Correlation of brain iron deposition and freezing of gait in Parkinson’s disease: a cross-sectional study
Introduction
Parkinson’s disease (PD) is a rapidly progressive neurodegenerative disease that causes a range of clinical motor and nonmotor symptoms (1). Motor dysfunction is a common symptom of PD. Freezing of gait (FOG) is a disabling paroxysmal motor phenomenon in PD that affects gait, movement, and speech and can occur in up to 80% of patients with PD (2). The underlying pathological basis of FOG is not fully understood, but it is thought that the loss of dopamine cells in the substantia nigra striatum leads to a gradient in striatal dopamine consumption, creating an imbalance between direct (promoting) and indirect (inhibiting) pathways through the basal ganglia and thus precipitating bradykinesia (3).
In PD, clinical agents, such as dopaminergic drugs, are often used to improve symptoms of FOG. FOG can be divided into 3 categories based on its response to dopaminergic drugs: dopamine-responsive FOG, dopamine-induced FOG, and dopamine-resistant FOG (4). Patients with FOG in the first category have symptoms that appear mainly in the “OFF” state, respond well to dopaminergic therapy, and show significant improvement in movement disorder symptoms with medication (5). The patients in our study belonged to this category. The OFF state is defined as 12 hours after the last PD drug treatment, and the “ON” state is defined as use of super-ON doses of levodopa (125% of the morning dose) on the same day (6).
Iron is an essential trace element that participates in various biological processes in the human body and plays an important role in the nervous system (7). Iron deposition in the reactive form may contribute to the emergence of neurodegenerative disease, including PD (8). The pathogenesis of PD is related to iron metabolism in the brain and the iron binding ability of central nerve melanin (9). Many studies have proven that iron deposition is an important marker in the development of PD (8,10), and many hypotheses have been proposed concerning this phenomenon. Increased iron intake, altered iron transporters, and increased the blood-brain barrier (BBB) permeability have all been suggested as potential factors that contribute to increased iron deposition in PD (11). One study found that areas involved in motor and cognitive functions and visual processing show the highest iron increase with aging (12). One study linked iron deposition in the brains of people with PD to movement disorders (13). Nonetheless, despite many hypotheses being advanced, the specific mechanism of iron deposition in the development of PD movement disorder remains unclear and under constant study.
Magnetic resonance imaging (MRI) is a reliable, noninvasive method for detecting brain iron deposition in patients with PD and does not harm the human body (14). A number of magnetic resonance (MR) methods have been used to measure the iron levels in the brain, including susceptibility-weighted imaging (SWI); R2, R2*, and R2' phase; and quantitative susceptibility mapping (QSM) (15). Among these, QSM is a widely used nuclear magnetic technology. Compared with the other techniques, it has the advantage of being able to quantitatively detect the information of the potential susceptibility distribution of biological objects. Magnetic susceptibility is a physical quantity that describes the interaction between a moving charge in an atom or molecule of a substance and an applied magnetic field (16). Iron has a high magnetic susceptibility, and previous studies have shown that the magnetic susceptibility measured by QSM is linearly related to the concentration of iron in the deep brain, and thus QSM can be used to quantitatively detect brain iron content (17,18). As a reliable clinical assessment technique, QSM has become a powerful tool for monitoring brain iron content, especially in neurodegenerative diseases (15,19).
Recently, several studies on the correlation between PD and iron deposition in the brain have been conducted. There is a consensus that iron levels are directly related to the onset of PD (20,21). A previous study reported that iron deposition in the substantia nigra was positively correlated with the degree of FOG in patients with PD (10) and the iron deposition in the substantia nigra increased with the deterioration of motor function, which is consistent with our own speculation (22). It has also been demonstrated that the pattern of iron deposition is different for different dyskinesia subtypes (23). However, related studies have only conducted differential analyses of iron deposition in patients with PD with or without FOG (10), but the correlation between specific gait data and iron deposition in the brain has not been examined.
Therefore, this study focused on assessing the basal ganglia pattern of iron deposition in patients with PD using region of interest (ROI)-based QSM analysis. Clinical assessments, including PD motor examination and gait measures during both the ON and OFF states in PD participants were further conducted, and the relationship between the ON and OFF states in dopamine-responsive gait impairment and their correlation with MR changes in PD were determined. We present this article in accordance with the STROBE reporting checklist (available at https://qims.amegroups.com/article/view/10.21037/qims-23-267/rc).
Methods
Ethical statement
This study was conducted in accordance with the Declaration of Helsinki (as revised in 2013) and was approved by the ethics committee of Beijing Friendship Hospital, Capital Medical University (No. 2023-P2-110-02). All participants involved in this study provided an informed consent form.
