Three-dimensional echocardiography and strain cardiac imaging in patients with prediabetes and type 2 diabetes mellitus
Introduction
Type 2 diabetes mellitus (T2DM) is an independent risk factor for cardiovascular diseases (1). The connection between diabetes and heart failure was observed more than 100 years ago, and it was recognized that heart failure was a common and noteworthy complication of diabetes (2). Diabetic cardiomyopathy (DCM) was defined as a myocardial disease independent of coronary arteriosclerotic heart disease, hypertension, and valvular disease (3). Currently, the criteria for the diagnosis of DCM include left ventricular (LV) diastolic insufficiency and/or reduced LV ejection fraction (LVEF), pathological LV hypertrophy, and interstitial fibrosis (4).
Numerous studies have confirmed that DCM leads to alterations in the LV structure and function (5,6), and early studies have considered LV diastolic dysfunction as the first marker of DCM, with advanced disease affecting systolic function (7). However, studies have shown that a reduction in LV systolic strain can be observed in diabetic patients without any obvious signs or symptoms of disease; therefore, researchers believe that a reduction in strain parameters representing changes in myocardial mechanics could be the preferred indicator and therapeutic target for LV systolic dysfunction in the early stages of DCM (8,9). Reports have shown that two-dimensional (2D) speckle tracking technology is a promising left LV evaluation technology (10,11). However, 2D speckle tracking echocardiography (2D-STE) is based on 2D planar tracking technology, which has limitations for out-of-plane cardiac motion. Moreover, 2D-STE methodology requires good image quality and optimization of frame rates, depends on the temporal stability of tracking patterns, and may be influenced by the anterior chest wall conformation (12,13). In recent years, with the rapid development of myocardial deformation imaging, three-dimensional (3D) speckle tracking technology has gradually moved from laboratory and animal research to clinical research, and is expected to become a new clinical diagnostic tool for the comprehensive and reliable evaluation of myocardial function. The tracking of myocardial spots by 3D-STE is extended from the original 2D space to the 3D space, which not only significantly increases the number of tracked myocardial spots, but can also obtain multidimensional space data in the same cardiac cycle, thus improving the accuracy, repeatability, and time efficiency of echocardiography in LV function analysis (14,15). In addition, 3D-STE can obtain new LV deformation parameters and provide more incremental information for mechanical changes of the left ventricle, such as area strain, cardiac twist, and torsion.
Theoretically, 3D-STE has potential applications in the diagnosis and treatment of any disease affecting myocardial deformation; however, there are few reports on whether 3D-STE is superior to 2D-STE in the assessment of subclinical LV systolic dysfunction in prediabetes and T2DM. The purpose of this study was to investigate the clinical application of 3D-STE in the evaluation of LV myocardial mechanical changes in prediabetic and diabetic patients with preserved LVEF by comparing the corresponding LV strain parameters of 3D-STE and 2D-STE and to explore the independent risk factors for subclinical LV systolic dysfunction in the prediabetic and diabetic populations.
Methods
Study population
This observational study was conducted from January 2021 to December 2022 and included 40 patients with T2DM, 35 prediabetic patients, and 33 healthy volunteers. All participants had blood pressure (BP) <140/90 mmHg and LVEF >52%. The diagnostic criteria for prediabetes and diabetes were in accordance with the 2020 guidelines of the American Diabetes Association and World Health Organization (16). The subgroups were as follows glycosylated hemoglobin A1c (HbA1c) ≥6.5% as the diabetic group, 5.7–6.4% as the prediabetic group, and <5.7% as the control group. The exclusion criteria were as follows: patients with coronary artery disease, hypertension, congenital heart disease, cardiomyopathy, and serious renal dysfunction (glomerular filtration rate <30 mL/min/1.73 m2). The study was conducted in accordance with the Declaration of Helsinki (revised in 2013), and was reviewed and approved by the Institutional Ethics Committee of the Second Affiliated Hospital of Nanchang University. All participants gave informed consent and signed an informed consent form.
Echocardiography
Echocardiographic examinations were performed using a GE Healthcare Vivid E95 ultrasonic diagnostic system equipped with EchoPAC (version 201; GE Healthcare, Chicago, IL, USA) offline analysis software. Routine transthoracic echocardiography was performed with respiratory and rhythm stabilization. Left atrium anteroposterior diameter (LAD), LV end-diastolic and end-systolic diameters (LVEDd and LVESd, respectively), interventricular septum (IVS), and LV posterior wall (LVPW) thickness in diastole were measured by M-mode echocardiography in LV long-axis views. LVEF was measured by biplane Simpson’s method. Peak E velocity and peak A velocity of the mitral orifice were calculated using pulsed Doppler in an apical 4-chamber view. Early peak diastolic velocity (e') of the mitral annulus was measured in an apical 4-chamber cardiac view using tissue Doppler imaging, and an average e' of the septal and lateral mitral annulus was computed. The E/A and E/e' values represent indices of LV filling pressure. All data were measured 3 times and averaged. All parameters were recorded and taken by the guidelines of the United States Society of Echocardiography (17).
