The application of 18F-FDG PET/CT imaging for human hepatocellular carcinoma: a narrative review
Introduction
Hepatocellular carcinoma (HCC) is the most common primary malignant tumor of the liver and the fourth leading cause of cancer-related death globally (1). The mean overall survival (OS) for HCC is 15.8 months whereas the 6-month and 1-year OS rates are 71.6% and 49.7%, respectively (2). The etiology of HCC is closely related to viral hepatitis, non-alcoholic fatty liver disease, and subsequent cirrhosis (3). Due to its morbidity and high mortality, HCC poses a significant threat to human health. The current treatment options for HCC patients mainly include radiofrequency ablation/percutaneous ethanol injection, partial liver resection, and transarterial chemoembolization (4).
Early diagnosis of HCC is particularly important when considering treatment options and the prognosis of patients. In clinical practice, liver biopsy is not routinely performed due to the risk of tumor spread and bleeding. At present, the diagnosis of HCC is mainly based on imaging examinations such as ultrasound, computed tomography (CT) and magnetic resonance imaging (MRI), and related laboratory tests. However, morphological characteristics, based on the number and size of tumors, do not incorporate the biological behavior of the tumor, and thus would not accurately predict aggressiveness, prognosis, and the risk of recurrence of the tumor.
18F-fluorodeoxyglucose (18F-FDG) positron emission tomography (PET) imaging is widely used for the management of several solid cancers and is also used to monitor recurrence, metastatic spread, and the response to therapy, thus providing valuable prognostic information (5,6). PET is valuable for reflecting the biological status of tumor aggressiveness which is highly associated with the tumor pathology (7). However, HCC cases frequently exhibit low 18F-FDG uptake. The so called “false-negative” rate of 18F-FDG PET/CT is almost 50% when imaging HCC (8). Therefore, 18F-FDG PET is not generally considered very useful for HCC visualization and is not currently a recommended standard imaging modality for the diagnosis of HCC. Subsequently, 11C-choline and other PET tracers have been used for HCC visualization; the detection rate and sensitivity of these methods for lesions has been reported to be higher than that of 18F-FDG (9). However, a growing body of evidence indicates that 18F-FDG PET/CT has important clinical significance for therapeutic strategy, staging, prognosis, and recurrence in HCC patients (10-12). Although multiple PET tracers have been developed and attempted for HCC visualization, none have been shown to be superior to 18F-FDG in clinical practice. Patients with 18F-FDG-positive lesions have a higher risk of recurrence; survival time and survival rates are significantly less favorable than those with 18F-FDG-negative lesions (13-15). 18F-FDG non-avidity HCC may reflect a relatively more favorable outcome.
Studies of patients with lung cancer, colorectal cancer, and microscopic peritoneal tumors have revealed that 18F-FDG uptake may not be intrinsic to solid cancer, and that hypoxia may be one of the most fundamental driving forces, thus resulting in altered energy metabolism by virtue of increased anaerobic glycolysis (16-21). Hypoxia-induced glucose transporter expression may lead to increased FDG uptake, leading to the transformation of HCC into a more aggressive disease phenotype, such as a larger tumor size, recurrence, and poor survival (22). The relationship between 18F-FDG uptake and hypoxia may help to explain the relationship between 18F-FDG uptake and prognosis, thereby providing more effective clinical treatment decisions for HCC patients.
Articles relating to the application of 18F-FDG PET/CT in HCC are controversial. Some believe that the role of 18F-FDG PET in the diagnosis of HCC is minimal (8,9), yet many others have shown that it is of great significance to the prognosis of HCC (10-12). Research has shown that the uptake of FDG in well-differentiated tumor cells is low and that the prognosis of patients is good. In this article, we revisit and review the usefulness of 18F-FDG PET/CT for visualizing, managing, and investigating the biological properties of HCC. In addition, we discuss the potential mechanisms underlying 18F-FDG uptake in HCC. We present this article in accordance with the Narrative Review reporting checklist (available at https://qims.amegroups.com/article/view/10.21037/qims-22-1420/rc).
Methods
A search was conducted in the database of PubMed for articles on the application of 18F-FDG PET/CT imaging for human HCC up to December 2021. The search terms used included the following: ‘Hepatocellular carcinoma’, ‘[18F]FDG PET/CT’, ‘Hypoxia’, ‘[11C]Choline’. The detailed search strategy is displayed in Table 1.
Table 1
Items | Specification |
---|---|
Date of search | 2021.12.31 |
Databases | PubMed |
Search terms used | ‘Hepatocellular carcinoma’[MeSH] OR ‘HCC’ [MeSH] |
‘[18F]FDG uptake’[MeSH] AND ‘Tumor’ [MeSH] | |
‘[18F]FDG PET/CT’ [MeSH] | |
‘Hepatocellular carcinoma’[MeSH] AND ‘[18F]FDG PET/CT’ [MeSH] | |
‘Hypoxia’ [MeSH] AND ‘Tumor’ [MeSH] | |
‘[11C]Choline’ [MeSH] AND ‘Hepatocellular carcinoma’ [MeSH] | |
‘[18F]FDG PET/CT’ [MeSH] AND ‘Tumor’ [MeSH] | |
Timeframe | 1990–2021 |
Inclusion and exclusion criteria | Articles about PET/CT and human hepatocellular carcinoma was mainly included. It excluded articles that have no [18F]FDG |
Selection process | It was conducted independently by Yong Yao and Xiao-Feng Li; data selection is the intersection of the search of two authors |
18F-FDG PET/CT, 18F-fluorodeoxyglucose positron emission tomography/computed tomography.
Non-18F-FDG PET imaging agents for HCC
18F-FDG PET is less sensitive for the diagnosis of HCC and is not routinely used as a diagnostic modality for HCC. This has led to studies of other non-18F-FDG PET imaging agents, such as 11C-choline, 11C-acetate, and its 18F-labeled derivatives. The advent of new radiotracers makes it possible to visualize other metabolic processes besides glucose metabolism.