Patient enrollment
A cross-sectional study design was employed, in which patients with PD receiving optimal treatment with dopaminergic medications were recruited from the Movement Disorders Program in the hospital. The exclusion criteria were atypical or secondary parkinsonism, confounding medical or psychiatric condition(s), any condition that prevented the ability to give informed consent, and other neurological diseases that could cause motor deficits. Community volunteers and patients’ companions without neurological disorders were recruited as healthy controls (HCs). Participants with poor image scan quality were not included in the study. We referred to similar literature for sample size determination and performed patient nerfing (24); ultimately, 24 patients and 36 HCs were included in this study. The information for demographic and clinical status of the participants are presented in Table 1. Movement disorder neurologists confirmed the diagnosis of idiopathic PD according to the UK Parkinson’s Disease Society Brain Bank criteria and evaluated all patients with PD. Patients with gait impairment were diagnosed according to their history and the Movement Disorder Society Unified-Parkinson Disease Rating Scale (MDS-UPDRS) assessment (a score ≥1 on items 2.12 or 2.13 on MDS-UPDRS-II or a score of ≥1 on items 3.10 or 3.11 on MDS-UPDRS-III) (25). Patients with PD were evaluated with the following tests to evaluate disease status and gait impairment: MDS-UPDRS, Berg Balance Scale (BBS), New Freezing of Gait Questionnaire (nFOGQ), and the Timed Up and Go (TUG). The MDS-UPDRS provides a comprehensive and detailed rating of disease severity; the BBS is a composite scale that assesses the body’s balance function, with a higher the score indicating better balance; and TUG is scored for gait and the risk of falling during the testing process. These tests have been proven to be effective tools in PD assessment (26). All clinical evaluations were carried out separately in both the OFF state and ON state. For all PD and control participants, objective/computerized gait assessments quantifying velocity, stride length, stride time and cadence at a self-selected pace (SSP) and fast pace (FP) were assessed on a 20-foot-long computerized Zeno Walkway system (ProtoKinetics, Havertown, PA, USA). All participants were evaluated with the Montreal Cognitive Assessment (MoCA), and all were screened for intelligent functioning using the Mini-Mental State Examination (MMSE). The Modified Apathy Evaluation Scale (MAES) was used to assess the severity of apathy in patients, the Beck Anxiety Inventory (BAI) was used to measure an individual’s level of anxiety, and the Beck Depression Inventory (BDI) was used to measure the degree of depressive symptoms in an individual. All scales were rated by the same experienced neurologist.
Table 1
Measurements | HC | PD (clinical parameters) | |
---|---|---|---|
ON state | OFF state | ||
Total | 36 | 24 | |
Age (years) | 64.5 (55.5–70.0) | 68.0 (63.5–71.8) | |
Sex (male) | 15 (41.7) | 7 (29.2) | |
Education (>9 years) | 16 (44.4) | 12 (50.0) | |
Duration of disease (months) | n/a | 58.0 (27.3–81.8) | |
MMSE | 28.0 (25.3–29.0) | 28.0 (28.0–29.0) | |
MAES | 11.5 (7.3–17.0) | 7.5 (5.0–14.8) | |
BAI | 24.0 (22.0–26.0) | 26.0 (24.3–30.5)** | |
BDI | 3.0 (1.0–4.8) | 8.0 (4.0–10.0)** | |
LEDD (mg) | n/a | 550.0 (456.3–718.8) | |
nFOGQ | n/a | 10.0 (41.7) | |
PDQ-39 | n/a | 23.0 (9.5–37.3) | |
MDS-UPDRS-III | n/a | 16.0 (13.3–22.3) | 29.0 (19.3–39.3)## |
MDS-UPDRS Total | n/a | 39.5 (25.3–48.8) | 54.0 (32.5–66.8)# |
TUG | 8.2 (7.3–8.8) | 10.1 (8.5–12.3)** | 11.6 (9.8–15.0)** |
BBS | 56.0 (54.0–56.0) | 52.0 (44.5–54.0)** | 49.5 (37.0–52.8)** |
Velocity SSP (cm/s) | 115.6 (109.1–127.0) | 102.2 (77.3–113.4)** | 92.7 (75.0–110.5)** |
Cadence SSP (step/min) | 110.5 (105.9–118.2) | 114.3 (105.5–119.1) | 112.9 (102.6–120.5) |
Stride time SSP (s) | 1.1 (1.0–1.1) | 1.0 (1.0–1.1) | 1.1 (1.0–1.2) |
Stride length SSP (cm) | 128.9 (115.2–136.4) | 105.4 (87.6–120.7)** | 101.4 (75.6–110.8)** |
Velocity FP (cm/s) | 147.0 (136.8–165.6) | 124.5 (94.8–132.8)** | 110.1 (96.4–134.3)** |
Cadence FP (step/min) | 126.6 (116.9–135.7) | 121.7 (114.0–130.0) | 125.8 (111.7–132.0) |
Stride time FP (s) | 0.9 (0.9–1.0) | 1.0 (0.9–1.1) | 1.1 (1.0–1.3) |
Stride length FP (cm) | 139.0 (128.0–151.7) | 120.0 (94.2–132.9)** | 113.3 (89.5–122.4)** |
Data are presented as n (%) or median (interquartile range). For control, there is no ON or OFF state. The same set of clinical data from the control group were used to analyze the PD group. **, P<0.01, compared with control group; #, P<0.05, compared with ON state; ##, P<0.01, compared with ON state. HC, heathy control; PD, Parkinson’s disease; MMSE, Mini-Mental State Examination; MAES, Modified Apathy Evaluation Scale; BAI, Back Anxiety Inventory; BDI, Back Depression Inventory; LEDD, levodopa equivalent daily dose; n/a, not available; nFOGQ, New Freezing of Gait Questionnaire; PDQ-39, quality of life questionnaire for patients with Parkinson’s disease; MDS-UPDRS, International Parkinson and Movement Disorder Society-Unified Parkinson’s Disease Rating Scale; MDS-UPDRS-III, MDS-UPDRS motor score; TUG, Timed Up and Go; BBS, Berg Balance Scale; SSP, self-selected pace; FP, fast pace.
