Imaging features of cardioembolic stroke on 4-dimensional computed tomography angiography
Introduction
Cardioembolic stroke is one of the 5 subtypes of acute ischemic stroke (AIS), accounting for approximately 27% of all strokes (1) according to the international Trial of ORG 10172 in Acute Stroke Treatment (TOAST) criteria (2). Cardioembolic stroke has more severe clinical symptoms and a higher stroke recurrence rate (3), as well as a poorer collateral status, than other stroke etiologies (4). As for treatment, in a previous study, the reperfusion success rate and the complete reperfusion rate after a single thrombectomy pass were higher with endovascular therapy for cardioembolic large-vessel occlusion than they were for other stroke types (5). Moreover, the secondary prevention therapies for cardioembolic stroke differ from those of other etiologies (6). Therefore, identification of cardioembolic stroke in the early stage has an essential influence on the endovascular treatment decision-making and the use of future anticoagulation therapy.
In addition to neurologic symptoms and brain imaging findings, the diagnosis of cardioembolic stroke requires the identification of at least 1 heart disease and associated risk factors. However, in emergency situations, more heart-related tests could be avoided by analyzing differences in imaging findings to identify cardioembolic stroke. The image characteristics of cardioembolic stroke differ from those of other types of stroke (7-9) due to differences in pathogenesis. A recent study showed that on computed tomography (CT) angiography (CTA), the features of clot including shorter clot and a higher clot burden score (CBS) were related to cardioembolic stroke (10). Furthermore, in a previous study, patients with cardioembolic stroke tended to have worse collateral flow compared with large artery atherosclerosis stroke (11). These imaging features have been used to distinguish cardioembolic stroke from non-cardioembolic stroke. For example, according to a previous study (12), the presence of the multi-segment clot sign on dynamic CTA indicates the type of extensive artery atherosclerosis.
In this study, as well as the traditional post-processing maximum intensity projection (MIP) reconstruction on 4-dimensional (4D)-CTA, a novel reconstruction method was utilized to observe clot characteristics. The time MIP (tMIP) is an innovative post-processing method that can be used to collect data from several phases of CT perfusion to generate new volume data. It accurately reflects the maximum values on all selected sequences (13) and displays the collateral conditions. In a previous study (14), tMIP had a better signal-to-noise ratio than traditional MIP. In this study, we explored the clot features of cardioembolic stroke using 4D-CTA MIP/tMIP images and assessed whether these features could assist in classifying stroke etiology. We present this article in accordance with the STARD reporting checklist (available at https://qims.amegroups.com/article/view/10.21037/qims-23-120/rc).
Methods
The study was conducted in accordance with the Declaration of Helsinki (as revised in 2013), and approved by the Human Ethics Committee of the First Affiliated Hospital of Chongqing Medical University (protocol code, 2021-274; date of approval June 6, 2021). Due to the retrospective design of the study, informed consent was not required.
Patients
The data of 294 patients with AIS who attended the First Affiliated Hospital of Chongqing Medical University from July 2020 to February 2022 were consecutively collected. Before clinical intervention, all patients underwent 4D-CTA/CT perfusion scanning. The inclusion criteria were as follows: (I) CTA showing occlusion of the M1/M2 segment of the middle cerebral artery (MCA) with/without intracranial internal carotid artery (ICA) occlusion and (II) complete image and clinical data. The exclusion criteria were as follows: (I) poor image quality and (II) the presence of other intercranial diseases, such as tumors or trauma.
CT protocol
All images were obtained using a 320-row detector CT scanner (Aquilion ONE, Canon Medical Systems Corp., Otawara, Japan). Whole-brain dynamic volume intermittent scanning (2-second interval in the arterial phase, 5-second in the venous phase) for CT perfusion/4D-CTA was performed after non-contrast-enhanced CT. The scan parameters were as follows: 80 kV, 150–310 mA, coverage of 140–160 mm, reconstruction with an adaptive iterative dose reduction 3-dimensional (3D) algorithm, slice thickness of 1.0 mm, and an interval of 1.0 mm to improve the reconstructive speed. Contrast agent was injected using a high-pressure syringe with P3T technology, and the injection speed and quantity were calculated automatically according to the patient’s sex, weight, and height (MEDRAD Stellant CT Injection System, Bayer Medical Care, Pittsburgh, KS, USA).
