Contrast-enhanced ultrasonography for identifying acute kidney injury in brain-dead donors
Introduction
Kidney transplantation is the best treatment option for end-stage renal disease and offers improved quality of life and survival of patients compared with dialysis treatment. At present, transplantation of organs from deceased donors remains the most prevalent form of transplantation, with brain-dead individuals being the main source of donations (1,2). After brain death, many organs and systems in the body undergo a series of dysfunctions and physiological changes that can affect the quality and function of donated organs (3). Acute kidney injury (AKI) is a syndrome characterized by the rapid loss of renal excretory function (4) and is frequently found in deceased donors. Although donor kidneys with AKI are more likely to undergo delayed graft function (5,6), AKI has a limited impact on graft success and long-term outcomes, thereby providing a safe and acceptable source of organs (7-9).
AKI in living individuals is usually diagnosed by a rapid increase in nitrogen metabolism end products (serum creatinine), decrease in urine output, or both (4). Thus far, few studies have used imaging to detect and identify AKI in deceased donors. Scintigraphy, positron-emission tomography, and various magnetic resonance imaging–based techniques offer promise for detecting renal perfusion in donors with AKI; however, high radiation exposure, nephrotoxicity, inability to perform at bedside, and safety concerns during transport and examination have limited the widespread use of these techniques (10,11). Contrast-enhanced ultrasonography (CEUS) is an emerging imaging technique that uses microbubbles to enhance tissue perfusion detection at the microvascular level. As a noninvasive, radiation-free, and easy-to-operate examination technique, CEUS is a valuable tool for monitoring and predicting acute complications after transplantation (12,13). The change of renal perfusion, especially at the microvascular level, appears to play a key role in the development of AKI (14). CEUS allows for the evaluation of renal microcirculation qualitatively and quantitatively, which is highly useful for detecting the condition change and prognosis of patients with AKI (15,16). Reliable pretransplant assessment of organ quality is essential, and the renal function of the donor organ can affect the short- and long-term outcomes of recipients. Considering that CEUS is non-nephrotoxic and repeatable at bedside, which avoids medically induced injury to the organs of deceased donors, we hypothesized that CEUS can accurately assess renal perfusion and function in brain-dead donors.
The purpose of this study was to detect renal microcirculatory perfusion in brain-dead donors using CEUS, to investigate the value of CEUS in identifying AKI, and to analyze the correlation between CEUS and preimplantation biopsy results of donor kidneys and early post-transplant renal function of grafts. We present this article in accordance with the STARD reporting checklist (available at https://qims.amegroups.com/article/view/10.21037/qims-23-207/rc).
Methods
Study design and sample
In this prospective study, kidney donors maintained in our organ procurement organization (OPO) from August 2020 to November 2022 were recruited (Figure 1). The inclusion criteria were age ≥18 years and brain death. The exclusion criteria encompassed donors maintained with extracorporeal membrane oxygenation (ECMO) and the presence of irregular kidney anatomy. All donors underwent CEUS within 24 hours prior to organ procurement, with a total of 100 donor kidneys examined. Six kidneys were excluded (four maintained with ECMO, one with renal hilar ectropion malformation, and one with image loss). A total of 94 donor kidneys were finally included in this study. The remaining patients were divided into the AKI (n=44) and non-AKI (n=50) groups based on the Kidney Disease Improving Global Outcome (KDIGO) criteria (4). The mean age of the donors was 45.1±10.4 [standard deviation (SD)] years, and the majority were male (86.2%).
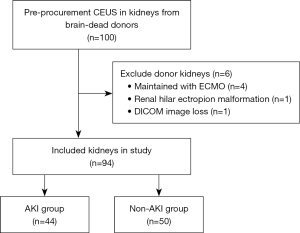
Ethical considerations
This prospective study was approved by the Ethics Committee of Zhongshan City People’s Hospital (No. K2019-058) and complied with the Declaration of Helsinki (as revised in 2013). The donors’ families were informed of the renal biopsy and CEUS procedure and possible risks 24 hours prior to the examination, and they provided signed informed consent. All recipients who received kidney donations also provided signed informed consent.
Ultrasound examinations
All donors were examined within 24 hours prior to organ procurement. Each donor was placed in a supine position for ultrasound examinations, and the examinations were performed using a Philips ultrasound machine (EPIQ5, Philips, Amsterdam, The Netherlands) and a convex transducer C5-1 ultrasound probe. All ultrasound images were analyzed independently by two radiologists, each with more than 10 years of experience in CEUS (YGX and XZL). Routine ultrasound and color Doppler examination were performed by same radiologists before CEUS. This included renal size, peak systolic velocity, end-diastolic velocity, and resistive index (RI) of the kidney artery. The primary gain, machine index, focus position, temporal gain compensation, and other preset values were kept constant while CEUS was being performed. The longitudinal section of the kidney was chosen as the fixed section for CEUS. After preparation of the contrast agent (SonoVue, Bracco Imaging B.V., Milan, Italy), 2.4 mL of the agent was pushed through a new cephalic vein, and 5 mL of saline was used to flush the tube after each push. Following the injection of SonoVue, the donors were disconnected from the ventilator (45–60 seconds) to eliminate the impact of breathing, and a 3-minute video of CEUS imaging was recorded in Digital Imaging and Communications in Medicine (DICOM) format for subsequent analysis. The whole process was assisted by intensive care unit (ICU) doctors and nurses. CEUS was first performed on the right kidney and then the left kidney with an interval of 30 minutes.