MRI acquisition
All participants underwent image acquisition on a 3.0-T MAGNETON Prisma MRI system (Siemens Healthineers, Erlangen, Germany). The high-resolution T1 structure sequence and QSM sequence were obtained by using the fast gradient echo sequence prepared via three-dimensional (3D) magnetization. The specific parameters were as follows: 3D T1-weighted (T1W) magnetization-prepared rapid gradient echo (MPRAGE) sequence for anatomic structure [repetition time (TR) =7.3 ms, time to echo (TE) =2.4 ms, inversion time (TI) =900 ms, flip angle =9°, and isotropic voxel size =1 mm3] and a multiecho gradient echo (ME-GRE) sequence for QSM (TR =50 ms, first TE =6.8 ms, TE interval =4.1 ms, number of echoes =10, flip angle =15°, and voxel size =1.5×1.5×2.0 mm3). In addition, we scanned fluid-attenuated inversion recovery (FLAIR) sequences to detect abnormalities in the brain (TR =8,500 ms, TE =87 ms, TI =2,440, flip angle =150°, and voxel size =0.4×0.4×6.0 mm3).
QSM preprocessing and quantitative analysis
Brain QSM mapping was calculated from complex ME-GRE image data using morphological-enabled dipole inversion and automatic uniform cerebrospinal fluid (CSF) zero-reference algorithms [morphology enabled dipole inversion (MEDI ) +0; 0.001 for L1 regularization and 0.01 for L2 regularization] (27). We used a nonlinear fit to the multiecho data to estimate the total field. First, the entire field was spatially unwrapped using a quality-guided region-growing algorithm (28). Then, the projection to dipole field (PDF) algorithm was used to remove the background field, calculate the local field, and invert the local field to obtain the final magnetic susceptibility map. We used structural priors (edges) derived from amplitude images and regularization terms to force a uniform sensitivity distribution of lateral intraventricular CSF in numerical inversion to improve QSM quality and provide CSF as an automatic sensitivity reference. The CSF mask was determined by thresholding the R2* mapping calculated from the GRE amplitude data and applying voxel connectivity (27). Traditional images (T1W, FLAIR) were processed using an automated FMRIB Software Library (FSL) pipeline bias field correction using FMRIB’s Automated Segmentation Tool (FAST) (29) and by linear coregistration to the ME-GRE magnitude image (which was in the same space as QSM) using FMRIB’s Linear Registration Tool (FLIRT) with 6 degrees of freedom (30). For ROI analysis, FMRIB’s Integrated Registration Segmentation Tool (FIRST) was used to segment selected subcortical structures on T1W images, and the resulting segmentation mask was linearly confocal to QSM. These masks were then visually inspected by an experienced neuroradiologist and, if necessary (for example, to remove veins or cerebral microbleeds with high positive susceptibility values), manually edited on QSM images using ITK-SNAP v. 3.8 software [developed by Paul A. Yushkevich and colleagues at the Penn Image Computing and Science Laboratory (PICSL), University of Pennsylvania]. The mean susceptibility value within each ROI was recorded (Figure 1).