STE
The 2D dynamic images of the LV apical 4-, 3-, and 2-chamber views, and 3 short-axis views of the left ventricle in 3 consecutive cardiac cycles at a frame rate of 50–70 frames per second (fps) were stored. The stored dynamic images were transferred to the EchoPAC (version 201) workstation, automatic functional imaging (AFI) was initiated, and the LV long-axis and short-axis dynamic images were selected in sequence. The software automatically divides the left ventricle into 17 segments and tracks the myocardial 3-layer motion trajectory, manually adjusts the unsatisfactory segments, and the system automatically calculates the 2D global longitudinal strain (GLS) and GCS. LV 3D echocardiographic data were obtained from the apical 4-chamber view using a 4V-D cardiac 3D volume transducer. Acquisition was conducted at a frame rate of 25–50 fps [or greater than (heart rate × 40%) fps]. The image should include the entire LV chamber and subepicardial myocardium. After ensuring the best temporal and spatial resolutions, 3D images of three consecutive cardiac cycles were stored. The images were imported into Echo PAC and 4D Auto LVQ software was launched, which automatically tracks the endocardial and epicardial contours and covers the myocardial thickness of the entire LV wall with manual adjustments for unsatisfactory segments. The software automatically tracks the LV myocardial motion, and finally, the system automatically analyzes the LV volume, ejection fraction, LV mass index, strain curve, and bull’s eye map of 3D GLS, GCS, GAS, and GRS (Figure 1). Strain is negative since it is a measure of myocardial shortening, and all strains used in this paper are absolute values.
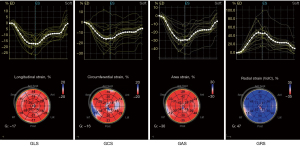
Reproducibility of 3D-STE
A total of 30 participants were selected using a simple random sampling method. To verify the reliability of inter-observer repeatability, the 3D strain parameters of the left ventricle were obtained by two attending physicians with experience in analysis according to the above analysis method. The second observer analyzed the data without knowing the results of the first observer’s measurements. To verify intra-observer repeatability, a physician performed two data analyses at 1-week intervals.
Statistical methods
All statistical analyses were performed using IBM SPSS Statistics for Windows 22.0 (IBM Corp., Armonk, NY, USA). Continuous variables were expressed as mean ± standard deviation (SD) or median (interquartile range), and multiple variables were compared using one-way analysis of variance (ANOVA) and Bonferroni post hoc multiple comparisons. GLS and GCS were measured by 2D and 3D and were compared using paired t-tests. Categorical variables were compared using the chi-squared test. Correlation was determined using a bivariate, two-tailed Pearson correlation test. Intra- and inter-observer agreements were assessed using the Bland-Altman analysis. Receiver operating characteristic (ROC) curve was constructed to determine the clinical diagnostic value of 3D-STE in evaluating subclinical LV dysfunction in patients with prediabetes and T2DM. Univariate and multivariate regression models were used to analyze independent risk factors for LV subclinical systolic dysfunction in the prediabetic and T2DM populations. Statistical significance was considered when P<0.05.
Results
Demographic and clinical characteristics
The body mass index (BMI) of the diabetic group was higher than that of the control group, and the difference between the two groups was statistically significant (25.41±3.89 vs. 22.37±3.20 kg/m2, P=0.003). The heart rate, systolic BP (SBP), and diastolic BP (DBP) of the prediabetes and diabetes groups were significantly higher than those of the control group (P<0.05). Plasma glucose (Glu) levels in the T2DM group were higher than those in the prediabetes and control groups (P<0.05), but there was no statistical difference between the prediabetes and control groups. HbA1c increased sequentially in the control, prediabetes, and diabetes groups, and there were statistically significant differences between the three groups (P<0.001) (Table 1).
Table 1
Parameters | CON (n=33) | TH1 (n=35) | TH2 (n=40) | P value | ||
---|---|---|---|---|---|---|
TH1 vs. CON | TH2 vs. CON | TH1 vs. TH2 | ||||
Age (years) | 48.42±13.27 | 54.51±11.63 | 53.30±12.01 | 0.131 | 0.284 | >0.999 |
Sex (male) | 18 (54.54) | 20 (57.14) | 20 (50.00) | 0.511 | 0.440 | 0.350 |
Duration (years) | – | 2.72±2.22 | 7.52±7.11 | – | – | <0.001 |
BMI (kg/m²) | 22.37±3.20 | 24.13±4.31 | 25.41±3.89 | 0.187 | 0.003 | 0.460 |
HR (bpm) | 68.42±8.98 | 74.91±12.50 | 76.34±9.67 | 0.036 | 0.005 | >0.999 |
SBP (mmHg) | 117.45±12.20 | 125.65±8.76 | 124.87±11.88 | 0.009 | 0.016 | >0.999 |
DBP (mmHg) | 71.78±9.35 | 79.20±7.08 | 78.30±7.93 | 0.001 | 0.003 | >0.999 |
Laboratory tests | ||||||
Glu (mmol/L) | 5.35±0.73 | 6.80±1.83 | 11.62±6.01 | 0.363 | <0.001 | <0.001 |
HbA1c (%) | 5.06±0.48 | 6.11±0.94 | 9.20±2.27 | 0.016 | <0.001 | <0.001 |
TC (mmol/L) | 5.00±2.07 | 5.37±1.97 | 4.84±1.11 | >0.999 | >0.999 | 0.584 |
TG (mmol/L) | 1.48±0.66 | 2.57±2.34 | 2.26±1.43 | 0.021 | 0.135 | >0.999 |
Cr (μmol/L) | 57.78±14.88 | 69.45±27.47 | 68.76±26.51 | 0.141 | 0.160 | >0.999 |
Urea (mmol/L) | 5.20±1.57 | 6.29±3.53 | 5.62±1.52 | 0.187 | >0.999 | 0.692 |
DM medication | ||||||
Melbinum | – | – | 10 (25.0) | – | – | – |
Sulfonylureas | – | – | 10 (25.0) | – | – | – |
α-GSDI | – | – | 8 (20.0) | – | – | – |
TZDs | – | – | 5 (12.5) | – | – | – |
Insulin | – | – | 4 (10.0) | – | – | – |
Diet treatment | – | – | 3 (7.5) | – | – | – |
Data are expressed as mean ± SD or n (%). P<0.05 was considered statistically significant. CON, the control group; TH1, the prediabetes group; TH2, the diabetes group; BMI, body mass index; HR, heart rate; SBP, systolic blood pressure; DBP, diastolic blood pressure; Glu, glucose; HbA1c, hemoglobin A1c; TC, total cholesterol; TG, triglyceride; Cr, creatinine; DM, diabetes mellitus; α-GSDI, α-glucosidase inhibitor; TZDs, thiazolidinedione drugs; SD, standard deviation.
