Predicting severe proximal left anterior descending coronary artery stenosis using proximal left anterior descending coronary artery tortuosity and the angle between the left main and anterior descending coronary arteries: a retrospective cross-sectional study
Introduction
Atherosclerosis is a chronic disease that results in insufficient blood supply to the heart muscle, which can lead to angina or heart attacks (1). Metabolic problems are considered causative factors for atherosclerosis of blood vessels; however, structural factors are also considered important contributors to this disease. Notably, coronary bifurcation angles influence plaque initiation in the coronary artery (2).
Tortuosity in the coronary arteries causes changes in blood flow, reducing blood pressure distal to the tortuous portion of the coronary artery, and causing myocardial ischemia (3). People with coronary tortuosity often suffer from chest pain during exercise but usually resolves with rest (4). Xie et al. (5) performed a patient-specific numerical study to investigate the effect of coronary tortuosity on coronary hemodynamics. They showed that tortuosity in the coronary arteries reduces resting blood flow by 8% compared to the average resting blood flow, but blood flow is reduced by 14.9% during exercise. A wider angle between the left main coronary artery (LM) and left anterior descending coronary artery (LAD) has been associated with an increased risk of coronary artery disease (CAD) in the proximal LAD (6).
Coronary plaques are common in the LM near the bifurcation angle. Coronary plaques are formed by two major coronary branches with disturbed flow and low endothelial shear stress (7). Low shear stress is thought to be atherogenic (8). Increased bifurcation angle decreases wall shear stress, leading to plaque proliferation (7). Few studies have evaluated CAD through structural analysis of the proximal LAD. Specifically, no studies have simultaneously considered the roles of the coronary artery tortuosity and bifurcation angle in atherosclerosis. Either of the two factors (coronary artery tortuosity and bifurcation angle) does not independently affect the vessel wall. Both factors affect the vessel wall in combination. Therefore, we aimed to combine these two factors into one descriptor for evaluating proximal LAD disease. We present this article in accordance with the STROBE reporting checklist (available at https://qims.amegroups.com/article/view/10.21037/qims-23-518/rc).
Methods
Study design
This retrospective study was conducted in accordance with the Declaration of Helsinki (as revised in 2013) and approved by the Institutional Review Board of Changwon Hospital, Gyeongsang National University School of Medicine (No. GNUCH 2020-09-017-002), and the requirement for informed consent was waived due to the retrospective nature of the study. We reviewed the medical records of 133 consecutive patients who underwent computed tomography angiography (CTA) between November 2019 and January 2020.
Participants
Men and women aged ≥20 years who underwent CTA with angina symptoms between November 2019 and January 2020 were eligible, excluding patients who underwent CTA after previous coronary artery bypass surgery or percutaneous coronary intervention. The patients were divided into two groups according to the presence of significant LAD stenosis on CTA (defined as LAD stenosis >50%), as interpreted by radiologists.
The definition of variables
The LM-LAD angle (θ) was defined as the angle between the LM extension and LAD, and cosθ was defined as cosine value for LM-LAD angle. The coronary tortuosity index (CTI) was defined as the true length of the central luminal line divided by the straight distance. It was calculated from the LAD ostium to 20 mm away (Figure 1). d20 was defined as the linear distance between the endpoints of the 20 mm actual curve of the LAD, and d20*cosθ was defined as cosθ multiplied by d20.
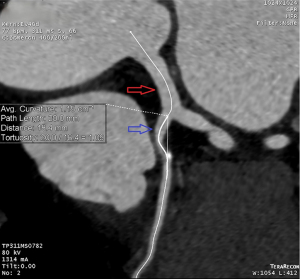
Data sources/measurement
Analysis and measurement of the coronary CTA (cCTA) scans were performed using commercially available post-processing software linked to the institutional picture archiving and communication system (PACS; version 4.4.11; Aquarius iNtuition TeraRecon, Foster City, CA, USA). Computed tomography (CT) scans were loaded and an independent review of the imaging data for each patient was performed by one investigator who received instructions on the standardized methods for the measurement of the degree of stenosis in the LAD and CTI of the proximal LAD. The straight length of the vessel was measured using the central luminal line of the flow path and was calculated using proprietary algorithms in TeraRecon software (TeraRecon). The angles between the LM and LAD were measured using both three-dimensional volume rendering and two-dimensional axial images (Figure 2).