Time-intensity analysis
Time-intensity curve (TIC) analysis was performed using an offline personal computer and an image analysis software program (ImageJ; National Institutes of Health, Bethesda, MD, USA). First, the images saved in DICOM format were decompressed into uncompressed Audio Video Interleave (AVI) files. Contrast images were viewed frame by frame using ImageJ software, and the time of the first echogenic microbubble observed in the kidney segmental artery (KA) was set as the initial time. A circular region of interest (ROI) with a diameter of 5 mm was created within the KA, kidney cortex (KC), and kidney medulla (KM). All ROIs were drawn at a central location (i.e., the middle third of the kidney). Intensity values were measured automatically using ImageJ, with each pixel having an intensity value between a minimum of 0 and a maximum of 255. After the intensity values of each ROI were measured, a TIC with a time of 20 seconds was created using Excel software (Microsoft, Redmond, WA, USA), starting from the arrival of the contrast agent at KA, and the arrival time (AT) was recorded. Time to peak (TTP) and peak intensity (PI) were evaluated according to TIC (Figure 2). The time interval (TI) values between the TTP of KA and KC [TI(KA-KC)], KA and KM [TI(KA-KM)], and KC and KM [TI(KC-KM)] were then calculated. All TIC parameters were measured 2 times for each patient to investigate the intraobserver variability. Furthermore, the TIC parameters of all patients were successively evaluated by two radiologists for analysis of interobserver variability.
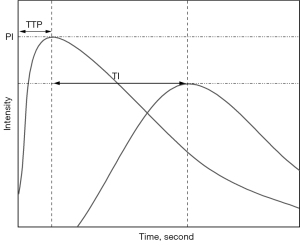
Biopsy analysis
Preimplantation biopsy and histological examination were performed on all kidneys, and the site of biopsy was the upper pole of the kidney. All biopsies were read and graded by trained nephropathologists using the Banff 2017 Preimplantation Kidney Biopsy guidelines, with the number of glomeruli, number of globally sclerosed glomeruli, percentage of global glomerulosclerosis, and the severity of interstitial fibrosis, tubular atrophy, interstitial inflammation, arterial intimal fibrosis, arteriolar hyalinosis, glomerular thrombi, and acute tubular injury/necrosis being reported (17). For grading based on the assessment results (none, mild, moderate, severe), a 4-point scale (0, 1, 2, 3) was used, with evaluation completed for interstitial inflammation; glomerular thrombi; acute tubular injury among acute lesions; and tubular atrophy, interstitial fibrosis, arterial intimal fibrosis, and arteriolar hyalinosis among chronic lesions. The sum of scores for individual variables yielded the composite scores, including the chronic Banff score (tubular atrophy, interstitial fibrosis, arterial intimal fibrosis, and arteriolar hyalinosis), acute Banff score (interstitial inflammation, glomerular thrombi, and acute tubular injury), and total Banff score.
Renal function assessment
Renal function was assessed using commonly used clinical indicators of renal function. Serum creatinine, 24-hour urine volume, and estimated glomerular filtration rate (eGFR) were collected from the hospital’s clinical laboratory system and mobile care system for donors and transplant recipients, and eGFR was calculated according to the Chronic Kidney Disease Epidemiology Collaborative (CKD-EPI) equation (18).
Statistical analysis
Numerical data are expressed as the mean and standard deviation or median with 25th–75th percentiles depending on the distribution of variables, and Student t-test or Mann-Whitney test was used to analyze the differences in clinical characteristics and CEUS parameters between the AKI and non-AKI groups. Categorical variables are expressed as numbers with percentages, and Pearson chi-squared test or Fisher exact test was used to analyze the differences between categorical parameters between the AKI and non-AKI groups. Intraobserver and interobserver agreement was assessed by calculating intraclass correlation coefficients (ICCs). Univariable and multivariable logistic regression analyses were conducted to identify factors associated with AKI. Pearson or Spearman correlation analysis was used to determine the correlation between CEUS-derived parameters and preimplantation biopsy results and renal function indicators of grafts. Receiver operating characteristic (ROC) curves were constructed, and the area under the receiver operating characteristic curve (AUC) was calculated using the trapezoidal rule. Optimal cutoff values for identifying AKI were identified from the highest Youden index. The sensitivity, specificity, positive predictive value (PPV), and negative predictive value (NPV) were calculated using cutoffs obtained from the ROC curves. Analysis of variance (ANOVA) was used to assess changes in peak intensity of kidney cortex (PIKC) and time to peak of kidney cortex (TTPKC) with the stage of AKI. A 2-sided P value <0.05 was considered statistically significant. Statistical analysis was performed using the R (R version 4.1.2, The R Foundation for Statistical Computing) language and associated data packages.