Comparison of conventional echocardiographic parameters
IVS and LVPW thickness in diastole were significantly higher in the diabetes group than in the control and prediabetes groups (P<0.05), but there was no statistical difference between the controls and the prediabetic group. Compared with the control group, the E value, and E/A value were significantly higher in the prediabetes and diabetes groups (P<0.05), but there was no statistical difference between the prediabetes and diabetes groups. The E/e value of the diabetic group was significantly higher than that of the control group (P<0.05), but there was no significant difference in E/e values in the prediabetic group compared with the other two groups (Table 2).
Table 2
Parameters | CON (n=33) | TH1 (n=35) | TH2 (n=40) | P value | ||
---|---|---|---|---|---|---|
TH1 vs. CON | TH2 vs. CON | TH1 vs. TH2 | ||||
LAD (mm) | 32.21±4.14 | 32.54±4.08 | 33.73±2.99 | >0.999 | 0.264 | 0.523 |
LVEDd (mm) | 45.94±4.06 | 45.57±3.87 | 44.65±3.17 | >0.999 | 0.421 | 0.849 |
LVESd (mm) | 28.93±3.39 | 30.65±3.18 | 29.85±3.09 | 0.085 | 0.680 | 0.828 |
IVSd (mm) | 8.85±1.06 | 9.22±1.19 | 10.05±1.11 | 0.497 | <0.001 | 0.006 |
LVPWd (mm) | 8.24±1.06 | 8.57±1.24 | 9.42±1.11 | 0.711 | <0.001 | 0.005 |
2D-LVEF (%) | 62.00±1.94 | 60.45±1.92 | 60.75±3.75 | 0.068 | 0.167 | >0.999 |
E (cm/s) | 86.91±20.33 | 65.61±16.30 | 69.28±15.07 | <0.001 | <0.001 | >0.999 |
E/A | 1.33±0.43 | 0.88±0.27 | 0.91±0.33 | <0.001 | <0.001 | >0.999 |
E/e' | 9.54±3.62 | 10.29±3.83 | 12.21±3.50 | >0.999 | 0.007 | 0.075 |
LVEDV (mL) | 86.32±18.68 | 83.22±19.30 | 84.43±19.10 | >0.999 | >0.999 | >0.999 |
LVESV (mL) | 33.03±10.36 | 34.71±10.95 | 34.85±9.47 | 0.999 | >0.999 | >0.999 |
3D-LVEF (%) | 61.73±6.59 | 58.73±6.49 | 58.66±4.83 | 0.121 | 0.092 | >0.999 |
Data are expressed as mean ± SD. P<0.05 was considered statistically significant. CON, control group; TH1, prediabetes group; TH2, diabetes group; LAD, left atrium anteroposterior diameter; LVEDd, left ventricular end-diastolic diameter; LVESd, left ventricular end-systolic diameter; IVSd, interventricular septum thickness in diastole; LVPWd, left ventricular posterior wall end-diastolic dimension; 2D, two-dimensional; LVEF, left ventricular ejection fraction; E, early diastolic mitral inflow velocity; A, late diastolic mitral inflow velocity; e', early diastolic mitral annular tissue velocity; LVEDV, left ventricular end-diastolic volume; LVESV, left ventricular end-systolic volume; 3D, three-dimensional; SD, standard deviation.
3D strain parameters
A total of 12 of the 1,836 segments were not used for strain calculation (1%) because the software was not satisfactory for myocardial tracking of the LV segments. 3D-STE analysis showed that GLS (18.64%±2.43% vs. 15.21%±1.49% vs. 13.49%±2.36%), GCS (18.09%±2.37% vs. 14.62%±1.75% vs. 12.95%±2.20%), GAS (31.30%±3.88% vs. 27.51%±3.31% vs. 24.80%±3.86%), and GRS (49.18%±5.91% vs. 39.17%±4.55% vs. 35.72%±7.19%) decreased in the controls, prediabetes patients, and the diabetes patients consecutively, and there was a statistical difference between the three groups (P <0.001) (Table 3, Figure 2). Paired t-test showed statistical differences in GLS and GCS between 2D-STE and 3D-STE (GLS: 15.62±3.02 vs. 18.84±2.80, P<0.001; GCS: 15.06±2.99 vs. 17.79±3.11, P<0.001 for 2D-STE and 3D-STE, respectively) (Table 4).