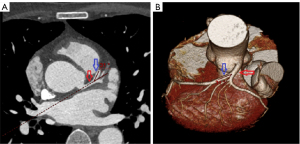
CTA protocol and image analysis
All cCTAs were performed using a third generation dual-source CT scanner (Siemens SOMATOM Force, Siemens Healthineers, Forchheim, Germany). At heart rates >60 bpm, intravenous beta blockers were administered prior to cCTA and, in the absence of contraindications, sublingual nitroglycerin (0.8 mg) was applied at all times. Iodinated contrast material (200 mL; Iomeron 400; Bracco Imaging S.p.A., Milan, Italy) was injected using a power injector at a flow rate of 5 mL/s, followed by a 50 mL saline chaser.
Statistical methods
Continuous variables were tested for normal distribution using the Kolmogorov-Smirnov test. Continuous variables were tested for normal distribution using the Kolmogorov-Smirnov test. Those with a normal distribution were expressed as means ± standard deviation (SD) and analyzed using the Student’s t-test. Those with a non-normal distribution were expressed as medians and analyzed using the Mann-Whitney U test. The chi-square test or Fisher’s exact test was used to compare categorical variables between groups. Binary logistic regression analysis was used to determine the independent predictors of significant proximal LAD stenosis. The odds ratio (OR) and its 95% confidence interval (CI) were calculated. Receiver operating characteristic (ROC) curve analyses of d20, cosθ, and d20*cosθ were performed to identify the optimal cut-off value for the prediction of significant proximal LAD stenosis by the Youden J statistic (9). Statistical analysis was performed using SPSS for Windows, version 21 (IBM Corporation, Chicago, IL, USA). A two-tailed P value <0.05 was considered significant.
Results
Participants
Baseline patient characteristics are shown in Table 1.
Table 1
Variables | LAD stenosis <50% (n=107) | LAD stenosis ≥50% (n=26) | P value |
---|---|---|---|
Age (years) | 59.7±12.8 | 66.3±10.8 | 0.017 |
Male | 58 (54.2) | 18 (69.2) | 0.243 |
Height (cm) | 163.3±9.2 | 163.8±7.8 | 0.794 |
Body mass index (kg/m2) | 24.6±4.0 | 24.5±6.3 | 0.948 |
Hypertension | 64 (59.8) | 22 (84.6) | 0.032 |
Dyslipidemia | 69 (64.5) | 18 (69.2) | 0.821 |
Chronic kidney disease | 4 (3.7) | 2 (7.7) | 0.730 |
Diabetes | 28 (26.2) | 13 (50.0) | 0.034 |
Pack years | 7.6±14.2 | 20.4±22.8 | 0.011 |
Family history | 7 (6.5) | 3 (11.5) | 0.651 |
Data are presented as mean ± SD or n (%). LAD, left anterior descending coronary artery; SD, standard deviation.
Outcome data
Proximal LAD stenosis of >50% was seen in 26 of the 133 patients. The two groups differed significantly in the age, presence of hypertension or, diabetes, and number of pack years (P=0.017, P=0.032, P=0.034, and P=0.011, respectively); however, there were no other significant differences. The mean LM-LAD angles of patients with <50% and >50% proximal LAD stenosis were 29.2° and 38.3°, respectively. Table 2 shows that the LM-LAD angles and cosθ differed significantly between the two groups (P<0.001 for both). There was no statistically significant difference in the linear distances between the d20 endpoints between the two groups (P=0.104); however, there was a statistically significant difference in d20*cosθ (P<0.001). Among the patients with >50% proximal LAD stenosis, 65.4% and 73.1% of patients had a d20 <19 mm and cosθ <0.8, respectively.
