Territorial arterial spin labeling perfusion imaging in a patient with hyperplastic anterior choroidal artery: a case description
Introduction
Hyperplastic anterior choroidal artery (AchA) is a rare cerebrovascular variant, with a reported prevalence of approximately 2.3% in digital subtraction angiography (DSA) and 0.55% in magnetic resonance angiography (MRA) (1,2). The variant may change cerebral artery perfusion territories, thereby impacting interpretation of the affected vessel in patients with ischemic stroke and the surgical approach of intracranial pathology. Previous studies have reported this variation being detected with computed tomography angiography (CTA), MRA, or DSA (2-8). However, CTA and MRA can only show the morphology of the hyperplastic AchA. Meanwhile, although DSA can show the blood supply region of the hyperplastic AchA, it is invasive, radiative, and incapable of providing perfusion information. Territorial arterial spin labeling (t-ASL) perfusion imaging can map perfusion territories of individual artery without the use of a contrast agent (9-11). The t-ASL perfusion imaging mainly includes 2 methods: vessel-encoded pseudo-continuous ASL (VE-pCASL) and superselective pseudocontinuous ASL (SS-pCASL). The ability of SS-pCASL to accurately determine the perfusion territory of a single vessel is better than that of VE-pCASL (12), and image postprocessing of SS-pCASL is relatively simple. Here, we describe a case of hyperplastic AchA and demonstrate its effect on cerebral blood flow distribution with t-ASL perfusion imaging. To our knowledge, there have been no published reports on t-ASL perfusion imaging findings of hyperplastic AchA.
Case presentation
A 48-year-old male presented with sudden onset vertigo. He had a history of smoking, and his triglyceride level was 3.69 mmol/L. No abnormality in the brain parenchyma appeared on magnetic resonance imaging (MRI). Three-dimensional time-of-flight MRA (3D-TOF-MRA) showed a prominent artery coursing almost parallel to the left posterior cerebral artery (PCA), which originated from the posterior aspect of the supraclinoid portion of the left internal carotid artery (ICA). Bilateral posterior communicating arteries (PComAs) could not be identified. Based on its origin and course, this vessel was originally thought to be a hyperplastic AchA (Figure 1A,1B). 3D ASL perfusion MRI indicated that bilateral whole-brain perfusion was basically symmetrical (Figure 2A). We used the SS-pCASL technique for territorial perfusion imaging, which was performed with a 3.0 T MR imaging scanner (Discovery MR750w, GE Healthcare, Chicago, IL, USA) and a 32-channel receive-only head coil. In general, for maximum labeling efficiency, straight-lined arterial segments should be considered for labeling. At the skull base, the plane on which the course of vertebral artery (VA) and ICA were relatively straight was selected as the labeling plane. Then, by directly placing the label spot at the center of the target vessel, we could obtain the single-vessel perfusion territories. The imaging parameters were as follows: postlabeling delay =1,525 ms, field of view =220×220 mm, slice thickness =4 mm, repetition time (TR) =4,598 ms, time to echo (TE) = 10.7 ms, points =512, arms =4, and number of excitations (NEX) =2. t-ASL perfusion MRI can selectively show the perfusion territories of the right and left ICAs and the right and left VAs. With this case, the bilateral ICAs supplied the bilateral frontal lobes, insula lobes, and lateral temporal lobes. However, due to the presence of the hyperplastic AchA, the left ICA also supplied the left medial temporal region (Figure 2B,2C). Bilateral VAs supplied the bilateral cerebellar hemispheres, occipital lobes, and thalamus. Because the left VA diameter was significantly larger than the right (Figure 1B), the blood supply area of the left VA was significantly larger than that of the right. In addition, the left VA also supplied the right posterior medial temporal region. However, the left medial temporal region had no supply from the left VA (Figure 2D,2E). Due to the history of smoking and hypertriglyceridemia (triglyceride level was 3.69 mmol/L), the patient was administered statins to decrease serum lipids and gastrodin to improve vascular circulation. Finally, with the remission of symptoms, the patient was discharged. All procedures performed in this study were conducted in accordance with the ethical standards of the institutional and/or national research committee(s) and with the Declaration of Helsinki (as revised in 2013). Written informed consent was obtained from the patient for publication of this case report and accompanying images. A copy of the written consent is available for review by the editorial office of this journal.
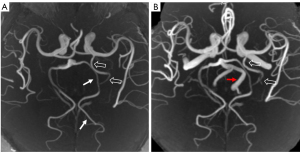
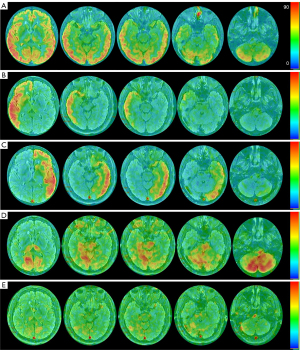
Discussion
Usually, AchA originates at the end of the internal carotid artery and is distant from the PComAs. It is divided into the cisternal segment and intraventricular segment. The cisternal segment extends from its origin until the choroidal fissure coursing inside the ambient cistern. After it enters the choroidal fissure, it is referred to as the intraventricular segment. Regardless of the variation, its general course is toward the lateral ventricular choroid plexus. At the fifth week of embryonic development, the vertebrobasilar system has not yet formed, and the carotid artery supplies almost the entire brain. At this time, AchA is the largest branch of the ICA, giving branches to the basal diencephalon as well as to the posteromedial part of the hemisphere. Usually, as the hemispheres grow, the contribution of AchA regresses as that of the PCA expands. However, a variant is occasionally encountered in which the AchA supplies the territories typically served by the PCA. The hyperplastic AchA can partially or completely replace PCA territories (13). Takahashi et al. (2) classified hyperplastic AchA into 4 subtypes based on the supply to the PCA distribution region: type 1 involves the anomalous AchA supplying the distribution area of the anterior temporal branch of the PCA, type 2 involves the anomalous AchA supplying most of the distribution area of the anterior and posterior temporal branches of the PCA, type 3 involves the anomalous AchA supplying the distribution area of the calcarine and occipitoparietal arteries, and type 4 involves the original PCA being almost totally replaced by the anomalous AchA. The case we encountered was type 2. Thus far, no study has directly reported the cerebral blood flow distribution of hyperplastic AchA captured via t-ASL perfusion imaging.