Image post-processing
A total of 19 phases with 3,040 frames of whole-brain images were collected. The arterial phase was selected according to the perfusion curves, and MIP maps were reconstructed. The tMIP images were reconstructed using images from the latter 17 phases, and both conventional MIP and tMIP images were reconstructed with a slice thickness of 5 mm (Figure 1).
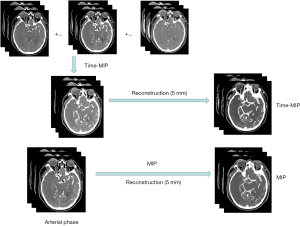
Clinical and image evaluation
Patients’ clinical data were extracted from the electronic medical records system, including data on sex, age, cerebrovascular disease risk factors (smoking history, hypertension, coronary heart disease, diabetes mellitus, dyslipidemia), stroke subtypes, and high/medium-risk sources of cardioembolic stroke (atrial fibrillation, mechanical prosthetic valve, left atrial/atrial appendage thrombus, sick sinus syndrome, dilated cardiomyopathy, recent myocardial infarction, patent foramen ovale, congestive heart failure). The patients were divided into the cardioembolic stroke and non-cardioembolic stroke (including large-artery atherosclerosis, stroke of other determined etiology, and stroke of undetermined etiology) groups according to the TOAST criteria.
When collating clinical data, the TOAST classification was checked by neuroradiologists against the standard. The images were observed and analyzed by 2 neuroradiologists with more than 8 years of experience in neuroimaging, who also recorded the data, including clot length, and summed the CBS and collateral score (CS) based on the images (Figure 2). In cases of disagreement, another blinded expert neuroradiologist with more than 10 years of experience in neuroimaging performed the evaluation.
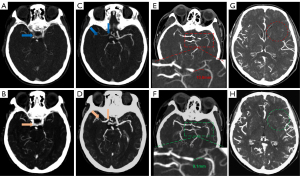
Clot length
The site of the intravascular contrast filling defect was considered to represent the clot location. When measuring the clot length, the section (axis or coronal) in which the clot was best displayed was selected.
CBS
The CBS has a total possible score of 10 (15). If there was occlusion of the supraclinoid ICA or the proximal or distal part of the M1 segment of the MCA, 2 points were subtracted. If the clot involved the infraclinoid ICA, the A1 segment of the anterior cerebral artery, or any branch of the M2 segment of the MCA, 1 point was subtracted. A lower score suggests a longer arterial filling defect (16). A CBS of 0–6 represents a long clot, whereas a CBS of 7–10 represents a short clot.
CS
We used the Tan collateral grading system (17), which can be defined as follows: 0= absent collaterals, 1= collaterals filling ≤50% of the ischemic region, 2= collaterals filling >50% and <100% of the ischemic region, and 3= collaterals filling 100% of the ischemic region.
Statistical analysis
Categorical data were presented as absolute numbers and proportions, whereas continuous data were presented as the median with interquartile range (IQR). The distribution normality was assessed using the Shapiro-Wilk test, and the F test was used to test the homogeneity of variance. Differences between indicators and stroke types were examined using the Mann-Whitney U test. To assess the association between the 4D-CTA findings and stroke etiology, a binary logistic regression was performed. Finally, the receiver operating characteristic (ROC) curve analysis was performed to test the efficiency of identifying cardioembolic stroke. We also obtained the Youden index to determine the optimal cut-off thresholds to identify cardioembolic stroke.
All statistical analyses were performed using the software SPSS 26.0 (IBM Corp., Armonk, NY, USA), and MedCalc, version 20.0.22 (MedCalc, Mariakerke, Belgium). A P value of <0.05 was considered statistically significant.