Table 2
Variables | LAD stenosis <50% (n=107) |
LAD stenosis ≥50% (n=26) | P value |
---|---|---|---|
LM-LAD angle (°) | 29.2±10.9 | 38.3±8.7 | <0.001 |
cosθ | 0.9±0.1 | 0.8±0.1 | <0.001 |
d20 (mm) | 19.0±0.8 | 18.7±0.7 | 0.104 |
d20*cosθ | 16.3±2.0 | 14.5±1.8 | <0.001 |
d20 <19 mm | 44 (41.1) | 17 (65.4) | 0.045 |
cosθ <0.8 | 27 (25.2) | 19 (73.1) | <0.001 |
Data are presented as mean ± SD or n (%). CTA, computed tomography angiography; LAD, left anterior descending coronary artery; LM, left main coronary artery; cosθ, cosine value for LM-LAD angle; d20, the linear distance between the endpoints of the 20 mm actual curve of the LAD; d20*cosθ, cosθ multiplied by d20; SD, standard deviation.
The areas under the ROC curves for d20, cosθ, and d20*cosθ were 0.634, 0.743, and 0.767, respectively. The cut-off values of 19 mm, 0.8, and 15.5 for d20, cosθ, and d20*cosθ, respectively, had sensitivities and specificities of 65.4 and 58.5, 73.1 and 74.8, and 80.8 and 71.0, respectively (Figure 3).
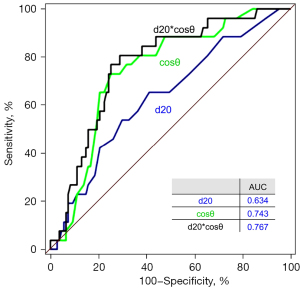
In the univariate analysis (Table 3), there were significant differences in the d20, cosθ <0.8, age, presence of hypertension or diabetes, and number of pack years [hazard ratio (HR): 2.70, 8.04, 1.05, 3.70, 2.82, and 1.04; 95% CI: 1.13–6.87, 3.17–22.57, 1.01–1.09, 1.30–13.30, 1.16–6.88, and 1.01–1.06; P=0.029, P<0.001, P=0.020, P=0.024, P=0.021, and P=0.002, respectively). However, in the multivariate analysis (Table 4), the d20*cosθ <15.5, presence of hypertension, and number of pack years (HR: 11.36, 4.54, and 1.04; 95% CI: 3.9–39.54, 1.39–18.32, and 1.01–1.07; and P<0.001, P=0.019, and P=0.003, respectively) were predictors of significant proximal LAD stenosis.
Table 3
Variables | Univariate OR (95% CI) | P value |
---|---|---|
d20 <19 mm | 2.70 (1.13–6.87) | 0.029 |
cosθ <0.8 | 8.04 (3.17–22.57) | <0.000 |
Age (years) | 1.05 (1.01–1.09) | 0.020 |
Male | 1.90 (0.78–4.98) | 0.169 |
Body mass index (kg/m2) | 1.00 (0.90–1.09) | 0.931 |
Hypertension | 3.70 (1.30–13.30) | 0.024 |
Dyslipidemia | 1.24 (0.51–3.26) | 0.649 |
Chronic kidney disease | 2.15 (0.29–11.67) | 0.394 |
Diabetes | 2.82 (1.16–6.88) | 0.021 |
Pack years | 1.04 (1.01–1.06) | 0.002 |
Family history | 1.86 (0.38–7.28) | 0.393 |
Data are presented as mean ± SD or n (%). LAD, left anterior descending coronary artery; OR, odds ratio; CI, confidence interval; d20, the linear distance between the endpoints of the 20 mm actual curve of the LAD; cosθ, cosine value for LM-LAD angle; LM, left main coronary artery; SD, standard deviation.