Due to heterogeneity within a tumor, the differential uptake patterns detected by different tracers can be exploited. 11C-acetate is used to evaluate the synthesis of fatty acids; these are related to the growth and invasion of tumor cells. Park et al. studied the combination of 18F-FDG and 11C-acetate in HCC patients and found that the sensitivity of the combined use of tracers was 83%, whereas that of the single tracers was 75% and 60%, respectively (13). Ho et al. also evaluated the use of 18F-FDG in combination with 11C-acetate for HCC patients. These authors found that the sensitivity of 11C-acetate alone in well-differentiated tumors was good, whereas that of 18F-FDG in poorly differentiated tumors was better (23). More choline, especially phosphatidylcholine, is needed as substrate for phospholipid synthesis during malignant tumor transformation; this promotes the development of nuclide-labeled choline compounds as imaging biomarkers for cell membrane synthesis. 11C-choline is a potential tracer that could supplement 18F-FDG to detect HCC lesions. Yamamoto et al. have shown that 11C-choline PET has a higher detection rate for moderately differentiated HCC lesions but not for poorly differentiated HCC lesions; 18F-FDG PET produced the opposite result (9). Furthermore, the development of 18F-fluoroacetate and 18F-fluorocholine overcame the problem created by the short half-life of 11C radionuclides. The alternative or complementary functions of these imaging agents in HCC characterization and the clinical impact of dual-trace PET with FDG and choline/acetate require further research and dosimetry considerations. Our previous clinical results showed that perfusion combined with metabolic 18F-FDG PET/CT could provide information relating to the blood perfusion and glucose metabolism of HCC and could be performed routinely for outcome prediction and anti-cancer management. In our opinion, 11C-choline PET provides little additional information beyond perfusion dynamic 18F-FDG PET.
Application of 18F-FDG PET in HCC
As a functional imaging method, 18F-FDG PET can reflect metabolic changes within the body and could be used for whole body imaging. Combined with the precise positioning of CT, 18F-FDG PET has important clinical significance for treatment strategies, staging, and the prognostic and efficacy evaluation of HCC patients, especially with regards to the detection of metastatic lesions and the monitoring of recurrence (Table 2). According to 18F-FDG PET results, we can accurately assess the benefits and risks of HCC patients, especially in the prediction of the aggressiveness of advanced HCC. We are also able to detect hidden malignancies and extrahepatic metastases that can be missed by other imaging methods (7). 18F-FDG PET allows for the characterization of cancer biology. Poorly differentiated HCCs are more often FDG-positive than well-differentiated HCCs (25). Furthermore, the pattern of 18F-FDG uptake could reflect the possibility of tumor progression and metastasis (26).
Table 2
Clinical significance | Author | Principal comments |
---|---|---|
Diagnostic | Yamamoto Y (9) | The detection rate and sensitivity of 11C-choline for lesions higher than that of 18F-FDG |
Ho CL (23) | The sensitivity of 11C-acetate alone in well-differentiated tumors was good, whereas that of 18F-FDG in poorly differentiated tumors was better | |
Khan MA (8) | The so called “false-negative” rate of 18F-FDG PET/CT is almost 50% when imaging HCC | |
Treatment strategies | Lee SM (10) | With a high FDG uptake, a variety of treatments may be more effective for tumor control |
Kitamura (24) | The level of FDG uptake before rescue liver transplantation was able to identify which patients could benefit from hepatectomy | |
Staging | Ferda J (25) | Poorly differentiated HCCs are more often FDG-positive than well-differentiated HCCs |
Lee JD (26) | the pattern of 18F-FDG uptake could reflect the possibility of tumor progression and metastasis | |
Prognostic | Haug AR (15) | The prognosis of FDG-positive HCC is significantly worse than that of FDG-negative HCC |
Ma W (27) | Patients with a low FDG uptake have longer progression-free survival and longer overall survival | |
Recurrence | Han JH (28) | FDG uptake was significantly associated with tumor recurrence |
Takada (29) | The 5-year recurrence rate of PET-negative patients was significantly lower | |
Ling (30) | The 3-year cumulative recurrence rate with a high FDG uptake was significantly higher |
18F-FDG PET/CT, 18F-fluorodeoxyglucose positron emission tomography/computed tomography; HCC, hepatocellular carcinoma.
The accurate staging of HCC is not only critical to therapeutic options; it is also critical to prognosis. 18F-FDG PET has shown promising results with regards to the detection of extrahepatic metastases. HCCs with a high FDG uptake are more aggressive than those with a low FDG uptake. The uptake of 18F-FDG by HCC is significantly and positively correlated with the tendency for extrahepatic metastasis, and metastatic HCC lesions would also increase the uptake of 18F-FDG (23). HCCs with high FDG uptake also have a high risk of early recurrence and distant metastasis (31). Trojan et al. showed that in patients with moderately or poorly differentiated HCC, 18F-FDG PET facilitated the effective non-invasive staging of patients with tumors >5 cm (32). Takeuchi et al. retrospectively analyzed the records of HCC patients who received FDG PET/CT prior to initial treatment and concluded that FDG PET/CT imaging biomarkers should be considered in the HCC staging system (33). Lin et al. further showed that the combined estimates of sensitivity, specificity, positive likelihood ratio (PLR), and negative likelihood ratio (NLR) of FDG PET for detecting metastatic HCC were 76.6%, 98.0%, 14.68, and 0.28, respectively (34). By evaluating the gene expression profiles of HCC, Lee et al. found that the pathological tumor grade was closely related to the 18F-FDG uptake pattern, and that HCCs with high 18F-FDG uptake had more aggressive biological characteristics than those with low uptake (35).
In addition to detecting unexpected extra-hepatic metastases, PET also increases the prognostic value of predicting survival. Previous studies have shown that among HCC patients receiving treatment, patients with a low FDG uptake have longer progression-free survival (PFS) and longer OS, thus indicating that the FDG avidity of HCC was significantly correlated with clinical outcomes (27,36). It has been reported that tumor doubling time has a positive association with FDG uptake of HCCs (37). Furthermore, Haug et al. have found that in terms of recurrence time, PFS, and survival, the prognosis of FDG-positive HCC is significantly less favorable than that of FDG-negative HCC (15).