Results
Among the 294 patients with AIS due to anterior-circulation artery occlusion, 151 patients were screened for MCA M1/M2 segment occlusion with or without intracranial ICA occlusion. A total of 26 patients were excluded because of incomplete image data or significant artifacts, and 4 patients were excluded because the occlusion was not the cause of stroke. A further 11 patients were excluded due to lack of clinical documentation. Finally, the data of 110 patients were included to calculate the CBS and CS. Of these, the data of 88 patients were further assessed to determine thrombus length (Figure 3).
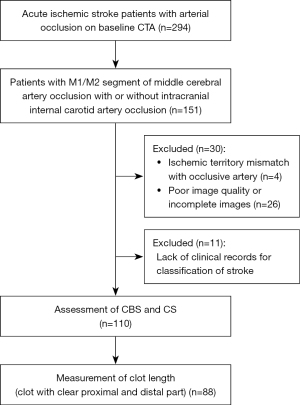
A total of 54 patients were included in the cardioembolic stroke group, and 56 patients were included in the non-cardioembolic stroke group. The median age of the patients in the cardioembolic stroke group was 74 years (IQR, 66–81 years), whereas the median age of the patients in the non-cardioembolic stroke group was 65 years (IQR, 56–76.5 years). The differences in baseline clinical characteristics between the 2 groups are shown in Table 1. Among the risk factors, patients with hypertension, coronary heart disease, atrial fibrillation, and congestive heart failure showed significant differences in cardioembolic stroke classification (P<0.05), especially atrial fibrillation. The clot length was measured in 39 patients in the cardioembolic stroke group and in 49 patients in the non-cardioembolic stroke group. In the cardioembolic stroke group, the median MIP and tMIP clot length was 12.0 mm (IQR, 8.3–17.4 mm) and 9.3 mm (IQR, 6.8–14.3 mm), respectively, whereas in the non-cardioembolic stroke group, the median MIP and tMIP clot length was 6.5 mm (IQR, 4.7–11.5 mm) and 5.8 mm (IQR, 3.9–10.6 mm), respectively. The non-parametric test showed that clot length, CBS, and CS were significantly different between the 2 groups (Table 2).
Table 1
Variables | Cardioembolic stroke (n=54) | Non-cardioembolic stroke (n=56) | z | P value |
---|---|---|---|---|
Age (years), median [IQR] | 74 [66–81] | 65 [56–76.5] | −2.96 | <0.05* |
Male, n (%) | 25 (46.3) | 35 (62.5) | – | 0.09 |
Smoking, n (%) | 18 (33.3) | 28 (50.0) | – | 0.08 |
Hypertension, n (%) | 32 (59.3) | 43 (76.8) | – | <0.05* |
Coronary heart disease, n (%) | 11 (20.4) | 3 (5.4) | – | <0.05* |
Diabetes mellitus, n (%) | 18 (33.3) | 18 (32.1) | – | 0.89 |
Dyslipidemia, n (%) | 6 (11.1) | 14 (25.0) | – | 0.06 |
High-risk sources of cardioembolic stroke, n (%) | ||||
Atrial fibrillation (other than lone atrial fibrillation) | 40 (74.1) | 7 (12.5) | – | <0.05* |
Mechanical prosthetic valve | 2 (3.7) | 0 | – | 0.15 |
Left atrial/atrial appendage thrombus | 2 (3.7) | 0 | – | 0.15 |
Sick sinus syndrome | 1 (1.9) | 0 | – | 0.31 |
Dilated cardiomyopathy | 2 (3.7) | 0 | – | 0.15 |
Recent myocardial infarction (<4 weeks) | 1 (1.9) | 0 | – | 0.31 |
Medium-risk sources of cardioembolic stroke, n (%) | ||||
Patent foramen ovale | 1 (1.9) | 0 | – | 0.31 |
Congestive heart failure | 15 (27.8) | 0 | – | <0.05* |
*, statistically significant P value. IQR, interquartile range.