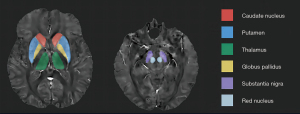
Statistical analysis
We analyzed the data using SPSS 20.0 software (IBM Corp., Armonk, NY, USA). For demographic data and clinical scales, categorical variables are expressed as percentages, and continuous variables are expressed as medians with interquartile ranges. The Shapiro-Wilk test was used for normality testing. The independent samples t-test was used for continuous variables conforming to a normal distribution, the rank-sum test was used for nonnormal distribution, and the chi-squared test was used for categorical variables. For the QSM data of patients with PD, we performed a within-group difference analysis on the left and right sides of each nucleus in the PD group and the HC group, and the results showed no significant difference. Therefore, we used the average of the 2-sided QSM values in the subsequent analysis. The difference test of the QSM value on each ROI between the PD group and the HC group was performed using the difference test of 2 independent samples. To remove the effect of disease stage, we performed a partial correlation analysis of the relationship between the QSM values and the scales, using duration of illness as a confounding factor. The correlation between the QSM value in the brain of patients with PD and gait was analyzed with Pearson correlation (if the data conformed to a normal distribution) or Spearman correlation analysis (if the data did not conform to the normal distribution). Due to the validation of multiple hypotheses, the results were corrected with cluster-level family-wise error (FWE) correction.
Results
Demographics and behavioral measures of the study participants
This study included 24 patients and 36 HCs. The demographic and clinical data are shown in Table 1. Normality tests showed that not all clinical and objective gait measurements were consistent with the normality assumption (Table 1). There was no significant difference between the 2 groups in terms of age (P=0.07), sex (P=0.33), or MMSE (P=0.43). The BAI and BDI scores of the PD group were significantly higher than those of the control group (both P values <0.01) (Table 1). Compared with the HC group, the PD group had significantly lower BBS scores and higher TUG values (both P values <0.01) (Table 1). The velocity and stride lengths of the HC group were higher than those of the PD group (both P values <0.01). There was no significant difference in cadence or stride time between the PD group and the HC group. In the PD group, the MDS-UPDRS-III scores and MDS-UPDRS total scores were higher in the ON state than in the OFF state (both P values <0.01) (Table 1).
Susceptibility value analysis across ROIs
The susceptibility value of the bilateral substantia nigra of the PD group was significantly higher than that of the HC group (FWE-corrected P<0.01) (Figure 2). In the PD group, the iron deposition in the substantia nigra of patients with FOG was significantly higher than that of patients without FOG (P=0.04) (Figure 3). There were no significant differences in brain iron in the red nucleus, thalamus, putamen, caudate nucleus, or globus pallidus between the PD group and the HC group.
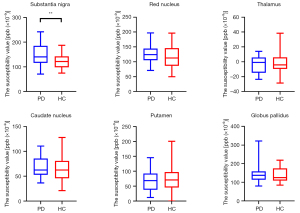
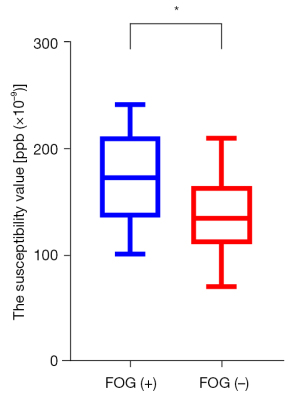
Correlation analysis
In the PD group, the susceptibility value of the substantia nigra was positively correlated with the nFOGQ (P=0.03; r=0.45) (Figure 4). The susceptibility values of the substantia nigra and gait data were not found to be significantly different (Figure 5).
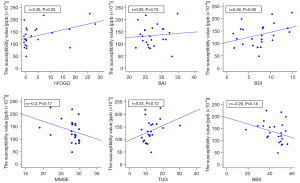
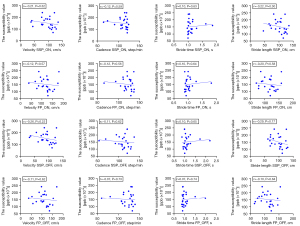
Discussion
We used QSM to identify brain tissue iron changes related to FOG in PD. For the first time, we demonstrated that tissue changes within the bilateral substantia nigra were related to FOG in PD and that brain iron increases in the bilateral substantia nigra were related to predictors of gait disorder outcome. The BAI score, BDI score, BBS score, TUG value, velocity, and the stride length were significantly different between the PD group and the HC group. No significant differences were found in the clinical scales of gait data for the ON state and OFF state in the PD group.