18F-FDG PET may be used not only to predict the prognosis, but also to adjust the surgical strategies according to the level of FDG uptake (38). Considering the differences in FDG uptake, HCC will exhibit different tumor characteristics; therefore, different treatment strategies are required according to the performance of FDG PET in HCC patients. A previous study showed that when liver transplantation was performed under neoadjuvant conditions, the level of FDG uptake before rescue liver transplantation could be used to identify which patients could benefit from hepatectomy (24). For HCC patients with a high FDG uptake, a variety of treatments may be more effective in tumor therapy, whereas patients with a low 18F-FDG uptake seem to be less affected by treatment modality (10). In addition, for HCC patients with a high FDG uptake, major hepatectomy should be chosen to minimize the possibility of residual tumors in order to obtain survival benefits (39). Kang et al. studied patients receiving living-donor transplantation and confirmed that combining FDG PET findings with clinical factors could effectively select HCC patients who are candidates for liver transplantation (40).
18F-FDG PET also plays a promising role in monitoring the recurrence of HCC (41). Han et al. found that 18F-FDG uptake was significantly associated with tumor recurrence in HCC patients undergoing curative surgical resection; patients with a high FDG uptake had worse survival (28). The overall risk of recurrence in PET-positive HCC patients is higher than that of negative patients. Takada et al. collected data from 182 HCC patients who received liver transplantation and found that the 5-year recurrence rate of PET-negative patients was significantly lower than that of other patients; furthermore, PET-positive status was a significant and independent risk factor for recurrence (29). A retrospective analysis of 67 HCC patients who underwent curative hepatic resection showed that a tumor-to-normal liver standardized uptake value ratio (TNR) ≥1.53 was an independent predictor of distant metastasis recurrence; the specificity, sensitivity, positive predictive value (PPV), negative predictive value (NPV), and accuracy of distant metastasis recurrence for HCC were 76.4%, 91.7%, 45.8%, 97.7%, and 79.1%, respectively (42). Ling et al. found that the 3-year cumulative recurrence rate with a high FDG uptake was 70.8%, which was significantly higher than that for HCC patients with a low FDG uptake (30).
18F-FDG PET imaging for HCC and hypoxia
The false-negative rate of 18F-FDG PET/CT is almost 50% when imaging patients with HCC. This leaves the question as to why some HCCs take up 18F-FDG yet others do not. It has been reported that 18F-FDG PET/CT has a low sensitivity in the diagnosis of HCC and that this is mainly related to the specificity of glucose uptake in HCC tumor cells. It is believed that in well-differentiated HCC tumor cells, the higher concentration of glucose-6-phosphatase can accelerate the rapid clearance of 18F-FDG, thus resulting in lower 18F-FDG content in tumor cells. The concentration of glucose-6-phosphatase in poorly differentiated HCC cells is relatively lower, the retention of 18F-FDG is higher, and the lesions show high concentrations of radioactivity (43,44).
18F-FDG uptake may be not intrinsic to cancer, although hypoxia may be one of the fundamental driving forces. The common feature of solid tumors is hypoxia; this is closely related to chemotherapy resistance and tumor prognosis (45,46). Hypoxia is caused by the oxygen consumption ratio of tumors exceeding the oxygen supply rate from the vasculature. Due to the unlimited proliferation of tumor cells exceeding the existing vasculature, the oxygen demand is increased (47).
The anoxic microenvironment plays a crucial role in inducing the high uptake of 18F-FDG, thus resulting in the transformation of glucose metabolism to anaerobic glycolysis (48-50). As an energy generation pathway, anaerobic glycolysis has relatively low utilization efficiency and requires more glucose substrate to produce equivalent adenosine triphosphate (ATP). The uptake of 18F-FDG is lower in tumor areas with good blood perfusion; therefore, oxygenated tumor cells cannot be mapped. In HCC, highly differentiated tumor cells with slow proliferation have less 18F-FDG uptake because the rate of angiogenesis remains high. In poorly differentiated liver cancer tissues, due to the excessive proliferation of tumor cells, the rate of angiogenesis cannot keep up, thus resulting in hypoxia; the rate of 18F-FDG uptake remains high. This also indirectly reflects the high 18F-FDG uptake in undifferentiated and poorly differentiated HCC.
Our previous studies demonstrated that 18F-FDG uptake was significantly higher in hypoxic portions of larger tumors and severe hypoxic ascites carcinomas (16,20). Hypoxia-specific probe pimonidazole showed that the spatial distribution of 18F-FDG in the peritoneal tumor model perfectly matched that in the tumor (Figure 1). In addition, our lung cancer clinical data provides indirect evidence that 18F-FDG PET reports tumor hypoxia (Figure 2) (20). Early 18F-FDG dynamic imaging is mainly defined by blood delivery or supply, which can be used to assess tumor perfusion. Over 60-min of 18F-FDG metabolic imaging, the activity accumulated in cells via the GLUT-1/hexokinase pathway, thus indicating that the uptake concentration of 18F-FDG was highest in the tumor volume associated with low blood perfusion (20).