Table 4
Variables | Multivariate OR (95% CI) | P value |
---|---|---|
d20*cosθ <15.5 | 11.36 (3.9–39.54) | <0.001 |
Hypertension | 4.54 (1.39–18.32) | 0.019 |
Pack years | 1.04 (1.01–1.07) | 0.003 |
Data are presented as mean ± SD or n (%). LAD, left anterior descending coronary artery; OR, odds ratio; CI, confidence interval; d20*cosθ, cosθ multiplied by d20; cosθ, cosine value for LM-LAD angle; LM, left main coronary artery; d20, the linear distance between the endpoints of the 20 mm actual curve of the LAD; SD, standard deviation.
Discussion
Previous studies have mostly investigated either coronary bifurcation angle or vessel tortuosity, expressing the risk for coronary disease as a single factor. In reality, blood vessels have multiple branches that form the bifurcation angle and are particularly bendy in a three-dimensional structure. Therefore, the effects of both factors (bifurcation angle and vessel tortuosity) cannot be considered separately when studying vascular disease.
In the present report, we provided the importance of the combined factors (d20*cosθ) of the LM-LAD angle and the LAD tortuosity when considering risk or predicting factors of significant proximal LAD stenosis. We found that these two factors were independently associated with a higher risk of significant proximal LAD stenosis, even after adjusting for age, hypertension, diabetes, pack years, and other covariates. These findings suggest that the structural analysis of the patient’s coronary artery is necessary to explore and identify the cause of CAD, and is also important for patient management.
We previously performed a study which found that the LM-LAD angle was, statistically, a more significant factor in the formation of the LAD stenotic lesions than the LAD- left circumflex coronary artery (LCX) angle (8). Malvè et al. reported that the LM-LAD angle is a better predictor of low shear stress than the LM-LCX angle (10). In addition, coronary artery tortuosity leads to a variation in blood flow and affects the local wall shear stress (11). The tortuosity index (TI) is the distance factor defined as TI = (L/D) − 1: where L is the vessel length, and D is the straight line distance between its end point (12).
Both the coronary artery bifurcation angles and tortuosity change the shear stress in blood vessels. In general, low shear stress is a causative factor for atherosclerosis (13-16). To our knowledge, there has been little research into the structural analysis of proximal LAD lesions. In particular, few studies have simultaneously analyzed the tortuosity and bifurcation angle.
The wall shear stress (τ) can be expressed as
where η is the apparent blood viscosity (0.035 poise), Q is the rate of blood flow through the vessel, and r is the vessel radius. The shear stress increases when the blood flow increases or blood vessel diameter decreases, and the shear stress decreases as the blood flow decreases (17).
At wider coronary artery bifurcation angles, regions of low shear stress form in the areas of bifurcation (18,19), and the fluid shear stress gradients (flow acceleration and deceleration) that are created in tortuous vessels have been shown to initiate platelet aggravation (20).
The proximal LAD forms a bifurcation angle, branches at the LM, and has tortuosity. Its blood flow is influenced by two factors, bifurcation angle and tortuosity. In other words, as the proximal LAD bifurcation angle increases, regions of low shear stress occur. Subsequently, regions of high and low shear stress occur on the bent outer and inner sides, respectively, resulting in shear gradients.
Using this rationale, we described the bifurcation angle and tortuosity as one (d20*cosθ). The vessel length was fixed at 20 mm, thus the d20 was the only variable that was used to represent tortuosity. This equation came not from a physical or mathematical calculation. Both factors have close effects on the shear stress, and the resulting effects on the vessel could also be considered closely related. Thus, it was expressed as a product of two factors and the results were statistically significant (Table 4). In particular, when d20*cosθ <15.5, the risk of proximal LAD lesion formation was high. To satisfy the inequality of d20*cosθ <15.5, d20 should be <19.94 mm when θ=39°. However, when θ>40°, d20 should be <20.23 mm. Since d20 is always <20 mm, all d20 values always satisfy the inequality at angles above 40°.