In this case, the left hyperplastic AchA supplied the left medial temporal region, and this was visually demonstrated on t-ASL perfusion maps. When there is vascular variation, whole-brain perfusion imaging (such as CT perfusion imaging and 3D-ASL perfusion imaging) cannot truly reflect the blood flow distribution of the variant vessels. At present, DSA and t-ASL are the main methods for visualizing the brain artery territories of single vessel in vivo. DSA is invasive, includes ionizing radiation, and requires the injection of contrast agent. Moreover, it shows the distribution of the cerebral vascular tree rather than the true cerebral blood perfusion, so it cannot quantify the cerebral blood flow. However, t-ASL has the advantages of not requiring contrast agents and nonionizing radiation. It reflects the true cerebral blood perfusion based on the free diffusion of water molecules, and the perfusion volume can be quantified. In ischemic stroke survivors with a vascular variant, t-ASL perfusion imaging helps the clinician to understand the influence of the vascular variant on the distribution pattern of the ischemic infarction, so as to aid in determining the true affected vessel (14).
In conclusion, AchA hyperplasia is a rare variant that affects cerebral blood flow distribution. t-ASL perfusion imaging can intuitively show the effect of this variant on cerebral blood flow distribution.
Acknowledgments
Funding: None.
Footnote
Conflicts of Interest: All authors have completed the ICMJE uniform disclosure form (available at https://qims.amegroups.com/article/view/10.21037/qims-23-269/coif). The authors have no conflicts of interest to declare.
Ethical Statement:
Open Access Statement: This is an Open Access article distributed in accordance with the Creative Commons Attribution-NonCommercial-NoDerivs 4.0 International License (CC BY-NC-ND 4.0), which permits the non-commercial replication and distribution of the article with the strict proviso that no changes or edits are made and the original work is properly cited (including links to both the formal publication through the relevant DOI and the license). See: https://creativecommons.org/licenses/by-nc-nd/4.0/.
References
- Uchino A, Saito N, Takahashi M, et al. Variations of the posterior cerebral artery diagnosed by MR angiography at 3 tesla. Neuroradiology 2016;58:141-6. [Crossref] [PubMed]
- Takahashi S, Suga T, Kawata Y, et al. Anterior choroidal artery: angiographic analysis of variations and anomalies. AJNR Am J Neuroradiol 1990;11:719-29.
- Shah AU, Joshi AR, Rai PR, et al. Hyperplastic Anterior Choroidal Artery-A Rare Variant Detected on MR Angiography. Indian J Radiol Imaging 2022;32:266-9. [Crossref] [PubMed]
- Hahm MH, Moon S. Anomalous hyperplastic anterior choroidal artery and its mimicker: a case series. Surg Radiol Anat 2021;43:1961-5. [Crossref] [PubMed]
- Das D, Kumar R, Dutta A. Hypertrophic anterior choroidal artery: images and brief embryological overview. BMJ Case Rep 2021;14:e240328. [Crossref] [PubMed]
- Coulier B. Duplication of the Posterior Cerebral Artery (PCA) or "True Fetal PCA": An Extremely Rare Variant. J Belg Soc Radiol 2018;102:29. [Crossref] [PubMed]
- Lambert SL, Williams FJ, Oganisyan ZZ, et al. Fetal-Type Variants of the Posterior Cerebral Artery and Concurrent Infarction in the Major Arterial Territories of the Cerebral Hemisphere. J Investig Med High Impact Case Rep 2016;4:2324709616665409. [Crossref] [PubMed]
- Antonietti LC, Glastonbury CM, Adler F, et al. Hyperplastic anterior choroidal artery identified using magnetic resonance angiography: a report of two cases. Cerebrovasc Dis 2006;22:450-2. [Crossref] [PubMed]
- Bokkers RP, De Cocker LJ, van Osch MJ, et al. Selective Arterial Spin Labeling: Techniques and Neurovascular Applications. Top Magn Reson Imaging 2016;25:73-80. [Crossref] [PubMed]
- Helle M, Norris DG, Rüfer S, et al. Superselective pseudocontinuous arterial spin labeling. Magn Reson Med 2010;64:777-86. [Crossref] [PubMed]
- Wong EC. Vessel-encoded arterial spin-labeling using pseudocontinuous tagging. Magn Reson Med 2007;58:1086-91. [Crossref] [PubMed]
- Hartkamp NS, Helle M, Chappell MA, et al. Validation of planning-free vessel-encoded pseudo-continuous arterial spin labeling MR imaging as territorial-ASL strategy by comparison to super-selective p-CASL MRI. Magn Reson Med 2014;71:2059-70. [Crossref] [PubMed]
- Raybaud C. Normal and abnormal embryology and development of the intracranial vascular system. Neurosurg Clin N Am 2010;21:399-426. [Crossref] [PubMed]
- Hartkamp NS, Hendrikse J, De Cocker LJ, et al. Misinterpretation of ischaemic infarct location in relationship to the cerebrovascular territories. J Neurol Neurosurg Psychiatry 2016;87:1084-90. [Crossref] [PubMed]