Results
Participants’ baseline demographic and clinical characteristics
Donors’ clinical background information, ultrasound and TIC parameters, and the final laboratory data before procurement are summarized in Table 1 (additional information and data are shown in Table S1). Based on KDIGO criteria, the proportion of donor kidneys with AKI was 46.8%, and kidneys of KDIGO stage I, II, and III accounted for 27.3% (n=12), 40.9% (n=18), and 31.8% (n=14), respectively. Statistically significant differences between the AKI and non-AKI groups were found in the clinical data of the donors in terms of mean age, serum creatinine, The Kidney Donor Profile Index (KDPI), eGFR, uric acid, and urea (P<0.05).
Table 1
Characteristic | Overall (n=94) | Non-AKI (n=50) | AKI (n=44) | P value |
---|---|---|---|---|
Clinical index | ||||
AKI stage (1/2/3) | 12/18/14 | 0/0/0 | 12/18/14 | <0.001* |
Sex (male/female) | 81/13 | 41/9 | 40/4 | 0.34 |
Mean age (years) | 45.1±10.4 | 43.1±12.5 | 47.4±6.8 | 0.041* |
BMI (kg/m2) | 22.53 (21.23, 24.22) | 22.49 (20.81, 23.67) | 22.6 (21.9, 27.04) | 0.13 |
KDPI | 0.27 (0.15, 0.49) | 0.21 (0, 0.37) | 0.37 (0.27, 0.51) | 0.001* |
Serum creatinine (µmol/L) | 91.5 (71.25, 169) | 75 (66, 88) | 170 (140, 247) | <0.001* |
eGFR (mL/min/1.73 m2) | 94.56 (58.02, 129.04) | 112.98 (95.17, 136.46) | 56.19 (43.22, 76.07) | <0.001* |
Uric acid (µmol/L) | 205 (137, 300.5) | 180 (140.25, 242.25) | 273 (134, 415) | 0.009* |
Urea (µmol/L) | 7.25 (3.88, 10.91) | 4.61 (2.45, 7.32) | 10.56 (7.36, 13.97) | <0.001* |
Total Banff score | 2.55±2.76 | 1.26±1.41 | 4.02±3.17 | <0.001* |
Chronic Banff score | 1.85±2 | 0.94±1.13 | 2.89±2.26 | <0.001* |
Acute Banff score | 0.7±1.04 | 0.32±0.51 | 1.14±1.29 | <0.001* |
US index | ||||
RI | 0.68±0.11 | 0.67±0.1 | 0.68±0.12 | 0.67 |
ATKA (seconds) | 9.88 (8.40, 13.27) | 8.72 (7.92, 10.73) | 11.77 (9.43, 16.03) | <0.001* |
TTPKA (seconds) | 8.11 (6.47, 11.32) | 7.99 (6.51, 10.26) | 8.54 (6.25, 12.19) | 0.64 |
TTPKC (seconds) | 7.87 (6.58, 10.1) | 6.97 (5.82, 8.67) | 8.86 (7.76, 11.18) | <0.001* |
TTPKM (seconds) | 13.7 (10.96, 17.18) | 12.88 (10.37, 16.44) | 15.32 (11.75, 17.42) | 0.08 |
PIKA | 180.42±31.76 | 189.44±29.3 | 170.17±31.65 | 0.003* |
PIKC | 163.12±38.39 | 176.8±34.02 | 147.58±37.48 | <0.001* |
PIKM | 118.23±34.19 | 127.42±32.16 | 107.79±33.76 | 0.005* |
TI(KA-KC) (seconds) | −0.3 (−1.82, 1.68) | −1.21 (−2.16, 0.2) | 0.54 (−0.96, 2.41) | 0.005* |
TI(KA-KM) (seconds) | 4.74±4.37 | 4.32±4.52 | 5.23±4.2 | 0.32 |
TI(KC-KM) (seconds) | 4.99±4.19 | 5.43±4.62 | 4.48±3.63 | 0.28 |
The values represent the mean ± SD, median (25th–75th percentiles), or number of patients. *, statistically significant difference between the AKI and non-AKI groups. AKI, acute kidney injury; BMI, body mass index; KDPI, The Kidney Donor Profile Index; eGFR, estimated glomerular filtration rate; US, ultrasound; RI, resistive index; AT, arrival time; KA, kidney segmental artery; TTP, time to peak; KC, kidney cortex; KM, kidney medulla; PI, peak intensity; TI, time interval; SD, standard deviation.