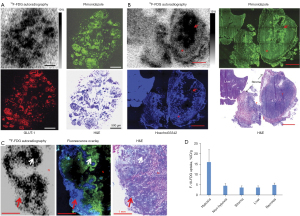
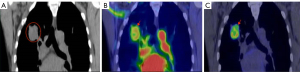
Similar results have been reported in other studies. Hwang et al. retrospectively analyzed the results of multi-detector CT and PET/CT of 66 HCC patients who underwent surgical treatment and confirmed that on the multi-detector CT, the increase of 18F-FDG uptake was associated with a reduction in arterial and portal perfusion. Furthermore, higher 18F-FDG uptake and lower perfusion patterns were significantly associated with shorter OS (51). Xia et al. reported that hypoxia-induced glucose transporter expression may lead to changes in 18F-FDG PET-CT imaging (22). Yamamoto et al. have developed new hyper-polarized MRI and electron paramagnetic resonance imaging procedures that can quantitatively evaluate the glycolysis and oxygenation status of tumors more directly (52). These authors demonstrated that PO2 was negatively correlated with 18F-FDG uptake. Kaira et al. have also found a significant correlation between hypoxia and 18F-FDG uptake (53). These findings demonstrated that viable and well-perfused cancer regions have low 18F-FDG uptake whereas areas with low to absent blood perfusion have high 18F-FDG uptake.
Possible mechanism underlying hypoxia-induced 18F-FDG uptake in HCC
In tumor PET imaging, the degree of hypoxia is highly correlated with 18F-FDG uptake. The specific mechanism of hypoxia-induced 18F-FDG uptake remains unclear. Yao et al. have shown that this may be related to the activation of macrophages, metabolic changes, and glycolysis-related enzyme activity changes induced by hypoxia (54). The lack of hypoxia-related basic research, such as immunohistochemistry and autoradiography, will need to be addressed in the future. Other studies have shown that age and the blood glucose level are also influential factors of liver standardized uptake value (SUV) (55,56).
Hypoxia will cause cells to activate the angiogenesis process to increase oxygen delivery; furthermore, cell fuel metabolism is adjusted from mitochondrial respiration to glycolysis (57,58). Another consequence of hypoxia is the activation of hypoxia inducible factor (HIF) which enhances glycolysis and glucose uptake by directly inducing the transcription of GLUT1 and glycolytic genes (59,60). In order to meet their bioenergetic demands, cells must then rely on glycolysis. Since anaerobic glycolysis is inefficient in energy production, hypoxic tissue cells require more 18F-FDG uptake. In order for tumor cells to meet their rapid growth needs, GLUT1 is overexpressed on the surface of tumor cells, which will facilitate more glucose transport to tumor cells (17). Xia et al. used quantitative reverse transcription polymerase chain reaction (qRT-PCR) to detect the expression of GLUT1 and GLUT3 in different HCC cells under hypoxic and normoxic conditions. These authors showed that hypoxia can significantly induce the expression of GLUT1 and GLUT3 and that this is related to the high rates of glycolysis and 18F-FDG uptake (22).
Hypoxia causes upregulation of hexokinase protein, which promotes glucose uptake and metabolism (61). The diagnostic accuracy of 18F-FDG PET in HCC is limited by the difference in enzyme expression between different tumor gradings. In contrast, the enzyme activity in moderately/poorly differentiated HCC compared with non-involved liver regions resulted in an increase in SUV, thus allowing tumor detection (11). Lee et al. found that HCC with a high tumor-to-liver SUV ratio (TLR) expressed higher levels of glucose transporter isoform 1 than HCC with a low TLR; furthermore, the proliferation and migration of HCC cells with high 18F-FDG uptake reduced after treatment with glucose uptake inhibitors (62). Chen et al. showed that the maximum standardized uptake in HCC patients was inversely correlated with the expression of fructose 1,6-bisphosphatase 1 (FBP1) and that FBP1 may inhibit 18F-FDG uptake through the HIF1A pathway (63).
In HCC, the infiltration of pro-inflammatory macrophages into the tumor microenvironment can promote tumor growth and is associated with invasion and metastasis (64). Active inflammatory processes are common in many tumors, which also predict aggressive behavior. In patients with non-small cell lung cancer, 18F-FDG uptake has been found to be highly correlated with tumor-associated macrophages (TAM) which can significantly enhance tumor hypoxia (65). Reinfeld et al. used PET tracers to measure the acquisition and uptake of glucose and glutamine by specific cell subgroups in the tumor microenvironment and found that the factor responsible for a tumor’s high-speed consumption of glucose is not the cancer cells but macrophages in tumor tissues and other immune cells (66). Different types of cells in the tumor environment consume different nutrients according to their metabolic activities. For example, immune cells and cancer cells preferentially obtain glucose and glutamine, respectively.
Understand the meaning of 18F-FDG uptake in HCC
Since 95% of solid malignant tumors have a certain degree of hypoxia, 18F-FDG PET can be used successfully for cancer surveillance. 18F-FDG accumulates heavily in hypoxic tumor cells with poor proliferation and low perfusion, but undergoes low uptake in oxygen-rich tumor cells with good proliferation and high perfusion (16). Therefore, the correct interpretation of 18F-FDG uptake is of great clinical significance for the formulation of clinical treatment programs, and the evaluation of curative effect and prognosis.
Since 18F-FDG uptake is not obvious in well-differentiated HCC, 18F-FDG PET is not currently a recommended standard imaging modality for the diagnosis of HCC. However, this procedure has important clinical significance in the therapeutic strategy, staging, prognosis, and recurrence of liver cancer patients. Jo et al. found that HCC cells with low proliferation potential under hypoxic conditions have a high possibility of inducing the epithelial-mesenchymal transition process and promoting cell invasion (67). Jo et al. speculated that HCC with a high FDG uptake had a better response to radiotherapy than HCC with low uptake (68). Well-differentiated HCC tumor cells grow slowly; therefore, the blood supply can keep up, hypoxia is not serious, and 18F-FDG uptake is low. However, poorly differentiated HCC tumor cells proliferate faster than angiogenesis; therefore, the blood supply cannot keep up, hypoxia is severe, and 18F-FDG uptake is high. In other words, a high FDG uptake indicates that tumor cells may be seriously hypoxic. The reason for this is that the proliferation rate of tumor cells exceeds the rate of angiogenesis. This also indirectly reflects the poorly differentiated state of tumor cells, thus indicating that the patient has a poor prognosis and a short lifespan. If FDG is not taken up, it means that the tumor cells are not severely hypoxic. The reason for this is that the tumor cells proliferate slowly and the blood supply can keep up. This also indirectly reflects the highly differentiated state of tumor cells.