Differences in QSM values of the PD group and the HC group in each ROI
In this study, we used quantitative susceptibility imaging to analyze the content of iron in the brains of patients with PD. We found that the iron deposition in the bilateral substantia nigra of patients with PD was higher than that of the HC group. This finding is consistent with the findings of a previous study (31). Ghassaban et al. found that the amount of iron deposited in the substantia nigra was the most sensitive region for distinguishing healthy individuals from patients with PD (32). In other brain regions in people with PD, such as the red nucleus, thalamus, and globus pallidus, QSM can also show a higher susceptibility (33). However, in our experiment, there was no statistically significant difference in iron deposition in the red nucleus, thalamus, putamen, caudate nucleus, or globus pallidus between patients with PD and the control group. A recent review found that most QSM-MRI studies published since 2000 have reported significant increases in brain iron only in the substantia nigra (34). Other brain regions, such as the red nucleus, the globus pallidus, the thalamus, the putamen, and the caudate nucleus, have been shown, only in a small number of few studies, to a show significant difference in brain iron deposition between patients with PD and healthy individuals. One study reported that changes in iron deposition in gray-matter nuclei are related to the progression of PD and can be used to predict the staging of PD (35). Our findings might also have been influenced by the stage of PD in which the patients were enrolled.
Differences in QSM values of the patients with FOG and the patients without FOG in the substantia nigra
FOG, a common movement disorder in patients with PD, is often observed in the late stage of PD. FOG is not only associated with motor impairment in patients with PD but is also more commonly associated with the nonmotor symptoms of PD, such as cognitive impairment, anxiety, and sleep disturbances (36). When it appears, it can cause varying degrees of damage to the quality of life of patients with PD (37). In our study, we found that the iron content in the substantia nigra of the FOG group was significantly higher than that of the non-FOG group. This result is consistent with several previous studies (10,38), as the iron content reflects the structural changes of the substantia nigra. The result confirms that the substantia nigra plays an important role in the generation of FOG although the specific mechanism of FOG in the substantia nigra needs further study.
Correlation between the QSM values of the substantia nigra and gait measurements in patients with FOG
In this study, we applied a computerized walking path system to collect the gait information of patients and evaluated the motion state of patients with PD using a clinical scale. The correlation between the obtained data and the iron deposition in brains of the patients was studied. The results showed that the iron deposition in the substantia nigra of patients with PD was positively correlated with the nFOGQ, which indicated that the iron content in the brain of patients with PD could reflect the FOG of patients to a certain extent. Iron deposition in the nigra and striatum subregions is associated with dopaminergic deficits and neurophysiological signs, thereby affecting motor function in patients with PD (24). In a previous study, excessive iron deposition was reported to affect the function of the nigrostriatal pathway, which led to movement disorders (39). Studies have found that the pattern of iron deposition in patients with different dyskinesia subtypes is also different and tends to be stratified according to the severity of dyskinesia (38,40), which is consistent with our findings.
Clinical index analysis of patients and controls
PD is a comprehensive neurodegenerative disease. Although PD can manifest as motor system dysfunction, nonmotor symptoms are also prominent features of this disease (41). Our results indicate that the velocity of the SSP, the stride length the SSP, the velocity of the FP, and the stride length FP of the HC group were higher than those of the PD group, suggesting that movement disorders are still important clinical manifestations of patients with PD. Therefore, the degree of movement impairment in patients with PD can be quantified via gait measurement. As PD spreads through the brain, patients develop cognitive and psychiatric disorders (42). Our results also showed that the BAI and BDI scores in the PD group were higher than those in the HC group, which confirmed that patients with PD were more affected by emotions and were prone to depression, anxiety, and other symptoms.
Levodopa is the most effective symptomatic treatment for dopamine deficiency in PD and can effectively improve the condition of patients with PD (43). We also found that in the PD group, the MDS-UPDRS and the MDS-UPDRS-III in the OFF state were significantly higher than those in the ON state, which indicated that levodopa had a significant effect on the relief of PD movement symptoms. This suggests that the patients we studied were patients with dopamine-responsive FOG, in which FOG appears mainly in the OFF stage and that dopamine can significantly improve its symptoms.
In patients with PD, we did not find a significant correlation between the velocity, stride length, stride time, cadence, or iron deposition in the brain both in the SSP and FP conditions. However, we can see that the gait information of the patients tended to correlate with iron deposition in the substantia nigra according to our results. However, this correlation was not statistically meaningful possibly due to the small number of patients with FOG in our study and may be a possible explanation for the development of gait disorders in patients with PD. A variety of gait indicators can be used to enrich the dimension of evaluation, accurately measure objective parameters, and develop the research into imaging combined with computerized gait evaluation. Although we did not find a significant correlation between the gait information or iron deposition in the brain, gait disorders in patients with PD tended to increase with increased iron deposition in the brain. There are few related studies on the quantitative analysis of gait data and iron deposition in the brain, which will be the direction of our future research.