Table 2
Variables | Cardioembolic stroke | Non-cardioembolic stroke | z | P value |
---|---|---|---|---|
MIP-CS, n (%) | N=54 | N=56 | −3.39 | <0.05* |
0 | 11 (20.4) | 5 (8.9) | ||
1 | 34 (63.0) | 26 (46.4) | ||
2 | 9 (16.7) | 18 (32.1) | ||
3 | 0 (0) | 7 (12.5) | ||
tMIP-CS, n (%) | N=54 | N=56 | −2.43 | <0.05* |
0 | 2 (3.7) | 0 (0) | ||
1 | 20 (37.0) | 16 (28.6) | ||
2 | 30 (55.6) | 27 (48.2) | ||
3 | 2 (3.7) | 13 (23.2) | ||
MIP-CBS, n (%) | N=54 | N=56 | −2.87 | <0.05* |
0–6 | 29 (53.7) | 15 (26.8) | ||
7–10 | 25 (46.3) | 41 (73.2) | ||
Clot length†, median [IQR] | N=39 | N=49 | ||
MIP-clot length (mm) | 12.0 [8.3–17.4] | 6.5 [4.7–11.5] | −4.08 | <0.05* |
tMIP-clot length (mm) | 9.3 [6.8–14.3] | 5.8 [3.9–10.6] | −3.51 | <0.05* |
*, statistically significant P value; †, data available from 88 patients. MIP, maximum intensity projection; CS, collateral score; tMIP, time maximum intensity projection; CBS, clot burden score; IQR, interquartile range.
Binary logistic regression showed that cardioembolic stroke was significantly associated with MIP-clot length [odds ratio (OR), 1.15; 95% confidence interval (CI): 1.02–1.29; P<0.05], tMIP-clot length (OR, 1.18; 95% CI: 1.02–1.36; P<0.05), and tMIP-CBS (OR, 3.96; 95% CI: 1.08–14.58; P<0.05), when setting non-cardioembolic stroke as the reference, after adjusting for age, hypertension, coronary heart disease, atrial fibrillation, and congestive heart failure (Table 3).
Table 3
Variables | OR | 95% CI | P value |
---|---|---|---|
MIP-clot length† | 1.15 | 1.02–1.29 | <0.05* |
tMIP-clot length† | 1.18 | 1.02–1.36 | <0.05* |
MIP-CBS | 2.77 | 0.94–8.16 | 0.07 |
MIP-CS | 1.72 | 0.55–5.37 | 0.35 |
tMIP-CBS | 3.96 | 1.08–14.58 | <0.05* |
tMIP-CS | 0.35 | 0.10–1.30 | 0.12 |
*, statistically significant P value; †, data available from 88 patients. OR, odds ratio; CI, confidence interval; MIP, maximum intensity projection; tMIP, time maximum intensity projection; CBS, clot burden score; CS, collateral score.
The ROC curve analyses were performed for MIP/tMIP-clot length, and tMIP-CBS, which were found to be significantly associated with cardioembolic stroke (Figure 4, Table 4). The area under the ROC curve (AUC) values of the MIP and tMIP clot length for identifying cardioembolic stroke were 0.75 (95% CI: 0.65–0.84, P<0.05) and 0.72 (95% CI: 0.61–0.81, P<0.05), respectively. The AUC value of tMIP CBS was 0.59 (95% CI: 0.50–0.69, P=0.07), and the difference between the AUC of tMIP-CBS and that of MIP/tMIP-clot length was statistically significant.