Poorly differentiated HCCs are more often FDG-positive than well-differentiated HCC. Patients with 18F-FDG-positive lesions have a higher risk of recurrence; furthermore, survival times and survival rates are significantly worse than those with 18F-FDG-negative lesions; therefore, conservative treatment and clinical care should be given priority. A negative result for 18F-FDG uptake is not meaningless; rather, it indicates that the patient has a good prognosis and that active treatment is necessary.
Conclusions
18F-FDG PET/CT is very useful for HCC visualization, management, and the evaluation of biological properties. A negative test for 18F-FDG uptake is not meaningless and may reflect a relatively better outcome. 18F-FDG-positive lesions indicate a significantly less favorable prognosis. Hypoxia may be one of the possible mechanisms underlying 18F-FDG uptake in HCC.
Acknowledgments
Funding: This work was supported by grants from
Footnote
Reporting Checklist: The authors have completed the Narrative Review reporting checklist. Available at https://qims.amegroups.com/article/view/10.21037/qims-22-1420/rc
Conflicts of Interest: All authors have completed the ICMJE uniform disclosure form (available at https://qims.amegroups.com/article/view/10.21037/qims-22-1420/coif). The authors have no conflicts of interest to declare.
Ethical Statement: The authors are accountable for all aspects of the work in ensuring that questions related to the accuracy or integrity of any part of the work are appropriately investigated and resolved.
Open Access Statement: This is an Open Access article distributed in accordance with the Creative Commons Attribution-NonCommercial-NoDerivs 4.0 International License (CC BY-NC-ND 4.0), which permits the non-commercial replication and distribution of the article with the strict proviso that no changes or edits are made and the original work is properly cited (including links to both the formal publication through the relevant DOI and the license). See: https://creativecommons.org/licenses/by-nc-nd/4.0/.
References
- Llovet JM, De Baere T, Kulik L, Haber PK, Greten TF, Meyer T, Lencioni R. Locoregional therapies in the era of molecular and immune treatments for hepatocellular carcinoma. Nat Rev Gastroenterol Hepatol 2021;18:293-313. [Crossref] [PubMed]
- Ziogas IA, Evangeliou AP, Giannis D, Hayat MH, Mylonas KS, Tohme S, Geller DA, Elias N, Goyal L, Tsoulfas G. The Role of Immunotherapy in Hepatocellular Carcinoma: A Systematic Review and Pooled Analysis of 2,402 Patients. Oncologist 2021;26:e1036-49. [Crossref] [PubMed]
- Torre LA, Bray F, Siegel RL, Ferlay J, Lortet-Tieulent J, Jemal A. Global cancer statistics, 2012. CA Cancer J Clin 2015;65:87-108. [Crossref] [PubMed]
- Chang L, Wang Y, Zhang J, Guo T. The best strategy for HCC patients at each BCLC stage: a network meta-analysis of observational studies. Oncotarget 2017;8:20418-27. [Crossref] [PubMed]
- Grootjans W, de Geus-Oei LF, Troost EG, Visser EP, Oyen WJ, Bussink J. PET in the management of locally advanced and metastatic NSCLC. Nat Rev Clin Oncol 2015;12:395-407. [Crossref] [PubMed]
- Civelek AC, Piotrowski B, Osman MM, Memisoglu E, Khayyat N, Reimers HJ, Naunheim KS. Cutaneous metastatic lung cancer detected with 18F-FDG PET. Ann Nucl Med 2006;20:147-9. [Crossref] [PubMed]
- Lee SD, Kim SH. Role of positron emission tomography/computed tomography in living donor liver transplantation for hepatocellular carcinoma. Hepatobiliary Surg Nutr 2016;5:408-14. [Crossref] [PubMed]
- Khan MA, Combs CS, Brunt EM, Lowe VJ, Wolverson MK, Solomon H, Collins BT, Di Bisceglie AM. Positron emission tomography scanning in the evaluation of hepatocellular carcinoma. J Hepatol 2000;32:792-7. [Crossref] [PubMed]
- Yamamoto Y, Nishiyama Y, Kameyama R, Okano K, Kashiwagi H, Deguchi A, Kaji M, Ohkawa M. Detection of hepatocellular carcinoma using 11C-choline PET: comparison with 18F-FDG PET. J Nucl Med 2008;49:1245-8. [Crossref] [PubMed]
- Lee SM, Kim HS, Lee S, Lee JW. Emerging role of (18)F-fluorodeoxyglucose positron emission tomography for guiding management of hepatocellular carcinoma. World J Gastroenterol 2019;25:1289-306. [Crossref] [PubMed]
- Kornberg A, Friess H. (18)F-fludeoxyglucose positron emission tomography for diagnosis of HCC: implications for therapeutic strategy in curative and non-curative approaches. Therap Adv Gastroenterol 2019;12:1756284819836205. [Crossref] [PubMed]
- Yamashige D, Kawamura Y, Kobayashi M, Shindoh J, Kobayashi Y, Okubo S, Muraishi N, Kajiwara A, Iritani S, Fujiyama S, Hosaka T, Saitoh S, Sezaki H, Akuta N, Suzuki F, Suzuki Y, Ikeda K, Arase Y, Hashimoto M, Kumada H. Potential and Clinical Significance of 18F-Fluorodeoxyglucose Positron Emission Tomography/Computed Tomography for Evaluating Liver Cancer Response to Lenvatinib Treatment. Oncology 2021;99:169-76. [Crossref] [PubMed]
- Park JW, Kim JH, Kim SK, Kang KW, Park KW, Choi JI, Lee WJ, Kim CM, Nam BH. A prospective evaluation of 18F-FDG and 11C-acetate PET/CT for detection of primary and metastatic hepatocellular carcinoma. J Nucl Med 2008;49:1912-21. [Crossref] [PubMed]