Limitations
Some limitations in this study should be mentioned. First, due to the limitations of the age of the patients and the severity of the disease, the total sample size was perhaps too small. Most of the patients had motor symptoms, but FOG only accounted for approximately half of these symptoms, so we need to expand the number of patients with FOG in further studies to clarify the role of dopaminergic drugs in FOG. Second, this study only found significant differences in 1 brain region of the substantia nigra, but did verify the biological role of iron deposition in the substantia nigra in PD. On the other hand, it showed that QSM technology may have certain limitations. Other components in the brain, such as iron in oligodendrocytes, affect the magnetic susceptibility of the tissue (44), which in turn results in a certain degree of imaging of the QSM value. Nevertheless, the quantitative analysis of QSM is generally more specific, deeper, and more comprehensive than other MR methods such as SWI, R2, R2*, and R2' in exploring the correlation between gait measurement and brain iron deposition in patients with FOG.
Conclusions
Collectively, our findings suggest that the iron deposition in the substantia nigra in patients with PD is increased compared with that of controls, which suggests that iron deposition in the substantia nigra is a credible indicator of PD. In addition, we found that iron deposition in the substantia nigra was associated with FOG, which indicates that iron deposition could be used as a basis for evaluating the motor function of patients with PD. As a new quantitative susceptibility measurement technique, QSM represent a powerful tool for evaluating the progression of PD.
Acknowledgments
We would like to express a deep thanks to the staff from the Department of Neurology, Beijing Friendship Hospital, Capital Medical University, for their support of our research.
Funding: This work was supported by grants from
Footnote
Reporting Checklist: The authors have completed the STROBE reporting checklist. Available at https://qims.amegroups.com/article/view/10.21037/qims-23-267/rc
Conflicts of Interest: All authors have completed the ICMJE uniform disclosure form (available at https://qims.amegroups.com/article/view/10.21037/qims-23-267/coif). The authors have no conflicts of interest to declare.
Ethical Statement: The authors are accountable for all aspects of the work in ensuring that questions related to the accuracy or integrity of any part of the work are appropriately investigated and resolved. This study was conducted in accordance with the Declaration of Helsinki (as revised in 2013) and was approved by the ethics committee of Beijing Friendship Hospital, Capital Medical University (No. 2023-P2-110-02). All participants involved signed an informed consent form.
Open Access Statement: This is an Open Access article distributed in accordance with the Creative Commons Attribution-NonCommercial-NoDerivs 4.0 International License (CC BY-NC-ND 4.0), which permits the non-commercial replication and distribution of the article with the strict proviso that no changes or edits are made and the original work is properly cited (including links to both the formal publication through the relevant DOI and the license). See: https://creativecommons.org/licenses/by-nc-nd/4.0/.
References
- Song W, Raza HK, Lu L, Zhang Z, Zu J, Zhang W, Dong L, Xu C, Gong X, Lv B, Cui G. Functional MRI in Parkinson's disease with freezing of gait: a systematic review of the literature. Neurol Sci 2021;42:1759-71. [Crossref] [PubMed]
- Hely MA, Reid WG, Adena MA, Halliday GM, Morris JG. The Sydney multicenter study of Parkinson's disease: the inevitability of dementia at 20 years. Mov Disord 2008;23:837-44. [Crossref] [PubMed]
- Bloem BR, Okun MS, Klein C. Parkinson's disease. Lancet 2021;397:2284-303. [Crossref] [PubMed]
- Nonnekes J, Snijders AH, Nutt JG, Deuschl G, Giladi N, Bloem BR. Freezing of gait: a practical approach to management. Lancet Neurol 2015;14:768-78. [Crossref] [PubMed]
- Wei X, Wang Z, Zhang M, Li M, Chen YC, Lv H, Tuo H, Yang Z, Wang Z, Ba F. Brain Surface Area Alterations Correlate With Gait Impairments in Parkinson's Disease. Front Aging Neurosci 2022;14:806026. [Crossref] [PubMed]