Comparison of CEUS parameters
CEUS parameters were compared between donor kidneys with AKI and those without AKI. ATKA (P<0.001), TTPKC (P<0.001), and TI (KA-KC) (P=0.005) of the non-AKI group were significantly shorter than those of the AKI group. PIKA (P=0.003), PIKC (P<0.001), and PIKM (P=0.005) of the non-AKI group were significantly higher than those of the AKI group (Figure 3). No significant differences were found in RI between the AKI and Non-AKI groups. TTPKC and PIKC values in patients with different stages of AKI (KDIGO stage 1, 2, or 3) and patients without AKI are shown in Figure 4. TTPKC (P=0.42) was not significantly lower with increasing stage of AKI, but PIKC (P=0.01) was significantly higher with increasing stage of AKI. The intraobserver ICC values for TTPKC and PIKC were 0.95 [95% confidence interval (CI): 0.92–0.97; P<0.001] and 0.98 (95% CI: 0.97–0.98; P<0.001), respectively, while the interobserver ICC values were 0.92 (95% CI: 0.87–0.95; P<0.001) and 0.95 (95% CI: 0.92–0.97; P<0.001), respectively. The ICCs for other CEUS parameters are shown in Table S2.
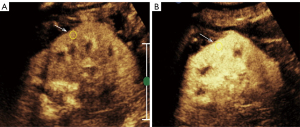
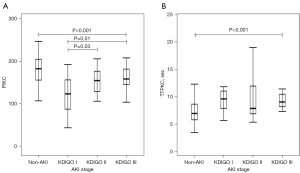
Independent factors associated with AKI
Univariable regression analysis showed that serum creatinine [odds ratio (OR) =1.05; 95% CI: 1.03–1.06; P<0.001], TTPKC (OR =1.27; 95% CI: 1.09–1.48; P=0.002), PIKA (OR =0.98; 95% CI: 0.97–0.99; P=0.005), PIKC (OR =0.98; 95% CI: 0.96–0.99; P=0.001), PIKM (OR =0.98; 95% CI: 0.97–1; P=0.007), and TI (KA-KC; OR =1.25; 95% CI: 1.06–1.46; P=0.007) were risk factors associated with AKI. Subsequently, multivariable regression analysis showed that serum creatinine (OR =1.06; 95% CI: 1.03–1.1; P<0.001); TTPKC (OR =1.38; 95% CI: 1.03–1.84; P=0.03), and PIKC (OR =0.95; 95% CI: 0.91–1; P=0.046) were the independent factors of AKI (Table 2). ROC curves were constructed for the three independent factors of AKI, and the AUC values for identifying AKI for serum creatinine, TTPKC, and PIKC were 0.91, 0.73, and 0.71, respectively (Table 3). The most appropriate cutoff value for identifying AKI for TTPKC was 7.33, and the sensitivity, specificity, NPV, and PPV were 81.8%, 62%, 79.5%, and 65.5%, respectively. The most appropriate cutoff value for identifying AKI for PIKC was 188.52, and the sensitivity, specificity, NPV, and PPV were 88.6%, 48%, 82.7%, and 60%, respectively.
Table 2
Parameter | Univariable analysis | Multivariable analysis | |||
---|---|---|---|---|---|
OR (95% CI) | P value | OR (95% CI) | P value | ||
Serum creatinine† | 1.05 (1.03–1.06) | <0.001 | 1.06 (1.03–1.1) | <0.001 | |
RI | 2.31 (0.05–100.2) | 0.66 | – | – | |
TTPKA | 1.04 (0.93–1.15) | 0.52 | – | – | |
TTPKC‡ | 1.27 (1.09–1.48) | 0.002 | 1.38 (1.03–1.84) | 0.03 | |
TTPKM | 1.1 (0.99–1.23) | 0.08 | – | – | |
PIKA† | 0.98 (0.97–0.99) | 0.005 | 1.03 (0.98–1.08) | 0.21 | |
PIKC‡ | 0.98 (0.96–0.99) | 0.001 | 0.95 (0.91–1) | 0.046 | |
PIKM† | 0.98 (0.97–1) | 0.007 | 0.99 (0.94–1.04) | 0.72 | |
TI(KA-KC)† | 1.25 (1.06–1.46) | 0.007 | 0.96 (0.67–1.37) | 0.83 | |
TI(KA-KM) | 1.05 (0.96–1.15) | 0.32 | – | – | |
TI(KC-KM) | 0.95 (0.86–1.04) | 0.27 | – | – |
†, significant factor in univariable analysis only; ‡, significant factor in both univariable and multivariable analyses. OR, odd ratio; CI, confidence interval; RI, resistive index; TTP, time to peak; KA, kidney segmental artery; KC, kidney cortex; KM, kidney medulla; PI, peak intensity; TI, time interval.