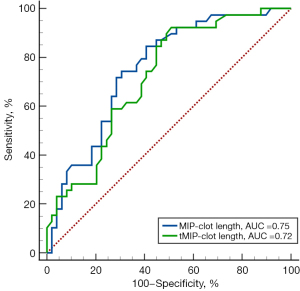
Table 4
Variables | Clot length (n=88) | CBS (n=110) | ||
---|---|---|---|---|
MIP | tMIP | tMIP | ||
AUC | 0.75 (0.65–0.84) | 0.72 (0.61–0.81) | 0.59 (0.50–0.69) | |
Sensitivity (%) | 84.62 (69.50–94.10) | 92.31 (79.10–98.40) | 35.19 (22.70–49.40) | |
Specificity (%) | 59.18 (44.20–73.00) | 48.98 (34.40–63.70) | 85.71 (73.80–93.60) | |
Youden index | 0.44 | 0.41 | 0.21 | |
Cut-off value | >7.4 | >5.4 | ≤6 |
ROC, receiver operating characteristic; CBS, clot burden score; MIP, maximum intensity projection; tMIP, time maximum intensity projection; AUC, area under the curve.
According to the ROC curve analysis, a cut-off value of >7.4 mm for MIP clot length [sensitivity: 84.62% (95% CI: 69.50–94.10%); specificity: 59.18% (95% CI: 44.20–73.00%)] and a cut-off value of >5.4 mm for tMIP clot length [sensitivity: 92.31% (95% CI: 79.10–98.40%); specificity: 48.98% (95% CI: 34.40–63.70%)] were able to categorize a thrombus as being of cardioembolic origin.
Discussion
The present study has several key findings. First, the optimal indicator for the identification of cardioembolic stroke was clot length, with AUC values of 0.75/0.72. Second, compared with tMIP, the clot length obtained by traditional MIP reconstruction was longer, and the CBS was lower, which suggested that MIP overestimated the clot burden to a certain extent. Third, the cardioembolism clot length was longer than the non-cardioembolism clot length, and the proportion of patients in the cardioembolic stroke group with poor collateral circulation and a CBS of <6 was higher than the proportion of patients in the non-cardioembolic stroke group.
In addition to clot length, CBS, and CS, several other clot imaging characteristics have been used to identify stroke subtypes. For example, Kufner et al. (18) used thrombus permeability to identify cardioembolic stroke. An AUC value of 0.75 was obtained, which was no different to the AUC value for clot length in the present study. However, the process of clot segmentation is complicated compared with the measurement of clot length. Moreover, patients with involvement of the proximal M2 segment of the MCA were included in this study. Clot length may be a simple, fast, and widely available method in the emergency setting. In terms of the CS, a previous study (19) revealed that collaterals on CT perfusion images showed an excellent ability to identify the stroke classification with an AUC value of 0.87, which is higher than the AUC value observed in the present study. The reason for this discrepancy may lie in the influence of vascular variation, slow blood flow, and leukoaraiosis on the collateral circulation (20).
In the present study, the clot features on 4D-CTA differed between diverse stroke etiologies and post-processing methods. The clot length was shorter and the CBS was higher on tMIP images. This phenomenon may be related to delayed contrast filling and antegrade/retrograde blood flow. MIP images were based on the arterial phase, whereas tMIP images reflected all chosen phases, including phases after the peak arterial phase. Thus, it is reasonable to speculate that the difference between MIP clot length and tMIP clot length was caused by delayed contrast filling. Zhou et al. (21) measured the length of delayed contrast filling on 4D-CTA to classify stroke. The length of delayed contrast filling was determined by the maximum distance between the proximal occlusion site at the peak arterial phase and the late phase. The authors concluded that the more extended segment of delayed contrast filling had good diagnostic efficacy for classifying cardioembolic stroke. When the delayed contrast filling length was >1.5 mm, the sensitivity and specificity for identifying cardioembolic stroke were 83.2% and 70.8%, respectively. This finding was consistent with our results. The difference between tMIP clot length and MIP clot length was 2.7 mm in the cardioembolic stroke group, which was greater than the difference in the non-cardioembolic stroke group (0.7 mm). Notably, clot length, location, and CBS have important effects on thrombolytic therapy, stent selection, and endovascular treatment (22-25). Some patients demonstrated an increase in the CBS when assessed using tMIP images, implying that there may be an overestimation of thrombus severity level when using MIP images. Smaller thrombi are associated with better neurological outcomes at 90 days (26); therefore, accurate and quantitative evaluation of clot length and CBS is necessary.
