requirement for informed consent was waived due to the retrospective and observational study design that did not require specific information about patients.
Open Access Statement: This is an Open Access article distributed in accordance with the Creative Commons Attribution-NonCommercial-NoDerivs 4.0 International License (CC BY-NC-ND 4.0), which permits the non-commercial replication and distribution of the article with the strict proviso that no changes or edits are made and the original work is properly cited (including links to both the formal publication through the relevant DOI and the license). See: https://creativecommons.org/licenses/by-nc-nd/4.0/.
References
- Bakaeen FG, Gaudino M, Whitman G, Doenst T, Ruel M, Taggart DP, Stulak JM, Benedetto U, Anyanwu A, Chikwe J, Bozkurt B, Puskas JD, Silvestry SC, Velazquez E, Slaughter MS, McCarthy PM, Soltesz EG, Moon MRAmerican Association for Thoracic Surgery Cardiac Clinical Practice Standards Committee. Invited Experts. 2021: The American Association for Thoracic Surgery Expert Consensus Document: Coronary artery bypass grafting in patients with ischemic cardiomyopathy and heart failure. J Thorac Cardiovasc Surg 2021;162:829-850.e1. [Crossref] [PubMed]
- Velazquez EJ, Lee KL, Jones RH, Al-Khalidi HR, Hill JA, Panza JA, Michler RE, Bonow RO, Doenst T, Petrie MC, Oh JK, She L, Moore VL, Desvigne-Nickens P, Sopko G, Rouleau JL. STICHES Investigators. Coronary-Artery Bypass Surgery in Patients with Ischemic Cardiomyopathy. N Engl J Med 2016;374:1511-20. [Crossref] [PubMed]
- Rizzello V, Poldermans D, Biagini E, Schinkel AF, Boersma E, Boccanelli A, Marwick T, Roelandt JR, Bax JJ. Prognosis of patients with ischaemic cardiomyopathy after coronary revascularisation: relation to viability and improvement in left ventricular ejection fraction. Heart 2009;95:1273-7. [Crossref] [PubMed]
- Nakae M, Kainuma S, Toda K, Miyagawa S, Yoshikawa Y, Hata H, et al. Incidence, determinants and clinical impact of left ventricular function recovery after surgical treatments for ischaemic cardiomyopathy. Eur J Cardiothorac Surg 2021;60:689-96. [Crossref] [PubMed]
- Dhore-Patil AS, Aneja A. Role of Cardiovascular Magnetic Resonance in Ischemic Cardiomyopathy. Heart Fail Clin 2021;17:41-56. [Crossref] [PubMed]
- Krittayaphong R, Zhang S, Tanapibunpon P, Kaolawanich Y, Nakyen S. Dark-blood late gadolinium-enhancement cardiac magnetic resonance imaging for myocardial scar detection based on simplified timing scheme: single-center experience in patients with suspected coronary artery disease. Quant Imaging Med Surg 2022;12:1037-50. [Crossref] [PubMed]
- Yang T, Lu MJ, Sun HS, Tang Y, Pan SW, Zhao SH. Myocardial scar identified by magnetic resonance imaging can predict left ventricular functional improvement after coronary artery bypass grafting. PLoS One 2013;8:e81991. [Crossref] [PubMed]
- Hwang HY, Yeom SY, Choi JW, Oh SJ, Park EA, Lee W, Kim KB. Cardiac Magnetic Resonance Predictor of Ventricular Function after Surgical Coronary Revascularization. J Korean Med Sci 2017;32:2009-15. [Crossref] [PubMed]
- Pegg TJ, Selvanayagam JB, Jennifer J, Francis JM, Karamitsos TD, Dall'Armellina E, Smith KL, Taggart DP, Neubauer S. Prediction of global left ventricular functional recovery in patients with heart failure undergoing surgical revascularisation, based on late gadolinium enhancement cardiovascular magnetic resonance. J Cardiovasc Magn Reson 2010;12:56. [Crossref] [PubMed]
- Hwang HY, Yeom SY, Park EA, Lee W, Jang MJ, Kim KB. Serial cardiac magnetic resonance imaging after surgical coronary revascularization for left ventricular dysfunction. J Thorac Cardiovasc Surg 2020;159:1798-805. [Crossref] [PubMed]
- Fu W, Zhao Y, Zhang K, Dai Q, Biekan J, Zheng J, Dong R, Mu J. Retrospective, observational analysis of cardiac function associated with global preoperative myocardial scar in patients with ischemic cardiomyopathy after coronary artery bypass grafting. J Thorac Dis 2022;14:4319-28. [Crossref] [PubMed]
- Cerqueira MD, Weissman NJ, Dilsizian V, Jacobs AK, Kaul S, Laskey WK, Pennell DJ, Rumberger JA, Ryan T, Verani MS. Standardized myocardial segmentation and nomenclature for tomographic imaging of the heart. A statement for healthcare professionals from the Cardiac Imaging Committee of the Council on Clinical Cardiology of the American Heart Association. Circulation 2002;105:539-42. [Crossref] [PubMed]
- Yang T, Lu M, Ouyang W, Li B, Yang Y, Zhao S, Sun H. Prognostic value of myocardial scar by magnetic resonance imaging in patients undergoing coronary artery bypass graft. Int J Cardiol 2021;326:49-54.