Table 3
Parameters | Cutoff | AUC | Sensitivity (%) | Specificity (%) | PPV (%) | NPV (%) | P value |
---|---|---|---|---|---|---|---|
Serum creatinine | 104 | 0.91 (0.85, 0.97) | 86.4 (76, 97); [38/44] | 86 (76, 96); [43/50] | 84.4 (74, 95); [38/45] | 87.8 (79, 97); [43/49] | <0.001 |
TTPKC | 7.33 | 0.73 (0.63, 0.83) | 81.8 (70, 93); [36/44] | 62 (49, 76); [31/50] | 65.5 (53, 78); [36/55] | 79.5 (69, 92); [31/39] | <0.001 |
PIKC | 188.52 | 0.71 (0.61, 0.82) | 88.6 (75, 96); [39/44] | 48 (34, 62); [24/50] | 60 (47, 72); [39/65] | 82.8 (64, 93); [24/29] | <0.001 |
TTPKC + PIKC + serum creatinine | 0.432 | 0.96 (0.93, 1) | 90.9 (82, 99); [40/44] | 96 (91, 100); [48/50] | 95.2 (89, 102); [40/42] | 92.3 (85, 100); [48/52] | <0.001 |
Data in parentheses are 95% CIs, with numbers of patients in square brackets. ROC, receiver operating characteristic; AKI, acute kidney injury; AUC, area under the ROC; PPV, positive predictive value; NPV, negative predictive value; TTPKC, time to peak of kidney cortex; PIKC, peak intensity of kidney cortex.
Preimplantation biopsy characteristics
Statistically significant differences between the AKI and non-AKI groups were found in pathological characteristics of donor kidneys in terms of percentage of global glomerulosclerosis, interstitial fibrosis, tubular atrophy, interstitial inflammation, arterial intimal fibrosis, arteriolar hyalinosis, glomerular thrombi, and acute tubular injury/necrosis (P<0.05) (pr-implantation biopsy characteristics are shown in Table S2). Based on the semiquantitative analysis, the total Banff score (mean 4.02±3.17 vs. 1.26±1.41; P<0.001), chronic Banff score (2.89±2.26 vs. 0.94±1.13; P<0.001), and acute Banff score (1.14±1.29 vs. 0.32±0.51; P<0.001) of the AKI group were significantly higher than those of the non-AKI group. The correlations of PIKC and TTPKC with preimplantation biopsy characteristics are shown in Table 4. TTPKC showed weak correlation with interstitial fibrosis (r=0.23; P=0.03). PIKC showed correlation with the total Banff score (r=−0.21; P=0.04), chronic Banff score (r=−0.27; P=0.01), arterial intimal fibrosis (r=−0.29, P=0.004), and arteriolar hyalinosis (r=−0.27; P=0.008).
Table 4
Characteristic | Correlation with PIKC | Correlation with TTPKC | |||
---|---|---|---|---|---|
r | P | r | P | ||
Total Banff score | −0.21* | 0.04* | 0.1 | 0.35 | |
Chronic Banff score | −0.27* | 0.01* | 0.05 | 0.63 | |
Acute Banff score | 0.02 | 0.85 | 0.14 | 0.19 | |
Percentage of global glomerulosclerosis | 0 | 0.99 | 0.05 | 0.66 | |
Interstitial fibrosis | −0.12 | 0.25 | 0.23* | 0.03* | |
Tubular atrophy | −0.06 | 0.56 | 0.05 | 0.61 | |
Interstitial inflammation | −0.09 | 0.40 | 0.13 | 0.23 | |
Arterial intimal fibrosis | −0.29* | 0.004* | −0.03 | 0.74 | |
Arteriolar hyalinosis | −0.27* | 0.008* | 0.07 | 0.51 | |
Glomerular thrombi | −0.02 | 0.87 | 0.18 | 0.09 | |
Acute tubular injury/necrosis | 0.08 | 0.46 | 0.08 | 0.46 |
*, the significant correlations. CEUS, contrast-enhanced ultrasonography; PIKC, peak intensity of kidney cortex; TTPKC, time to peak of kidney cortex.
Correlation between CEUS and renal function parameters
A total of 92 kidney transplants were completed out of 94 donor kidneys. Two donor kidneys with AKI were discarded due to unsatisfactory biopsy results. All kidney transplants were performed by an experienced surgeon. The recipients’ baseline demographic characteristics and early post-transplant renal function are shown in Table S3. The correlations of TTPKC and PIKC with renal function parameters are summarized in Table 5. TTPKC showed weak and nonsignificant correlations with serum creatinine, 24-hour urine volume, and eGFR. PIKC also showed weak and nonsignificant correlations with serum creatinine and 24-hour urine volume. In comparison, PIKC showed the strongest correlation with eGFR on postoperative day 7 in the AKI group (r=−0.46; P=0.046) but not in the non-AKI group. Significant correlations with PIKC were invariably negative.