- Ida Y, Tamai H, Shingaki N, Shimizu R, Maeshima S, Maekita T, Iguchi M, Terada M, Kitano M. Prognostic value of (18)F-fluorodeoxyglucose positron emission tomography in patients with small hepatocellular carcinoma treated by radiofrequency ablation. Cancer Imaging 2020;20:74. [Crossref] [PubMed]
- Haug AR. Imaging of primary liver tumors with positron-emission tomography. Q J Nucl Med Mol Imaging 2017;61:292-300. [Crossref] [PubMed]
- Li XF, Du Y, Ma Y, Postel GC, Civelek AC. (18)F-fluorodeoxyglucose uptake and tumor hypoxia: revisit (18)f-fluorodeoxyglucose in oncology application. Transl Oncol 2014;7:240-7. [Crossref] [PubMed]
- Li XF, Ma Y, Sun X, Humm JL, Ling CC, O'Donoghue JA. High 18F-FDG uptake in microscopic peritoneal tumors requires physiologic hypoxia. J Nucl Med 2010;51:632-8. [Crossref] [PubMed]
- Huang T, Civelek AC, Li J, Jiang H, Ng CK, Postel GC, Shen B, Li XF. Tumor microenvironment-dependent 18F-FDG, 18F-fluorothymidine, and 18F-misonidazole uptake: a pilot study in mouse models of human non-small cell lung cancer. J Nucl Med 2012;53:1262-8. [Crossref] [PubMed]
- Li XF, Huang T, Jiang H, Wang X, Shen B, Wang X, Ng CK, Postel GC, Civelek AC. Combined Injection of (18)F-Fluorodeoxyglucose and 3'-Deoxy-3'-[(18)F]fluorothymidine PET Achieves More Complete Identification of Viable Lung Cancer Cells in Mice and Patients than Individual Radiopharmaceutical: A Proof-of-Concept Study. Transl Oncol 2013;6:775-83.
- Shen B, Huang T, Sun Y, Jin Z, Li XF. Revisit 18F-fluorodeoxyglucose oncology positron emission tomography: "systems molecular imaging" of glucose metabolism. Oncotarget 2017;8:43536-42. [Crossref] [PubMed]
- Zhang G, Li J, Wang X, Ma Y, Yin X, Wang F, Zheng H, Duan X, Postel GC, Li XF. The reverse Warburg effect and 18F-FDG uptake in non-small cell lung cancer A549 in mice: a pilot study. J Nucl Med 2015;56:607-12. [Crossref] [PubMed]
- Xia H, Chen J, Gao H, Kong SN, Deivasigamani A, Shi M, Xie T, Hui KM. Hypoxia-induced modulation of glucose transporter expression impacts (18)F-fluorodeoxyglucose PET-CT imaging in hepatocellular carcinoma. Eur J Nucl Med Mol Imaging 2020;47:787-97. [Crossref] [PubMed]
- Ho CL, Chen S, Yeung DW, Cheng TK. Dual-tracer PET/CT imaging in evaluation of metastatic hepatocellular carcinoma. J Nucl Med 2007;48:902-9. [Crossref] [PubMed]
- Kitamura K, Hatano E, Higashi T, Seo S, Nakamoto Y, Yamanaka K, Iida T, Taura K, Yasuchika K, Uemoto S. Preoperative FDG-PET predicts recurrence patterns in hepatocellular carcinoma. Ann Surg Oncol 2012;19:156-62. [Crossref] [PubMed]
- Ferda J, Ferdová E, Baxa J, Kreuzberg B, Daum O, Třeška V, Skalický T. The role of 18F-FDG accumulation and arterial enhancement as biomarkers in the assessment of typing, grading and staging of hepatocellular carcinoma using 18F-FDG-PET/CT with integrated dual-phase CT angiography. Anticancer Res 2015;35:2241-6.
- Lee JD, Yang WI, Park YN, Kim KS, Choi JS, Yun M, Ko D, Kim TS, Cho AE, Kim HM, Han KH, Im SS, Ahn YH, Choi CW, Park JH. Different glucose uptake and glycolytic mechanisms between hepatocellular carcinoma and intrahepatic mass-forming cholangiocarcinoma with increased (18)F-FDG uptake. J Nucl Med 2005;46:1753-9.
- Ma W, Jia J, Wang S, Bai W, Yi J, Bai M, Quan Z, Yin Z, Fan D, Wang J, Han G. The prognostic value of 18F-FDG PET/CT for hepatocellular carcinoma treated with transarterial chemoembolization (TACE). Theranostics 2014;4:736-44. [Crossref] [PubMed]
- Han JH, Kim DG, Na GH, Kim EY, Lee SH, Hong TH, You YK. Evaluation of prognostic factors on recurrence after curative resections for hepatocellular carcinoma. World J Gastroenterol 2014;20:17132-40. [Crossref] [PubMed]
- Takada Y, Kaido T, Shirabe K, Nagano H, Egawa H, Sugawara Y, et al. Significance of preoperative fluorodeoxyglucose-positron emission tomography in prediction of tumor recurrence after liver transplantation for hepatocellular carcinoma patients: a Japanese multicenter study. J Hepatobiliary Pancreat Sci 2017;24:49-57. [Crossref] [PubMed]
- Ling LL, Hsu CC, Yong CC, Elsarawy AM, Chan YC, Wang CC, Li WF, Lin TL, Kuo FY, Cheng YF, Lin LM, Chen CL, Lin CC. FDG-PET predicted unfavorable tumor histology in living donor liver transplant recipients; a retrospective cohort study. Int J Surg 2019;69:124-31. [Crossref] [PubMed]
- Lee JW, Hwang SH, Kim HJ, Kim D, Cho A, Yun M. Volumetric parameters on FDG PET can predict early intrahepatic recurrence-free survival in patients with hepatocellular carcinoma after curative surgical resection. Eur J Nucl Med Mol Imaging 2017;44:1984-94. [Crossref] [PubMed]
- Trojan J, Schroeder O, Raedle J, Baum RP, Herrmann G, Jacobi V, Zeuzem S. Fluorine-18 FDG positron emission tomography for imaging of hepatocellular carcinoma. Am J Gastroenterol 1999;94:3314-9. [Crossref] [PubMed]
- Takeuchi S, Rohren EM, Abdel-Wahab R, Xiao L, Morris JS, Macapinlac HA, Hassan MM, Kaseb AO. Refining prognosis in patients with hepatocellular carcinoma through incorporation of metabolic imaging biomarkers. Eur J Nucl Med Mol Imaging 2017;44:969-78. [Crossref] [PubMed]
- Lin CY, Chen JH, Liang JA, Lin CC, Jeng LB, Kao CH. 18F-FDG PET or PET/CT for detecting extrahepatic metastases or recurrent hepatocellular carcinoma: a systematic review and meta-analysis. Eur J Radiol 2012;81:2417-22.