Table 3
Parameters | CON (n=33) | TH1 (n=35) | TH2 (n=40) | P value | ||
---|---|---|---|---|---|---|
TH1 vs. CON | TH2 vs. CON | TH1 vs. TH2 | ||||
GLS (%) | 18.64±2.43 | 15.21±1.49 | 13.49±2.36 | <0.001 | <0.001 | 0.002 |
GCS (%) | 18.09±2.37 | 14.62±1.75 | 12.95±2.20 | <0.001 | <0.001 | 0.003 |
GAS (%) | 31.30±3.88 | 27.51±3.31 | 24.80±3.86 | <0.001 | <0.001 | 0.006 |
GRS (%) | 49.18±5.91 | 39.17±4.55 | 35.72±7.19 | <0.001 | <0.001 | 0.046 |
Data are expressed as mean ± SD. P<0.05 was considered statistically significant. 3D, three-dimensional; CON, control group; TH1, prediabetes group; TH2, diabetes group; GLS, global longitudinal strain; GCS, global circumferential strain; GAS, global area strain; GRS, global radial strain; SD, standard deviation.
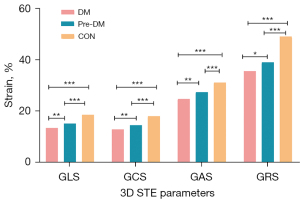
Table 4
Parameters | 3D-STE (n=108) | 2D-STE (n=108) | P value |
---|---|---|---|
GLS (%) | 15.62±3.02 | 18.84±2.80 | <0.001 |
GCS (%) | 15.06±2.99 | 17.79±3.11 | <0.001 |
Data are expressed as mean ± SD. P<0.05 was considered statistically significant. 3D, three-dimensional; 2D, two-dimensional; STE, speckle tracking echocardiography; GLS, global longitudinal strain; GCS, global circumferential strain; SD, standard deviation.
ROC analysis
According to the 2016 United States Echocardiography and European Association of Cardiovascular Imaging recommendations for the echocardiographic assessment of LV diastolic dysfunction (17), patients with abnormal blood Glu levels were divided into a diastolic dysfunction group (n=51) and a normal diastolic function group (n=24). The ROC curve showed that the area under the curve (AUC) of 3D-STE global strain parameters (GLS, GCS, GAS, GRS) was 0.898, 0.831, 0.863, and 0.868, respectively, and the AUC of 2D-STE global strain parameters (GLS, GCS) was 0.867 and 0.636, respectively (Figure 3).
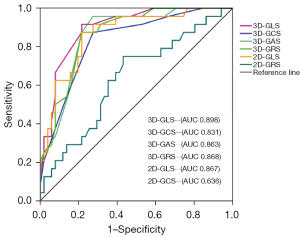
Independent risk factors for subclinical LV systolic dysfunction
Table 5 illustrates the risk factors for subclinical LV systolic dysfunction in the entire study population. Variables with a value of P<0.05 in the univariate analysis [i.e., BMI, duration, heart rate, SBP, DBP, Glu, HbA1c, and triglyceride (TG)] were included in the stepwise multivariate regression model. Multivariate regression analysis revealed that HbA1c (β=−0.588, P<0.001) and BMI (β=−0.215, P=0.001) were independent risk factors for subclinical LV systolic dysfunction in patients with prediabetes and T2DM.
Table 5
Parameters | Univariate | Multivariate | |||||
---|---|---|---|---|---|---|---|
β | t value | P value | β | t value | P value | ||
BMI (kg/m2) | −0.292 | −4.340 | 0.011 | −0.215 | −3.513 | 0.001 | |
Duration (years) | |||||||
<1 | −4.243 | −6.875 | <0.001 | – | – | – | |
1–5 | −3.650 | −6.154 | <0.001 | – | – | – | |
6–10 | −4.496 | −7.062 | <0.001 | – | – | – | |
>10 | −5.824 | −7.566 | <0.001 | – | – | – | |
Heart rate (bpm) | −0.076 | −2.962 | 0.004 | – | – | – | |
SBP (mmHg) | −0.06 | −2.411 | 0.018 | – | – | – | |
DBP (mmHg) | −0.114 | −3.587 | 0.001 | – | – | – | |
Glu (mmol/L) | −0.214 | −3.617 | <0.001 | – | – | – | |
HbA1c (%) | −0.665 | −6.182 | <0.001 | −0.588 | −3.513 | <0.001 | |
TG (mmol/L) | −0.537 | −3.232 | 0.002 | – | – | – |
LV, left ventricular; BMI, body mass index; SBP, systolic blood pressure; DBP, diastolic blood pressure; Glu, glucose; HbA1c, hemoglobin A1c; TG, triglyceride.
Repeatability test of LV 3D strain parameters
There was good intra-and inter-observer agreement for the LV systolic 3D global strain parameters. Bland-Altman analysis showed that the intraclass correlation coefficient (ICC) in the GLS, GCS, GAS, and GRS groups ranged from 0.963 to 0.981 and from 0.956 to 0.985, respectively (Table 6, Figure 4).