Table 5
Characteristic | AKI (n=42) | Non-AKI (n=50) | |||
---|---|---|---|---|---|
Correlation with PIKC | Correlation with TTPKC | Correlation with PIKC | Correlation with TTPKC | ||
Preoperative kidney function | |||||
Serum creatinine (µmol/L) | r=0.27; P=0.09 | r=−0.07; P=0.64 | r=0.17; P=0.25 | r=−0.14; P=0.35 | |
eGFR (mL/min/1.73 m2) | r=−0.06; P=0.78 | r=−0.08; P=0.72 | r=0.12; P=0.51 | r=0.15; P=0.41 | |
Postoperative kidney function | |||||
Day 3 urine volume (mL) | r=0.04; P=0.78 | r=−0.26; P=0.11 | r=−0.06; P=0.69 | r=−0.06; P=0.67 | |
Day 7 urine volume (mL) | r=0.01; P=0.97 | r=−0.2; P=0.21 | r=0.01; P=0.96 | r=0.15; P=0.32 | |
Day 3 serum creatinine (µmol/L) | r=0.09; P=0.6 | r=−0.09; P=0.58 | r=0.14; P=0.35 | r=−0.01; P=0.97 | |
Day 7 serum creatinine (µmol/L) | r=0.13; P=0.43 | r=0.04; P=0.79 | r=0.08; P=0.6 | r=0.05; P=0.74 | |
Day 30 serum creatinine (µmol/L) | r=0.19; P=0.32 | r=0.13; P=0.49 | r=0.19; P=0.22 | r=0.07; P=0.64 | |
Day 90 serum creatinine (µmol/L) | r=0.17; P=0.44 | r=0.3; P=0.16 | r=0.07; P=0.69 | r=0.06; P=0.72 | |
Day 7 eGFR (mL/min/1.73 m2) | r=−0.46*; P=0.046* | r=−0.1; P=0.7 | r=0.17; P=0.35 | r=0.03; P=0.89 | |
Day 30 eGFR (mL/min/1.73 m2) | r=−0.45; P=0.08 | r=−0.01; P=0.97 | r=−0.2; P=0.3 | r=−0.05; P=0.8 | |
Day 90 eGFR (mL/min/1.73 m2) | r=−0.03; P=0.91 | r=−0.04; P=0.87 | r=0.15; P=0.45 | r=−0.01; P=0.96 |
*, the strongest correlations. CEUS, contrast-enhanced ultrasonography; AKI, acute kidney injury; PIKC, peak intensity of kidney cortex; TTPKC, time to peak of kidney cortex; eGFR, estimated glomerular filtration rate.
Discussion
In this study, we applied CEUS to detect blood perfusion in the kidneys of brain-dead donors. We found that the microcirculation blood perfusion of donor kidneys with AKI was significantly reduced compared with that of donor kidneys without AKI and that the reduced perfusion in the KC may be a characteristic manifestation of AKI. CEUS-derived quantitative parameters (PIKC and TTPKC) could be used to identify AKI; furthermore, PIKC and TTPKC correlated with preimplantation biopsy findings, and PIKC showed the strongest correlation with eGFR on postoperative day 7 in the donor kidneys with AKI.
AKI is a common clinical syndrome in the ICU setting and occurs in more than 50% of patients (19). Renal ischemia caused by impaired renal perfusion is traditionally considered one of the most common causative factors leading to AKI in acutely ill patients (14). CEUS may be the only study tool clinically available for the noninvasive quantification and assessment of renal perfusion and intra-organ blood flow distribution during AKI (10).
AKI is frequently seen in brain-dead donors. Therefore, assessing renal perfusion in the donor kidney is critical, but to our knowledge, no studies have used CEUS to detect and identify AKI in brain-dead donors. In our study, donor kidneys with AKI had reduced blood perfusion in all ROIs compared with donor kidneys without AKI, and CEUS-derived quantitative parameters, TTPKC and PIKC, were shown to be independent risk factors for AKI. The PI reflects the amount of microbubble contrast agent in the vascular bed of the kidney, while the TTP reflects the enhancement process of the contrast agent in the kidney, which truly and directly reflects the perfusion of the contrast agent in the kidney and is a sensitive indicator of renal perfusion (12). We observed a significant delay in TTP and a decrease in PI in the cortex of kidneys with AKI compared with kidneys without AKI, which may be directly related to the impaired cortical microcirculation in the kidney and consequently inadequate perfusion. Harrois et al. (20) were the first to use CEUS to quantify renal cortical perfusion in patients with septic shock and found that reduced cortical renal perfusion was associated with the development of severe AKI; however, no significant difference was found between the intensity parameters of patients without AKI and those with severe AKI. An animal study by Cao et al. (16) found not only significant differences between mice with ischemic AKI and sham mice in renal cortical perfusion but also that perfusion decreased with the severity of AKI. In our study, by comparing PIKC across AKI stages, we found that PIKC increased significantly with the severity of AKI, which may be related to our organ maintenance procedures. Specifically, when brain-dead donors developed severe AKI (persistent elevation of serum creatinine and decrease in urine output), we administered hemodialysis to promote recovery of renal function. This process generally continued until organ procurement, and renal function in kidneys with severe AKI might have improved by the time we performed bedside CEUS prior to procurement.