- Lee JD, Yun M, Lee JM, Choi Y, Choi YH, Kim JS, Kim SJ, Kim KS, Yang WI, Park YN, Han KH, Lee WJ, Yoo N, Lim SM, Park JH. Analysis of gene expression profiles of hepatocellular carcinomas with regard to 18F-fluorodeoxyglucose uptake pattern on positron emission tomography. Eur J Nucl Med Mol Imaging 2004;31:1621-30. [Crossref] [PubMed]
- Kim MJ, Kim YS, Cho YH, Jang HY, Song JY, Lee SH, Jeong SW, Kim SG, Jang JY, Kim HS, Kim BS, Lee WH, Park JM, Lee JM, Lee MH, Choi DL. Use of (18)F-FDG PET to predict tumor progression and survival in patients with intermediate hepatocellular carcinoma treated by transarterial chemoembolization. Korean J Intern Med 2015;30:308-15. [Crossref] [PubMed]
- Lee JW, Na JO, Kang DY, Lee SY, Lee SM. Prognostic Significance of FDG Uptake of Bone Marrow on PET/CT in Patients With Non-Small-Cell Lung Cancer After Curative Surgical Resection. Clin Lung Cancer 2017;18:198-206. [Crossref] [PubMed]
- Jiang C, Zhao L, Xin B, Ma G, Wang X, Song S. (18)F-FDG PET/CT radiomic analysis for classifying and predicting microvascular invasion in hepatocellular carcinoma and intrahepatic cholangiocarcinoma. Quant Imaging Med Surg 2022;12:4135-50. [Crossref] [PubMed]
- Ahn SG, Jeon TJ, Lee SD, Kim SH, Cho HJ, Yun M, Park YN, Lee JD, Park SJ, Kim KS. A survival benefit of major hepatectomy for hepatocellular carcinoma identified by preoperative [18F] fluorodeoxyglucose positron emission tomography in patients with well-preserved hepatic function. Eur J Surg Oncol 2013;39:964-73.
- Kang YK, Choi JY, Paeng JC, Kim YI, Kwon HW, Cheon GJ, Suh KS, Kwon CHD, Lee DS, Kang KW. Composite criteria using clinical and FDG PET/CT factors for predicting recurrence of hepatocellular carcinoma after living donor liver transplantation. Eur Radiol 2019;29:6009-17. [Crossref] [PubMed]
- Lim C, Salloum C, Chalaye J, Lahat E, Costentin CE, Osseis M, Itti E, Feray C, Azoulay D. 18F-FDG PET/CT predicts microvascular invasion and early recurrence after liver resection for hepatocellular carcinoma: A prospective observational study. HPB (Oxford) 2019;21:739-47. [Crossref] [PubMed]
- Morio K, Kawaoka T, Aikata H, Namba M, Uchikawa S, Kodama K, Ohya K, Fujino H, Nakahara T, Murakami E, Yamauchi M, Tsuge M, Hiramatsu A, Imamura M, Nakamura Y, Akagi M, Awai K, Kobayashi T, Ohdan H, Chayama K. Preoperative PET-CT is useful for predicting recurrent extrahepatic metastasis of hepatocellular carcinoma after resection. Eur J Radiol 2020;124:108828. [Crossref] [PubMed]
- Wudel LJ Jr, Delbeke D, Morris D, Rice M, Washington MK, Shyr Y, Pinson CW, Chapman WC. The role of [18F]fluorodeoxyglucose positron emission tomography imaging in the evaluation of hepatocellular carcinoma. Am Surg 2003;69:117-24; discussion 124-6.
- Torizuka T, Tamaki N, Inokuma T, Magata Y, Sasayama S, Yonekura Y, Tanaka A, Yamaoka Y, Yamamoto K, Konishi J. In vivo assessment of glucose metabolism in hepatocellular carcinoma with FDG-PET. J Nucl Med 1995;36:1811-7.
- Leimgruber A, Hickson K, Lee ST, Gan HK, Cher LM, Sachinidis JI, O'Keefe GJ, Scott AM. Spatial and quantitative mapping of glycolysis and hypoxia in glioblastoma as a predictor of radiotherapy response and sites of relapse. Eur J Nucl Med Mol Imaging 2020;47:1476-85. [Crossref] [PubMed]
- Crispin-Ortuzar M, Apte A, Grkovski M, Oh JH, Lee NY, Schöder H, Humm JL, Deasy JO. Predicting hypoxia status using a combination of contrast-enhanced computed tomography and [18F]-Fluorodeoxyglucose positron emission tomography radiomics features. Radiother Oncol 2018;127:36-42.
- Beer AJ, Lorenzen S, Metz S, Herrmann K, Watzlowik P, Wester HJ, Peschel C, Lordick F, Schwaiger M. Comparison of integrin alphaVbeta3 expression and glucose metabolism in primary and metastatic lesions in cancer patients: a PET study using 18F-galacto-RGD and 18F-FDG. J Nucl Med 2008;49:22-9. [Crossref] [PubMed]
- Clavo AC, Brown RS, Wahl RL. Fluorodeoxyglucose uptake in human cancer cell lines is increased by hypoxia. J Nucl Med 1995;36:1625-32.