When d20 is 20 mm, that is, when there is no bending, the LM-LAD angle must be >39° to satisfy the inequality. In summary, when there is no bending, the risk of proximal LAD lesion formation occurs at an LM-LAD angle >39°. In other words, if the LM-LAD angle is <39°, the risk of proximal LAD lesion formation is affected by both the d20 and the LM-LAD angle. If it is > 40°, the risk of proximal LAD lesions is affected by the angle rather than the tortuosity. And in the absence of tortuosity of LAD, risk of proximal LAD lesions are affected when the LM-LAD angle is >40°. In the study that we performed previously (3), we found that an LM-LAD angle >40° was a predictor for significant LAD stenosis.
Limitation
This was a single center, retrospective study, and the sample size of the patients with significant LAD stenosis was small. Thus, a larger, multi-center, prospective study is needed. The findings of this study have limited generalizability due to the complex anatomy of the LAD. The LAD does not bend in a single, consistent location, and there are several changes in the blood vessel along its course. In future, we plan to repeat our measurements, including measurements divided by location, i.e., the mid and distal LAD, to find a generalizable formula that may apply to the entire LAD. Further, it is clinically important to study the cCTA/Fractional Flow Reserve to correlate the LM-LAD angle and TI where hemodynamically significant proximal LAD stenosis are seen.
Conclusions
As the tortuosity and LM-LAD angle increased (d20 and cosθ decreased, respectively), the shear stress gradient on the inner wall of the vessel increased, which increased the chance of proximal LAD lesion formation. Thus, we propose that d20*cosθ might be useful as a predictor of proximal LAD stenosis.
Acknowledgments
The authors are grateful to Byeong Yeol Ahn and Tae Hee Kim from Changwon Science High School for their physical and mathematical opinions.
Funding: None.
Footnote
Reporting Checklist: The authors have completed the STROBE reporting checklist. Available at https://qims.amegroups.com/article/view/10.21037/qims-23-518/rc
Conflicts of Interest: All authors have completed the ICMJE uniform disclosure form (available at https://qims.amegroups.com/article/view/10.21037/qims-23-518/coif). The authors have no conflicts of interest to declare.
Ethical Statement: The authors are accountable for all aspects of the work in ensuring that questions related to the accuracy or integrity of any part of the work are appropriately investigated and resolved. This retrospective study was conducted in accordance with the Declaration of Helsinki (as revised in 2013) and approved by the Institutional Review Board of Changwon Hospital, Gyeongsang National University School of Medicine (No. GNUCH 2020-09-017-002), and the requirement for informed consent was waived due to the retrospective nature of the study.
Open Access Statement: This is an Open Access article distributed in accordance with the Creative Commons Attribution-NonCommercial-NoDerivs 4.0 International License (CC BY-NC-ND 4.0), which permits the non-commercial replication and distribution of the article with the strict proviso that no changes or edits are made and the original work is properly cited (including links to both the formal publication through the relevant DOI and the license). See: https://creativecommons.org/licenses/by-nc-nd/4.0/.
References
- Buradi A, Mahalingam A. Impact of coronary tortuosity on the artery hemodynamics. Biocybern Biomed Eng 2020;40:126-47.
- Morbiducci U, Kok AM, Kwak BR, Stone PH, Steinman DA, Wentzel JJ. Atherosclerosis at arterial bifurcations: evidence for the role of haemodynamics and geometry. Thromb Haemost 2016;115:484-92. [Crossref] [PubMed]
- Gupta A, Panda P, Sharma YP, Mahesh A, Sharma P, Mahesh NK. Clinical profile of patients with coronary tortuosity and its relation with coronary artery disease. Int J Cardiol Cardiovasc Res 2018;4:66-71.