We also observed that the sensitivity of TTPKC and PIKC for identifying AKI was 81.8% (36 of 44 kidneys) and 88.6% (39 of 44 kidneys), respectively. Some kidneys with AKI not being correctly identified may be related to the recovery of renal function. The kidney has a strong ability to recover from injury, and AKI is considered a reversible process if the cause is corrected (21). Similarly, the sensitivity of final serum creatinine before procurement was only 86.4% (38 of 44 kidneys) in this study. The diagnosis of AKI is based on the changes in serum creatinine and urine volume within 7 days (4), whereas our CEUS examination is typically performed prior to procurement, which may better facilitate the surgeon’s assessment of real-time renal perfusion and function in donors. In addition, these two CEUS-derived parameters are measured in the KC and can be obtained simultaneously by TICs, which is easier to operate in clinical practice.
Another finding of this study was the correlation between CEUS-derived parameters and preimplantation biopsy results and early post-transplant renal function in grafts from donor kidneys with AKI. Among them, the correlation between PIKC and preimplantation biopsy was mainly manifest as chronic lesions in the kidney microartery. We believe that the main reasons for this are the anatomical characteristics of the kidney and the properties of the microbubble contrast agent. The arterial system of the kidney is rich in blood flow, with the cortex taking approximately 90% of the total blood flow to the kidney. The microbubble contrast agent used in CEUS is entirely intravascular, and it reaches the microvasculature of the kidney to allow for the capture of images that quantify renal microvascular blood volume and flow, and these hemodynamic changes can help to reveal renal function (22). When chronic lesions of the renal microvasculature occur, the microcirculation in the KC is impaired and CEUS shows a decrease in cortical perfusion. Whether there is a relationship between CEUS-derived perfusion volume and renal function has not been adequately investigated. A recent study by El-Bandar et al. (23) which used CEUS to quantify perfusion in living donor kidneys suggested, for the first time, that signal intensity of CEUS in normal-weight living donor kidneys may correlate with pre- and post-transplant renal function. In our study, PIKC also showed the strongest negative correlation with eGFR in grafts from donor kidneys with AKI. In contrast, PIKC and TTPKC did not show significant correlations with renal function in grafts from donor kidneys without AKI. This further suggests that reduced perfusion in the KC may be a characteristic manifestation of AKI. However, it should be noted that intensity parameters are influenced by BMI, organ depth, and other factors.
Limitations
This study has several limitations. First, we employed single-center design with a small sample size. Larger prospective clinical studies or multicenter studies are needed to further confirm the findings of this study. Second, CEUS is an operator-dependent examination, and measurement heterogeneity is a common limitation of all applications of CEUS in quantifying renal perfusion. To minimize the resulting heterogeneity, all examinations were performed the same radiologists and machine. Third, the influence of potential biases such as donor maintenance procedures cannot be ruled out.
Conclusions
CEUS can be used to identify AKI in brain-dead donors. Decreased microcirculation blood perfusion in the KC may be a characteristic manifestation of AKI, and CEUS-derived parameters (PIKC and TTPKC) might be used to identify AKI. Furthermore, there is a correlation between CEUS-derived parameters and pretransplant biopsy results and early post-transplant renal function of grafts. As the demand for kidney transplantation increases, CEUS promises to be a routine examination for donor kidney perfusion and function assessment, but some uncertainties to this approach require further research.
Acknowledgments
We would like to thank Ziyi Lu and Bing Chu (Pathology Department, Zhongshan Hospital of Sun Yat-sen University, Zhongshan City People’s Hospital) for their assistance with the pathological analysis as well as LetPub (www.letpub.com) for linguistic assistance and pre-submission expert review.
Funding: None.
Footnote
Reporting Checklist: The authors have completed the STARD reporting checklist. Available at https://qims.amegroups.com/article/view/10.21037/qims-23-207/rc
Conflicts of Interest: All authors have completed the ICMJE uniform disclosure form (available at https://qims.amegroups.com/article/view/10.21037/qims-23-207/coif). The authors have no conflicts of interest to declare.
Ethical Statement: The authors are accountable for all aspects of the work in ensuring that questions related to the accuracy or integrity of any part of the work are appropriately investigated and resolved. The study was conducted in accordance with the Declaration of Helsinki (as revised in 2013) and was approved by the Ethics Committee of Zhongshan City People’s Hospital (No. K2019-058). The donors’ families were informed of the renal biopsy and CEUS procedure and possible risks 24 hours prior to the examination, and they provided signed informed consent. All recipients who received kidney donations also provided signed informed consent.
Open Access Statement: This is an Open Access article distributed in accordance with the Creative Commons Attribution-NonCommercial-NoDerivs 4.0 International License (CC BY-NC-ND 4.0), which permits the non-commercial replication and distribution of the article with the strict proviso that no changes or edits are made and the original work is properly cited (including links to both the formal publication through the relevant DOI and the license). See: https://creativecommons.org/licenses/by-nc-nd/4.0/.