- Burgman P, Odonoghue JA, Humm JL, Ling CC. Hypoxia-Induced increase in FDG uptake in MCF7 cells. J Nucl Med 2001;42:170-5.
- Dirckx N, Tower RJ, Mercken EM, Vangoitsenhoven R, Moreau-Triby C, Breugelmans T, Nefyodova E, Cardoen R, Mathieu C, Van der Schueren B, Confavreux CB, Clemens TL, Maes C. Vhl deletion in osteoblasts boosts cellular glycolysis and improves global glucose metabolism. J Clin Invest 2018;128:1087-105. [Crossref] [PubMed]
- Hwang SH, Lee M, Lee N, Park S, Kim CK, Park MA, Yun M. Increased 18F-FDG Uptake on PET/CT is Associated With Poor Arterial and Portal Perfusion on Multiphase CT. Clin Nucl Med 2016;41:296-301. [Crossref] [PubMed]
- Yamamoto K, Brender JR, Seki T, Kishimoto S, Oshima N, Choudhuri R, Adler SS, Jagoda EM, Saito K, Devasahayam N, Choyke PL, Mitchell JB, Krishna MC. Molecular Imaging of the Tumor Microenvironment Reveals the Relationship between Tumor Oxygenation, Glucose Uptake, and Glycolysis in Pancreatic Ductal Adenocarcinoma. Cancer Res 2020;80:2087-93. [Crossref] [PubMed]
- Kaira K, Shimizu K, Kitahara S, Yajima T, Atsumi J, Kosaka T, Ohtaki Y, Higuchi T, Oyama T, Asao T, Mogi A. 2-Deoxy-2-[fluorine-18] fluoro-d-glucose uptake on positron emission tomography is associated with programmed death ligand-1 expression in patients with pulmonary adenocarcinoma. Eur J Cancer 2018;101:181-90.
- Yao Y, Li YM, He ZX, Civelek AC, Li XF. Likely Common Role of Hypoxia in Driving (18)F-FDG Uptake in Cancer, Myocardial Ischemia, Inflammation and Infection. Cancer Biother Radiopharm 2021;36:624-31. [Crossref] [PubMed]
- Cao Y, Zhou K, Diao W, Long X, Tian F, Su M, Jia Z. Age-related changes of standardized uptake values in the blood pool and liver: a decade-long retrospective study of the outcomes of 2,526 subjects. Quant Imaging Med Surg 2021;11:95-106. [Crossref] [PubMed]
- Wang R, Fan Q, Tian R, Su M. Intrapatient repeatability of background (18)F-FDG uptake on PET/CT. Quant Imaging Med Surg 2021;11:4172-80. [Crossref] [PubMed]
- Matsui T, Nakata N, Nagai S, Nakatani A, Takahashi M, Momose T, Ohtomo K, Koyasu S. Inflammatory cytokines and hypoxia contribute to 18F-FDG uptake by cells involved in pannus formation in rheumatoid arthritis. J Nucl Med 2009;50:920-6. [Crossref] [PubMed]
- Payen VL, Brisson L, Dewhirst MW, Sonveaux P. Common responses of tumors and wounds to hypoxia. Cancer J 2015;21:75-87. [Crossref] [PubMed]
- Cheng Y, Chen G, Hong L, Zhou L, Hu M, Li B, Huang J, Xia L, Li C. How does hypoxia inducible factor-1α participate in enhancing the glycolysis activity in cervical cancer? Ann Diagn Pathol 2013;17:305-11. [Crossref] [PubMed]
- Airley RE, Mobasheri A. Hypoxic regulation of glucose transport, anaerobic metabolism and angiogenesis in cancer: novel pathways and targets for anticancer therapeutics. Chemotherapy 2007;53:233-56. [Crossref] [PubMed]
- Wyatt E, Wu R, Rabeh W, Park HW, Ghanefar M, Ardehali H. Regulation and cytoprotective role of hexokinase III. PLoS One 2010;5:e13823. [Crossref] [PubMed]
- Lee M, Jeon JY, Neugent ML, Kim JW, Yun M. 18F-Fluorodeoxyglucose uptake on positron emission tomography/computed tomography is associated with metastasis and epithelial-mesenchymal transition in hepatocellular carcinoma. Clin Exp Metastasis 2017;34:251-60. [Crossref] [PubMed]
- Chen R, Li J, Zhou X, Liu J, Huang G. Fructose-1,6-Bisphosphatase 1 Reduces (18)F FDG Uptake in Hepatocellular Carcinoma. Radiology 2017;284:844-53. [Crossref] [PubMed]
- Huang Y, Ge W, Zhou J, Gao B, Qian X, Wang W. The Role of Tumor Associated Macrophages in Hepatocellular Carcinoma. J Cancer 2021;12:1284-94. [Crossref] [PubMed]
- Jeong H, Kim S, Hong BJ, Lee CJ, Kim YE, Bok S, et al. Tumor-Associated Macrophages Enhance Tumor Hypoxia and Aerobic Glycolysis. Cancer Res 2019;79:795-806. [Crossref] [PubMed]
- Reinfeld BI, Madden MZ, Wolf MM, Chytil A, Bader JE, Patterson AR, et al. Cell-programmed nutrient partitioning in the tumour microenvironment. Nature 2021;593:282-8. [Crossref] [PubMed]
- Jo H, Lee J, Jeon J, Kim SY, Chung JI, Ko HY, Lee M, Yun M. The critical role of glucose deprivation in epithelial-mesenchymal transition in hepatocellular carcinoma under hypoxia. Sci Rep 2020;10:1538. [Crossref] [PubMed]
- Jo IY, Son SH, Kim M, Sung SY, Won YK, Kang HJ, Lee SJ, Chung YA, Oh JK, Kay CS. Prognostic value of pretreatment (18)F-FDG PET-CT in radiotherapy for patients with hepatocellular carcinoma. Radiat Oncol J 2015;33:179-87. [Crossref] [PubMed]