- Gaibazzi N, Rigo F, Reverberi C. Severe coronary tortuosity or myocardial bridging in patients with chest pain, normal coronary arteries, and reversible myocardial perfusion defects. Am J Cardiol 2011;108:973-8. [Crossref] [PubMed]
- Xie X, Wang Y, Zhu H, Zhou H, Zhou J. Impact of coronary tortuosity on coronary blood supply: a patient-specific study. PLoS One 2013;8:e64564. [Crossref] [PubMed]
- Yahia M, Farid W, Lotfy M, Osama M, El Deep HA. Association between bifurcation angle of the left main coronary artery and severity of stenosis of the proximal left anterior descending artery. Cardiovasc J Afr 2023;34:93-7. [Crossref] [PubMed]
- Wentzel JJ, Chatzizisis YS, Gijsen FJ, Giannoglou GD, Feldman CL, Stone PH. Endothelial shear stress in the evolution of coronary atherosclerotic plaque and vascular remodelling: current understanding and remaining questions. Cardiovasc Res 2012;96:234-43. [Crossref] [PubMed]
- Moon SH, Byun JH, Kim JW, Kim SH, Kim KN, Jung JJ, Kang DH, Yang JH, Choi JY, Jang IS, Park HO, Lee CE, Ahn JH. Clinical usefulness of the angle between left main coronary artery and left anterior descending coronary artery for the evaluation of obstructive coronary artery disease. PLoS One 2018;13:e0202249. [Crossref] [PubMed]
- Hajian-Tilaki K. Receiver Operating Characteristic (ROC) Curve Analysis for Medical Diagnostic Test Evaluation. Caspian J Intern Med 2013;4:627-35.
- Malvè M, Gharib AM, Yazdani SK, Finet G, Martínez MA, Pettigrew R, Ohayon J. Tortuosity of coronary bifurcation as a potential local risk factor for atherosclerosis: CFD steady state study based on in vivo dynamic CT measurements. Ann Biomed Eng 2015;43:82-93. [Crossref] [PubMed]
- Li Y, Liu NF, Gu ZZ, Chen Y, Lu J, Feng Y, Ma GS, Shen CX. Coronary tortuosity is associated with reversible myocardial perfusion defects in patients without coronary artery disease. Chin Med J (Engl) 2012;125:3581-3.
- Dougherty G, Varro J. A quantitative index for the measurement of the tortuosity of blood vessels. Med Eng Phys 2000;22:567-74. [Crossref] [PubMed]
- Malek AM, Alper SL, Izumo S. Hemodynamic shear stress and its role in atherosclerosis. JAMA 1999;282:2035-42. [Crossref] [PubMed]
- Han SH, Puma J, García-García HM, Nasu K, Margolis P, Leon MB, Lerman A. Tissue characterisation of atherosclerotic plaque in coronary artery bifurcations: an intravascular ultrasound radiofrequency data analysis in humans. EuroIntervention 2010;6:313-20. [Crossref] [PubMed]
- Gijsen FJ, Wentzel JJ, Thury A, Lamers B, Schuurbiers JC, Serruys PW, van der Steen AF. A new imaging technique to study 3-D plaque and shear stress distribution in human coronary artery bifurcations in vivo. J Biomech 2007;40:2349-57. [Crossref] [PubMed]
- Cecchi E, Giglioli C, Valente S, Lazzeri C, Gensini GF, Abbate R, Mannini L. Role of hemodynamic shear stress in cardiovascular disease. Atherosclerosis 2011;214:249-56. [Crossref] [PubMed]
- Behnke BJ, Prisby RD, Lesniewski LA, Donato AJ, Olin HM, Delp MD. Influence of ageing and physical activity on vascular morphology in rat skeletal muscle. J Physiol 2006;575:617-26. [Crossref] [PubMed]
- Juan YH, Tsay PK, Shen WC, Yeh CS, Wen MS, Wan YL. Comparison of the Left Main Coronary Bifurcating Angle among Patients with Normal, Non-significantly and Significantly Stenosed Left Coronary Arteries. Sci Rep 2017;7:1515. [Crossref] [PubMed]
- Sun Z, Cao Y. Multislice CT angiography assessment of left coronary artery: correlation between bifurcation angle and dimensions and development of coronary artery disease. Eur J Radiol 2011;79:e90-5. [Crossref] [PubMed]
- Luna DJ, R, Pandian NK, Mathur T, Bui J, Gadangi P, Kostousov VV, Hui SR, Teruya J, Jain A. Tortuosity-powered microfluidic device for assessment of thrombosis and antithrombotic therapy in whole blood. Sci Rep 2020;10:5742. [Crossref] [PubMed]