References
- Vanholder R, Domínguez-Gil B, Busic M, et al. Organ donation and transplantation: a multi-stakeholder call to action. Nat Rev Nephrol 2021;17:554-68. [Crossref] [PubMed]
- Lentine KL, Smith JM, Hart A, et al. OPTN/SRTR 2020 Annual Data Report: Kidney. Am J Transplant 2022;22:21-136. [Crossref] [PubMed]
- Yoshikawa MH, Rabelo NN, Welling LC, et al. Brain death and management of the potential donor. Neurol Sci 2021;42:3541-52. [Crossref] [PubMed]
- Ronco C, Bellomo R, Kellum JA. Acute kidney injury. Lancet 2019;394:1949-64. [Crossref] [PubMed]
- Liu C, Hall IE, Mansour S, et al. Association of Deceased Donor Acute Kidney Injury With Recipient Graft Survival. JAMA Netw Open 2020;3:e1918634. [Crossref] [PubMed]
- Siedlecki A, Irish W, Brennan DC. Delayed graft function in the kidney transplant. Am J Transplant 2011;11:2279-96. [Crossref] [PubMed]
- Heilman RL, Smith ML, Kurian SM, et al. Transplanting Kidneys from Deceased Donors With Severe Acute Kidney Injury. Am J Transplant 2015;15:2143-51. [Crossref] [PubMed]
- Hall IE, Schröppel B, Doshi MD, et al. Associations of deceased donor kidney injury with kidney discard and function after transplantation. Am J Transplant 2015;15:1623-31. [Crossref] [PubMed]
- Koyawala N, Parikh CR. A Review of Donor Acute Kidney Injury and Posttransplant Outcomes. Transplantation 2020;104:1553-9. [Crossref] [PubMed]
- Schneider AG, Goodwin MD, Bellomo R. Measurement of kidney perfusion in critically ill patients. Crit Care 2013;17:220. [Crossref] [PubMed]
- Privratsky JR, Wang N, Qi Y, et al. Dynamic contrast-enhanced MRI promotes early detection of toxin-induced acute kidney injury. Am J Physiol Renal Physiol 2019;316:F351-9. [Crossref] [PubMed]
- Jin Y, Yang C, Wu S, et al. A novel simple noninvasive index to predict renal transplant acute rejection by contrast-enhanced ultrasonography. Transplantation 2015;99:636-41. [Crossref] [PubMed]
- Hai Y, Chong W, Liu JB, et al. The Diagnostic Value of Contrast-Enhanced Ultrasound for Monitoring Complications After Kidney Transplantation-A Systematic Review and Meta-Analysis. Acad Radiol 2021;28:1086-93. [Crossref] [PubMed]
- Matejovic M, Ince C, Chawla LS, et al. Renal Hemodynamics in AKI: In Search of New Treatment Targets. J Am Soc Nephrol 2016;27:49-58. [Crossref] [PubMed]
- Liu PQ, Ding CW, Zhang YC, et al. Diagnostic value of ultrasound and contrast-enhanced ultrasound in septic acute kidney injury. J Clin Ultrasound 2022;50:505-14. [Crossref] [PubMed]
- Cao W, Cui S, Yang L, et al. Contrast-Enhanced Ultrasound for Assessing Renal Perfusion Impairment and Predicting Acute Kidney Injury to Chronic Kidney Disease Progression. Antioxid Redox Signal 2017;27:1397-411. [Crossref] [PubMed]
- Liapis H, Gaut JP, Klein C, et al. Banff Histopathological Consensus Criteria for Preimplantation Kidney Biopsies. Am J Transplant 2017;17:140-50. [Crossref] [PubMed]
- Levey AS, Stevens LA, Schmid CH, et al. A new equation to estimate glomerular filtration rate. Ann Intern Med 2009;150:604-12. [Crossref] [PubMed]
- Hoste EA, Bagshaw SM, Bellomo R, et al. Epidemiology of acute kidney injury in critically ill patients: the multinational AKI-EPI study. Intensive Care Med 2015;41:1411-23. [Crossref] [PubMed]
- Harrois A, Grillot N, Figueiredo S, et al. Acute kidney injury is associated with a decrease in cortical renal perfusion during septic shock. Crit Care 2018;22:161. [Crossref] [PubMed]
- Vanmassenhove J, Kielstein J, Jörres A, et al. Management of patients at risk of acute kidney injury. Lancet 2017;389:2139-51. [Crossref] [PubMed]
- Emanuel AL, Meijer RI, van Poelgeest E, et al. Contrast-enhanced ultrasound for quantification of tissue perfusion in humans. Microcirculation 2020;27:e12588. [Crossref] [PubMed]
- El-Bandar N, Lerchbaumer MH, Peters R, et al. Kidney Perfusion in Contrast-Enhanced Ultrasound (CEUS) Correlates with Renal Function in Living Kidney Donors. J Clin Med 2022;11:791. [Crossref] [PubMed]