Table 6
Parameters | Intra-observer variability | Inter-observer variability | |||
---|---|---|---|---|---|
ICC | 95% CI | ICC | 95% CI | ||
GLS (%) | 0.981 | 0.907–0.991 | 0.985 | 0.918–0.993 | |
GCS (%) | 0.970 | 0.881–0.972 | 0.956 | 0.831–0.959 | |
GAS (%) | 0.963 | 0.857–0.966 | 0.962 | 0.851–0.964 | |
GRS (%) | 0.970 | 0.883–0.972 | 0.966 | 0.865–0.968 |
3D, three-dimensional; ICC, intraclass correlation coefficient; CI, confidence interval; GLS, global longitudinal strain; GCS, global circumferential strain; GAS, global area strain; GRS, global radial strain.
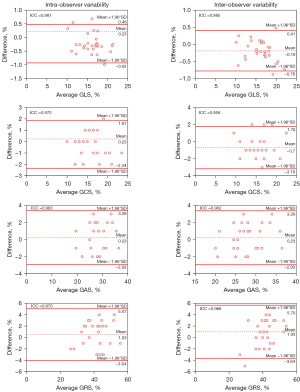
Discussion
This study has three main findings: (I) patients with prediabetes and T2DM with preserved LVEF had subclinical LV systolic dysfunction, which was characterized by a progressive decrease in global systolic strain with increasing blood Glu levels. (II) 3D-STE is a reliable clinical imaging technique for evaluating LV systolic function. GAS is a unique strain parameter of 3D-STE that may be a new sensitive index for the early detection of subclinical LV dysfunction. (III) Hb1Ac and BMI were independently associated with subclinical LV systolic dysfunction in prediabetic and diabetic patients with preserved LVEF.
Subclinical LV systolic dysfunction is characterized by LV diastolic dysfunction, and LVEF is often within the normal range. At present, several studies have found that there is a decrease in myocardial strain in LV subclinical systolic dysfunction, and some studies suggest that the early stage of LV systolic dysfunction is to maintain LVEF by the compensatory increase in radial or circumferential strain (18-20). Although conventional echocardiography cannot detect this change in the early stage of the disease, it is undeniable that myocardial mechanics have decreased. Due to the complex spiral motion of the three layers of myocardial fibers in the left ventricle to maintain LV function, the disruption of the 3D mechanical balance inevitably leads to discordant or ineffective LV contraction, resulting in LV dysfunction. Dogdus et al. (21) used 3D-STE to analyze the LV 3D global systolic strain parameters of 120 patients who had positive stress tests or were scheduled for coronary angiography after myocardial imaging; the results showed that the 3D global strain parameters of the group with borderline coronary artery disease were significantly reduced compared with those of the group with nonborderline coronary artery disease, and the Gensini score was positively correlated with the 3D LV systolic strain parameters. The results of this study showed that 3D GLS, GCS, GAS, and GRS were significantly reduced in prediabetic and diabetic patients with preserved LVEF, which is consistent with the results of a meta-analysis (22). Therefore, 3D-STE is a non-invasive and accurate imaging technique for detecting subclinical LV systolic dysfunction.
Area strain is a new 3D-STE strain parameter that refers to the percentage of endocardial surface area reduction during LV cardiac deformation in end-systole and end-diastole (23). It can be considered a combination of longitudinal and circumferential strains and reflects the dynamic changes in the LV myocardium in multiple directions (24). The findings of the present study showed that area strain was significantly lower in patients with prediabetes and T2DM than in control cases, confirming the sensitivity of area strain in the assessment of subclinical LV dysfunction. Our results are consistent with the findings of Huang et al. (25), who concluded that GAS is a reliable new parameter for identifying early abnormalities of myocardial function in patients with systemic lupus erythematosus with normal LVEF. Yu et al. (26) applied ROC curve analysis to show that, compared with other 3D strain parameters and LVEF, 3D GAS had the highest AUC for diagnosing children with Duchenne muscular dystrophy. Yu et al. reported that area strain has significant advantages in predicting severe adverse cardiovascular events and could be a good indicator for assessing cardiac function and monitoring prognosis (27).
Although 2D-STE is one of the most commonly used imaging modalities for diagnosing subclinical LV dysfunction, it has certain limitations. It requires more time to acquire 2D images of different cardiac cycles and from different views to obtain strain parameters, whereas 3D-STE allows simultaneous tracking of myocardial motion trajectories in 3D space and real-time acquisition of full-volume LV data, thus allowing a more accurate and comprehensive evaluation of changes in cardiac mechanics. In this study, we found that the global longitudinal and circumferential strain values of the left ventricle on 3D-STE were significantly lower than 2D strain values, and this difference may be because 2D-STE only tracks myocardial motion in the 2D plane and ignores the shortening, thickening, and torsional motion of the left ventricle from the apical to the basal segments (28), and the difference in thickness measured in short-axis sections is thought to be responsible for the apparent variability between 2D and 3D GCS (29). Thus, 3D-STE can provide more reliable LV deformation data than 2D-STE (30). In this study, the diagnostic value of 3D-STE and 2D-STE for subclinical LV dysfunction was evaluated using the ROC curve. The results showed that the global 3D-STE and 2D-STE strain parameters had good diagnostic values; however, the global 3D-STE strain parameters were generally superior to the 2D-STE parameters. The ROC curve area of 3D GLS was the largest among all strain parameters, consistent with the anatomical feature that the left ventricle myocardium consists mainly of longitudinal myocardium responsible for systolic function.