- Tomlinson CL, Stowe R, Patel S, Rick C, Gray R, Clarke CE. Systematic review of levodopa dose equivalency reporting in Parkinson's disease. Mov Disord 2010;25:2649-53. [Crossref] [PubMed]
- An H, Zeng X, Niu T, Li G, Yang J, Zheng L, Zhou W, Liu H, Zhang M, Huang D, Li J. Quantifying iron deposition within the substantia nigra of Parkinson's disease by quantitative susceptibility mapping. J Neurol Sci 2018;386:46-52. [Crossref] [PubMed]
- Foley PB, Hare DJ, Double KL. A brief history of brain iron accumulation in Parkinson disease and related disorders. J Neural Transm (Vienna) 2022;129:505-20. [Crossref] [PubMed]
- Thirupathi A, Chang YZ. Brain Iron Metabolism and CNS Diseases. Adv Exp Med Biol 2019;1173:1-19. [Crossref] [PubMed]
- Naduthota RM, Honnedevasthana AA, Lenka A, Saini J, Geethanath S, Bharath RD, Christopher R, Yadav R, Gupta AK, Pal PK. Association of freezing of gait with nigral iron accumulation in patients with Parkinson's disease. J Neurol Sci 2017;382:61-5. [Crossref] [PubMed]
- Ward RJ, Zucca FA, Duyn JH, Crichton RR, Zecca L. The role of iron in brain ageing and neurodegenerative disorders. Lancet Neurol 2014;13:1045-60. [Crossref] [PubMed]
- Burgetova R, Dusek P, Burgetova A, Pudlac A, Vaneckova M, Horakova D, Krasensky J, Varga Z, Lambert L. Age-related magnetic susceptibility changes in deep grey matter and cerebral cortex of normal young and middle-aged adults depicted by whole brain analysis. Quant Imaging Med Surg 2021;11:3906-19. [Crossref] [PubMed]
- Mochizuki H, Choong CJ, Baba K. Parkinson's disease and iron. J Neural Transm (Vienna) 2020;127:181-7. [Crossref] [PubMed]
- Pietracupa S, Martin-Bastida A, Piccini P. Iron metabolism and its detection through MRI in parkinsonian disorders: a systematic review. Neurol Sci 2017;38:2095-101. [Crossref] [PubMed]
- Yan F, He N, Lin H, Li R. Iron deposition quantification: Applications in the brain and liver. J Magn Reson Imaging 2018;48:301-17. [Crossref] [PubMed]
- Schweser F, Deistung A, Reichenbach JR. Foundations of MRI phase imaging and processing for Quantitative Susceptibility Mapping (QSM). Z Med Phys 2016;26:6-34. [Crossref] [PubMed]
- Pyatigorskaya N, Santin MD. QSM as a new powerful tool for clinical practice in neuroimaging. J Neuroradiol 2021;48:25-7. [Crossref] [PubMed]
- Chen K, Zhang L, Mao H, Chen K, Shi Y, Meng X, Wang F, Hu X, Fang X. The impact of iron deposition on the fear circuit of the brain in patients with Parkinson's disease and anxiety. Front Aging Neurosci 2023;15:1116516. [Crossref] [PubMed]
- Wang F, Zhang M, Li Y, Li Y, Gong H, Li J, Zhang Y, Zhang C, Yan F, Sun B, He N, Wei H. Alterations in brain iron deposition with progression of late-life depression measured by magnetic resonance imaging (MRI)-based quantitative susceptibility mapping. Quant Imaging Med Surg 2022;12:3873-88. [Crossref] [PubMed]
- Kim MJ, Oh SB, Kim J, Kim K, Ryu HS, Kim MS, Ayton S, Bush AI, Lee JY, Chung SJ. Association of metals with the risk and clinical characteristics of Parkinson's disease. Parkinsonism Relat Disord 2018;55:117-21. [Crossref] [PubMed]
- Thomas GEC, Leyland LA, Schrag AE, Lees AJ, Acosta-Cabronero J, Weil RS. Brain iron deposition is linked with cognitive severity in Parkinson's disease. J Neurol Neurosurg Psychiatry 2020;91:418-25. [Crossref] [PubMed]
- Wieler M, Gee M, Camicioli R, Martin WR. Freezing of gait in early Parkinson's disease: Nigral iron content estimated from magnetic resonance imaging. J Neurol Sci 2016;361:87-91. [Crossref] [PubMed]
- Zhang X, Li L, Qi L, Fu Y, Sun D, Chen S, Xu W, Liu C, Zhou X, He G. Distribution pattern of iron deposition in the basal ganglia of different motor subtypes of Parkinson's disease. Neurosci Lett 2023;807:137249. [Crossref] [PubMed]
- Uchida Y, Kan H, Sakurai K, Inui S, Kobayashi S, Akagawa Y, Shibuya K, Ueki Y, Matsukawa N. Magnetic Susceptibility Associates With Dopaminergic Deficits and Cognition in Parkinson's Disease. Mov Disord 2020;35:1396-405. [Crossref] [PubMed]
- Hughes AJ, Daniel SE, Ben-Shlomo Y, Lees AJ. The accuracy of diagnosis of parkinsonian syndromes in a specialist movement disorder service. Brain 2002;125:861-70. [Crossref] [PubMed]