- Di Bella G, Pizzino F, Aquaro GD, Bracco A, Manganaro R, Pasanisi E, Petersen C, Zito C, Chubuchny V, Emdin M, Khandheria BK, Carerj S, Pingitore A. CMR predictors of secondary moderate to severe mitral regurgitation and its additive prognostic role in previous myocardial infarction. J Cardiol 2022;79:90-7. [Crossref] [PubMed]
- Neumann FJ, Sousa-Uva M, Ahlsson A, Alfonso F, Banning AP, Benedetto U, et al. 2018 ESC/EACTS Guidelines on myocardial revascularization. Eur Heart J 2019;40:87-165. [Crossref] [PubMed]
- Heidenreich PA, Bozkurt B, Aguilar D, Allen LA, Byun JJ, Colvin MM, et al. 2022 AHA/ACC/HFSA Guideline for the Management of Heart Failure: A Report of the American College of Cardiology/American Heart Association Joint Committee on Clinical Practice Guidelines. J Am Coll Cardiol 2022;79:e263-e421. [Crossref] [PubMed]
- Fitzgibbon GM, Kafka HP, Leach AJ, Keon WJ, Hooper GD, Burton JR. Coronary bypass graft fate and patient outcome: angiographic follow-up of 5,065 grafts related to survival and reoperation in 1,388 patients during 25 years. J Am Coll Cardiol 1996;28:616-26. [Crossref] [PubMed]
- Schinkel AF, Poldermans D, Rizzello V, Vanoverschelde JL, Elhendy A, Boersma E, Roelandt JR, Bax JJ. Why do patients with ischemic cardiomyopathy and a substantial amount of viable myocardium not always recover in function after revascularization? J Thorac Cardiovasc Surg 2004;127:385-90. [Crossref] [PubMed]
- Baer FM, Theissen P, Crnac J, Schmidt M, Deutsch HJ, Sechtem U, Schicha H, Erdmann E. Head to head comparison of dobutamine-transoesophageal echocardiography and dobutamine-magnetic resonance imaging for the prediction of left ventricular functional recovery in patients with chronic coronary artery disease. Eur Heart J 2000;21:981-91. [Crossref] [PubMed]
- Bax JJ, Poldermans D, Elhendy A, Cornel JH, Boersma E, Rambaldi R, Roelandt JR, Fioretti PM. Improvement of left ventricular ejection fraction, heart failure symptoms and prognosis after revascularization in patients with chronic coronary artery disease and viable myocardium detected by dobutamine stress echocardiography. J Am Coll Cardiol 1999;34:163-9. [Crossref] [PubMed]
- Doukas D, Porcaro K, Marot J, Burke L, Joyce C, Weaver F, Nguyen J, Cao JJ, Mathew V, Heroux A, Syed MA. Clinical characteristics and outcomes of patients with severe left ventricular dysfunction undergoing cardiac MRI viability assessment prior to revascularization. Int J Cardiovasc Imaging 2021;37:675-84. [Crossref] [PubMed]
- Chery G, Kamp N, Kosinski AS, Schmidler GS, Lopes RD, Patel M, Al-Khatib SM. Prognostic value of myocardial fibrosis on cardiac magnetic resonance imaging in patients with ischemic cardiomyopathy: A systematic review. Am Heart J 2020;229:52-60. [Crossref] [PubMed]
- Kancharla K, Weissman G, Elagha AA, Kancherla K, Samineni S, Hill PC, Boyce S, Fuisz AR. Scar quantification by cardiovascular magnetic resonance as an independent predictor of long-term survival in patients with ischemic heart failure treated by coronary artery bypass graft surgery. J Cardiovasc Magn Reson 2016;18:45. [Crossref] [PubMed]
- Dang Y, Hou Y. The prognostic value of late gadolinium enhancement in heart diseases: an umbrella review of meta-analyses of observational studies. Eur Radiol 2021;31:4528-37. [Crossref] [PubMed]
- Kwon DH, Obuchowski NA, Marwick TH, Menon V, Griffin B, Flamm SD, Hachamovitch R. Jeopardized Myocardium Defined by Late Gadolinium Enhancement Magnetic Resonance Imaging Predicts Survival in Patients With Ischemic Cardiomyopathy: Impact of Revascularization. J Am Heart Assoc 2018;7:e009394. [Crossref] [PubMed]
- Gerber BL, Rousseau MF, Ahn SA, le Polain de Waroux JB, Pouleur AC, Phlips T, Vancraeynest D, Pasquet A, Vanoverschelde JL. Prognostic value of myocardial viability by delayed-enhanced magnetic resonance in patients with coronary artery disease and low ejection fraction: impact of revascularization therapy. J Am Coll Cardiol 2012;59:825-35. [Crossref] [PubMed]
- Bolli R, Solankhi M, Tang XL, Kahlon A. Cell therapy in patients with heart failure: a comprehensive review and emerging concepts. Cardiovasc Res 2022;118:951-76. [Crossref] [PubMed]