From the comparison of the general clinical data among the three groups, we observed that there were no significant differences in BP levels and lipid profiles. However, the BP and lipid levels were higher in the prediabetes group than in the control and diabetic groups. It could be speculated that the decrease in GLS, GCS, GAS, and GRS in the prediabetes group compared with the control group may be partly attributed to more severe metabolic disturbances in the prediabetes group. Meanwhile, the diabetic group had relatively lower BP and lipid profiles, which may be attributed to the positive effects of diet control and medication during treatment.
Regression analysis in this study showed that HbA1c level and BMI were independent risk factors for subclinical LV systolic dysfunction. Diabetes is a known risk factor for heart failure, and HbA1c is an essential predictor of new-onset heart failure. Each 1% increase in HbA1c levels increases the risk of heart failure by 8–36% (31). Hyperglycemia combined with heart failure is biologically plausible: firstly, hyperglycemia leads to an increase in reactive oxygen species and oxidative stress, which directly damages proteins and DNA and induces apoptosis in cardiomyocytes, leading to cardiac insufficiency (32); secondly, hyperglycemia also activates DNA reparative enzymes and affects glycolytic pathways, which can induce cellular damage and negatively affects myocardial contraction and relaxation (33). Similarly, overweight/obesity is a risk factor for the development of heart failure, and over 80% of heart failure patients with preserved ejection fraction are overweight/obese (34,35), Ho et al. (36) showed that higher circulating leptin concentrations were associated with worse GLS, suggesting that circulating adipokines may play a role in obesity-related LV injury. In addition, obesity leads to the release of pro-inflammatory cytokines and fibrotic factors, leading to cardiac remodeling and exacerbation of the risk of heart failure (37). Therefore, we believe that controlling blood Glu levels as well as reducing body weight can effectively prevent heart failure progression of heart failure.
There were several limitations in our study. First, the sample size was small, which inevitably led to bias in the results. We will conduct a study with a larger sample in the future, which we expect will avoid the effects of the small sample size. Second, no comparison with cardiovascular magnetic resonance imaging was performed in this study. This will be amended soon as our team progresses, and more results will be presented.
Conclusions
This study confirms that the application of 3D-STE can sensitively provide evidence of subclinical LV systolic dysfunction in prediabetic and diabetic patients with preserved ejection fraction, and provides a theoretical basis for proactive strategies to prevent progression to heart failure in a population with abnormally elevated blood Glu.
Acknowledgments
Funding: None.
Footnote
Conflicts of Interest: All authors completed the ICMJE uniform disclosure form (available at https://qims.amegroups.com/article/view/10.21037/qims-23-560/coif). The authors declare no conflicts of interest.
Ethical Statement: The authors are accountable for all aspects of the work, ensuring that questions related to the accuracy or integrity of any part of the work are appropriately investigated and resolved. This study was conducted in accordance with the Declaration of Helsinki (revised in 2013). The study was approved by the Institutional Ethics Committee of the Second Affiliated Hospital of Nanchang University, and all participants provided informed consent and signed an informed consent form.
Open Access Statement: This is an Open Access article distributed in accordance with the Creative Commons Attribution-NonCommercial-NoDerivs 4.0 International License (CC BY-NC-ND 4.0), which permits the non-commercial replication and distribution of the article with the strict proviso that no changes or edits are made and the original work is properly cited (including links to both the formal publication through the relevant DOI and the license). See: https://creativecommons.org/licenses/by-nc-nd/4.0/.
References
- Swoboda PP, McDiarmid AK, Erhayiem B, Ripley DP, Dobson LE, Garg P, Musa TA, Witte KK, Kearney MT, Barth JH, Ajjan R, Greenwood JP, Plein S. Diabetes Mellitus, Microalbuminuria, and Subclinical Cardiac Disease: Identification and Monitoring of Individuals at Risk of Heart Failure. J Am Heart Assoc 2017;6:e005539. [Crossref] [PubMed]
- Lorenzo-Almorós A, Tuñón J, Orejas M, Cortés M, Egido J, Lorenzo Ó. Diagnostic approaches for diabetic cardiomyopathy. Cardiovasc Diabetol 2017;16:28. [Crossref] [PubMed]