- Opara J, Małecki A, Małecka E, Socha T. Motor assessment in Parkinson`s disease. Ann Agric Environ Med 2017;24:411-5. [Crossref] [PubMed]
- Liu Z, Spincemaille P, Yao Y, Zhang Y, Wang Y. MEDI+0: Morphology enabled dipole inversion with automatic uniform cerebrospinal fluid zero reference for quantitative susceptibility mapping. Magn Reson Med 2018;79:2795-803. [Crossref] [PubMed]
- Cusack R, Papadakis N. New robust 3-D phase unwrapping algorithms: application to magnetic field mapping and undistorting echoplanar images. Neuroimage 2002;16:754-64. [Crossref] [PubMed]
- Zhang Y, Brady M, Smith S. Segmentation of brain MR images through a hidden Markov random field model and the expectation-maximization algorithm. IEEE Trans Med Imaging 2001;20:45-57. [Crossref] [PubMed]
- Jenkinson M, Bannister P, Brady M, Smith S. Improved optimization for the robust and accurate linear registration and motion correction of brain images. Neuroimage 2002;17:825-41. [Crossref] [PubMed]
- Guan X, Xuan M, Gu Q, Huang P, Liu C, Wang N, Xu X, Luo W, Zhang M. Regionally progressive accumulation of iron in Parkinson's disease as measured by quantitative susceptibility mapping. NMR Biomed 2017;30: [Crossref] [PubMed]
- Ghassaban K, He N, Sethi SK, Huang P, Chen S, Yan F, Haacke EM. Regional High Iron in the Substantia Nigra Differentiates Parkinson's Disease Patients From Healthy Controls. Front Aging Neurosci 2019;11:106. [Crossref] [PubMed]
- Langkammer C, Pirpamer L, Seiler S, Deistung A, Schweser F, Franthal S, Homayoon N, Katschnig-Winter P, Koegl-Wallner M, Pendl T, Stoegerer EM, Wenzel K, Fazekas F, Ropele S, Reichenbach JR, Schmidt R, Schwingenschuh P. Quantitative Susceptibility Mapping in Parkinson's Disease. PLoS One 2016;11:e0162460. [Crossref] [PubMed]
- Rao IY, Hanson LR, Johnson JC, Rosenbloom MH, Frey WH 2nd. Brain Glucose Hypometabolism and Iron Accumulation in Different Brain Regions in Alzheimer's and Parkinson's Diseases. Pharmaceuticals (Basel) 2022.
- Fu X, Deng W, Cui X, Zhou X, Song W, Pan M, Chi X, Xu J, Jiang Y, Wang Q, Xu Y. Time-Specific Pattern of Iron Deposition in Different Regions in Parkinson's Disease Measured by Quantitative Susceptibility Mapping. Front Neurol 2021;12:631210. [Crossref] [PubMed]
- Falla M, Cossu G, Di Fonzo A. Freezing of gait: overview on etiology, treatment, and future directions. Neurol Sci 2022;43:1627-39. [Crossref] [PubMed]
- Zhang W, Yang Z, Li H, Huang D, Wang L, Wei Y, Zhang L, Ma L, Feng H, Pan J, Guo Y, Chan P. Multimodal Data for the Detection of Freezing of Gait in Parkinson's Disease. Sci Data 2022;9:606. [Crossref] [PubMed]
- Chen J, Cai T, Li Y, Chi J, Rong S, He C, Li X, Zhang P, Wang L, Zhang Y. Different iron deposition patterns in Parkinson's disease subtypes: a quantitative susceptibility mapping study. Quant Imaging Med Surg 2020;10:2168-76. [Crossref] [PubMed]
- Song T, Li J, Mei S, Jia X, Yang H, Ye Y, Yuan J, Zhang Y, Lu J. Nigral Iron Deposition Is Associated With Levodopa-Induced Dyskinesia in Parkinson's Disease. Front Neurosci 2021;15:647168. [Crossref] [PubMed]
- Homayoon N, Pirpamer L, Franthal S, Katschnig-Winter P, Kögl M, Seiler S, Wenzel K, Hofer E, Deutschmann H, Fazekas F, Langkammer C, Ropele S, Schmidt R, Schwingenschuh P. Nigral iron deposition in common tremor disorders. Mov Disord 2019;34:129-32. [Crossref] [PubMed]
- Chendo I, Silva C, Duarte GS, Prada L, Voon V, Ferreira JJ. Frequency and Characteristics of Psychosis in Parkinson's Disease: A Systematic Review and Meta-Analysis. J Parkinsons Dis 2022;12:85-94. [Crossref] [PubMed]
- Fénelon G, Parant J, Cleret de Langavant L. Victor Parant (1848-1924) and the first report of psychosis in the course of Parkinson's disease with dementia. Rev Neurol (Paris) 2021;177:1221-7. [Crossref] [PubMed]
- Rosqvist K, Horne M, Hagell P, Iwarsson S, Nilsson MH, Odin P. Levodopa Effect and Motor Function in Late Stage Parkinson's Disease. J Parkinsons Dis 2018;8:59-70. [Crossref] [PubMed]
- Nikparast F, Ganji Z, Danesh Doust M, Faraji R, Zare H. Brain pathological changes during neurodegenerative diseases and their identification methods: How does QSM perform in detecting this process? Insights Imaging 2022;13:74. [Crossref] [PubMed]