- Marwick TH, Ritchie R, Shaw JE, Kaye D. Implications of Underlying Mechanisms for the Recognition and Management of Diabetic Cardiomyopathy. J Am Coll Cardiol 2018;71:339-51. [Crossref] [PubMed]
- Fontes-Carvalho R, Ladeiras-Lopes R, Bettencourt P, Leite-Moreira A, Azevedo A. Diastolic dysfunction in the diabetic continuum: association with insulin resistance, metabolic syndrome and type 2 diabetes. Cardiovasc Diabetol 2015;14:4. [Crossref] [PubMed]
- Jørgensen PG, Jensen MT, Mogelvang R, von Scholten BJ, Bech J, Fritz-Hansen T, Galatius S, Biering-Sørensen T, Andersen HU, Vilsbøll T, Rossing P, Jensen JS. Abnormal echocardiography in patients with type 2 diabetes and relation to symptoms and clinical characteristics. Diab Vasc Dis Res 2016;13:321-30. [Crossref] [PubMed]
- Nabeshima Y, Seo Y, Takeuchi M. A review of current trends in three-dimensional analysis of left ventricular myocardial strain. Cardiovasc Ultrasound 2020;18:23. [Crossref] [PubMed]
- Miki T, Yuda S, Kouzu H, Miura T. Diabetic cardiomyopathy: pathophysiology and clinical features. Heart Fail Rev 2013;18:149-66. [Crossref] [PubMed]
- Tanaka H, Tatsumi K, Matsuzoe H, Matsumoto K, Hirata KI. Impact of diabetes mellitus on left ventricular longitudinal function of patients with non-ischemic dilated cardiomyopathy. Cardiovasc Diabetol 2020;19:84. [Crossref] [PubMed]
- Ernande L, Bergerot C, Rietzschel ER, De Buyzere ML, Thibault H, Pignonblanc PG, Croisille P, Ovize M, Groisne L, Moulin P, Gillebert TC, Derumeaux G. Diastolic dysfunction in patients with type 2 diabetes mellitus: is it really the first marker of diabetic cardiomyopathy? J Am Soc Echocardiogr 2011;24:1268-1275.e1. [Crossref] [PubMed]
- Morris DA, Ma XX, Belyavskiy E, Aravind Kumar R, Kropf M, Kraft R, Frydas A, Osmanoglou E, Marquez E, Donal E, Edelmann F, Tschöpe C, Pieske B, Pieske-Kraigher E. Left ventricular longitudinal systolic function analysed by 2D speckle-tracking echocardiography in heart failure with preserved ejection fraction: a meta-analysis. Open Heart 2017;4:e000630. [Crossref] [PubMed]
- Zhong X, Chen L, Peng G, Sheng Y, Liu X, Zheng Y, Huang Y, Xu J, Liu Y. Early assessment of subclinical myocardial injury in systemic lupus erythematosus by two-dimensional longitudinal layer speckle tracking imaging. Quant Imaging Med Surg 2022;12:2947-60. [Crossref] [PubMed]
- Urbano-Moral JA, Arias-Godinez JA, Ahmad R, Malik R, Kiernan MS, DeNofrio D, Pandian NG, Patel AR. Evaluation of myocardial mechanics with three-dimensional speckle tracking echocardiography in heart transplant recipients: comparison with two-dimensional speckle tracking and relationship with clinical variables. Eur Heart J Cardiovasc Imaging 2013;14:1167-73. [Crossref] [PubMed]
- Sonaglioni A, Nicolosi GL, Rigamonti E, Lombardo M, La Sala L. Molecular Approaches and Echocardiographic Deformation Imaging in Detecting Myocardial Fibrosis. Int J Mol Sci 2022;23:10944. [Crossref] [PubMed]
- Seo Y, Ishizu T, Enomoto Y, Sugimori H, Yamamoto M, Machino T, Kawamura R, Aonuma K. Validation of 3-dimensional speckle tracking imaging to quantify regional myocardial deformation. Circ Cardiovasc Imaging 2009;2:451-9. [Crossref] [PubMed]
- Obokata M, Nagata Y, Wu VC, Kado Y, Kurabayashi M, Otsuji Y, Takeuchi M. Direct comparison of cardiac magnetic resonance feature tracking and 2D/3D echocardiography speckle tracking for evaluation of global left ventricular strain. Eur Heart J Cardiovasc Imaging 2016;17:525-32. [Crossref] [PubMed]
- Lee JH, Park JH. Strain Analysis of the Right Ventricle Using Two-dimensional Echocardiography. J Cardiovasc Imaging 2018;26:111-24. [Crossref] [PubMed]
- Nagueh SF, Smiseth OA, Appleton CP, Byrd BF 3rd, Dokainish H, Edvardsen T, Flachskampf FA, Gillebert TC, Klein AL, Lancellotti P, Marino P, Oh JK, Alexandru Popescu B, Waggoner AD. Houston, Texas; Oslo, Norway; Phoenix, Arizona; Nashville, Tennessee; Hamilton, Ontario, Canada; Uppsala, Sweden; Ghent and Liège, Belgium; Cleveland, Ohio; Novara, Italy; Rochester, Minnesota; Bucharest, Romania; and St. Recommendations for the Evaluation of Left Ventricular Diastolic Function by Echocardiography: An Update from the American Society of Echocardiography and the European Association of Cardiovascular Imaging. Eur Heart J Cardiovasc Imaging 2016;17:1321-60. [Crossref] [PubMed]
- Ng AC, Delgado V, Bertini M, van der Meer RW, Rijzewijk LJ, Shanks M, Nucifora G, Smit JW, Diamant M, Romijn JA, de Roos A, Leung DY, Lamb HJ, Bax JJ. Findings from left ventricular strain and strain rate imaging in asymptomatic patients with type 2 diabetes mellitus. Am J Cardiol 2009;104:1398-401. [Crossref] [PubMed]
- Cikes M, Solomon SD. Beyond ejection fraction: an integrative approach for assessment of cardiac structure and function in heart failure. Eur Heart J 2016;37:1642-50. [Crossref] [PubMed]
- Nemes A, Kormányos Á, Domsik P, Kalapos A, Gyenes N, Lengyel C, Valkusz Z. Diabetes mellitus deteriorates left ventricular deformation in acromegaly-analysis from the three-dimensional speckle-tracking echocardiographic MAGYAR-Path study. Quant Imaging Med Surg 2021;11:410-4. [Crossref] [PubMed]
- Dogdus M, Simsek E, Cinar CS. 3D-speckle tracking echocardiography for assessment of coronary artery disease severity in stable angina pectoris. Echocardiography 2019;36:320-7. [Crossref] [PubMed]
- Ghoreyshi-Hefzabad SM, Jeyaprakash P, Gupta A, Vo HQ, Pathan F, Negishi K. Three-Dimensional Global Left Ventricular Myocardial Strain Reduced in All Directions in Subclinical Diabetic Cardiomyopathy: A Systematic Review and Meta-Analysis. J Am Heart Assoc 2021;10:e020811. [Crossref] [PubMed]
- Galderisi M, Esposito R, Schiano-Lomoriello V, Santoro A, Ippolito R, Schiattarella P, Strazzullo P, de Simone G. Correlates of global area strain in native hypertensive patients: a three-dimensional speckle-tracking echocardiography study. Eur Heart J Cardiovasc Imaging 2012;13:730-8. [Crossref] [PubMed]
- Enzensberger C, Degenhardt J, Tenzer A, Doelle A, Axt-Fliedner R. First experience with three-dimensional speckle tracking (3D wall motion tracking) in fetal echocardiography. Ultraschall Med 2014;35:566-72. [Crossref] [PubMed]
- Huang BT, Yao HM, Huang H. Left ventricular remodeling and dysfunction in systemic lupus erythematosus: a three-dimensional speckle tracking study. Echocardiography 2014;31:1085-94. [Crossref] [PubMed]
- Yu HK, Xia B, Liu X, Han C, Chen W, Li Z. Initial application of three-dimensional speckle-tracking echocardiography to detect subclinical left ventricular dysfunction and stratify cardiomyopathy associated with Duchenne muscular dystrophy in children. Int J Cardiovasc Imaging 2019;35:67-76. [Crossref] [PubMed]
- Yu Z, Pan H, Cheng Z, Lu K, Hu H. Evaluation of Left Ventricular Systolic Function in Patients with Coronary Microvascular Dysfunction by Three-Dimensional Speckle-Tracking Imaging. Braz J Cardiovasc Surg 2022;37:321-7. [Crossref] [PubMed]
- Muraru D, Niero A, Rodriguez-Zanella H, Cherata D, Badano L. Three-dimensional speckle-tracking echocardiography: benefits and limitations of integrating myocardial mechanics with three-dimensional imaging. Cardiovasc Diagn Ther 2018;8:101-17. [Crossref] [PubMed]
- Yodwut C, Weinert L, Klas B, Lang RM, Mor-Avi V. Effects of frame rate on three-dimensional speckle-tracking-based measurements of myocardial deformation. J Am Soc Echocardiogr 2012;25:978-85. [Crossref] [PubMed]
- Wu VC, Takeuchi M, Otani K, Haruki N, Yoshitani H, Tamura M, Abe H, Lin FC, Otsuji Y. Effect of through-plane and twisting motion on left ventricular strain calculation: direct comparison between two-dimensional and three-dimensional speckle-tracking echocardiography. J Am Soc Echocardiogr 2013;26:1274-1281.e4. [Crossref] [PubMed]
- Dunlay SM, Givertz MM, Aguilar D, Allen LA, Chan M, Desai AS, Deswal A, Dickson VV, Kosiborod MN, Lekavich CL, McCoy RG, Mentz RJ. PiÑa IL; . Type 2 Diabetes Mellitus and Heart Failure, A Scientific Statement From the American Heart Association and Heart Failure Society of America. J Card Fail 2019;25:584-619. [Crossref] [PubMed]
- Aragno M, Mastrocola R, Medana C, Catalano MG, Vercellinatto I, Danni O, Boccuzzi G. Oxidative stress-dependent impairment of cardiac-specific transcription factors in experimental diabetes. Endocrinology 2006;147:5967-74. [Crossref] [PubMed]
- Gawlowski T, Stratmann B, Stork I, Engelbrecht B, Brodehl A, Niehaus K, Körfer R, Tschoepe D, Milting H. Heat shock protein 27 modification is increased in the human diabetic failing heart. Horm Metab Res 2009;41:594-9. [Crossref] [PubMed]
- Suto M, Tanaka H, Mochizuki Y, Mukai J, Takada H, Soga F, Dokuni K, Hatani Y, Hatazawa K, Matsuzoe H, Sano H, Shimoura H, Ooka J, Matsumoto K, Hirota Y, Ogawa W, Hirata KI. Impact of overweight on left ventricular function in type 2 diabetes mellitus. Cardiovasc Diabetol 2017;16:145. [Crossref] [PubMed]
- Haass M, Kitzman DW, Anand IS, Miller A, Zile MR, Massie BM, Carson PE. Body mass index and adverse cardiovascular outcomes in heart failure patients with preserved ejection fraction: results from the Irbesartan in Heart Failure with Preserved Ejection Fraction (I-PRESERVE) trial. Circ Heart Fail 2011;4:324-31. [Crossref] [PubMed]
- Ho JE, McCabe EL, Wang TJ, Larson MG, Levy D, Tsao C, Aragam J, Mitchell GF, Benjamin EJ, Vasan RS, Cheng S. Cardiometabolic Traits and Systolic Mechanics in the Community. Circ Heart Fail 2017;10:e003536. [Crossref] [PubMed]
- Mahajan R, Stokes M, Elliott A, Munawar DA, Khokhar KB, Thiyagarajah A, Hendriks J, Linz D, Gallagher C, Kaye D, Lau D, Sanders P. Complex interaction of obesity, intentional weight loss and heart failure: a systematic review and meta-analysis. Heart 2020;106:58-68. [Crossref] [PubMed]