Interestingly, there existed an interaction between the collateral circulation and clot length. This could explain our observation showing that the clot length in patients with cardioembolic stroke was longer than that in non-cardioembolic stroke patients. Qazi et al. (27) interpreted that the thrombus was composed of in-situ and new thrombi formed by surrounding red blood cell stasis. Poor collateral circulation and lack of small arteries around occluded segments may lead to more extended thrombus formation, and slow collateral flow also has an impact on clot length.
A recent CT-based radiomics study (28) found that the diagnostic effectiveness of clot features extracted by radiomics methods in predicting cardioembolic stroke was superior to that of radiological clot features. This result emphasized that radiomic features could help to optimize the clot characteristic assessment. However, in the process of clot segmentation, in order to avoid overestimation of clot length on CTA images, researchers used a combination of CTA and digital subtraction angiography to confirm the proximal and distal part of the clot. The tMIP post-processing method we adopted might be used for precise segmentation of clot in the future, thereby further utilizing radiomics to identify cardioembolic stroke.
This study had some limitations that should be noted. First, the sample size was small. Some patients were excluded because vascular shadows overlapped in the thrombus segment, especially on tMIP images. Second, this study was a single-center, retrospective study, and selection bias was apparent when screening patients. In the future, we will include patients with anterior and posterior cerebral artery thrombi to expand the sample size. Finally, the history of anticoagulant and antiplatelet medication, which can influence the clot features, was not considered.
Conclusions
Our study confirmed that clot length could identify cardioembolic stroke and provide imaging clues to classify stroke etiology. The tMIP technique improved the overestimation of clot load that was apparent with the MIP technique, thus providing more accurate results.
Acknowledgments
We would like to thank our imaging technicians and colleagues from the neurology departments for their support in making this study possible.
Funding: None.
Footnote
Reporting Checklist: The authors have completed the STARD reporting checklist. Available at https://qims.amegroups.com/article/view/10.21037/qims-23-120/rc
Conflicts of Interest: All authors have completed the ICMJE uniform disclosure form (available at https://qims.amegroups.com/article/view/10.21037/qims-23-120/coif). The authors have no conflicts of interest to declare.
Ethical Statement: The authors are accountable for all aspects of the work in ensuring that questions related to the accuracy or integrity of any part of the work are appropriately investigated and resolved. The study was conducted in accordance with the Declaration of Helsinki (as revised in 2013) and approved by the Human Ethics Committee of the First Affiliated Hospital of Chongqing Medical University (protocol code 2021-274; date of approval was June 6, 2021). Due to the retrospective design of the study, informed consent was not required.
Open Access Statement: This is an Open Access article distributed in accordance with the Creative Commons Attribution-NonCommercial-NoDerivs 4.0 International License (CC BY-NC-ND 4.0), which permits the non-commercial replication and distribution of the article with the strict proviso that no changes or edits are made and the original work is properly cited (including links to both the formal publication through the relevant DOI and the license). See: https://creativecommons.org/licenses/by-nc-nd/4.0/.
References
- Kolominsky-Rabas PL, Weber M, Gefeller O, Neundoerfer B, Heuschmann PU. Epidemiology of ischemic stroke subtypes according to TOAST criteria: incidence, recurrence, and long-term survival in ischemic stroke subtypes: a population-based study. Stroke 2001;32:2735-40. [Crossref] [PubMed]
- Adams HP Jr, Bendixen BH, Kappelle LJ, Biller J, Love BB, Gordon DL, Marsh EE 3rd. Classification of subtype of acute ischemic stroke. Definitions for use in a multicenter clinical trial. TOAST. Trial of Org 10172 in Acute Stroke Treatment. Stroke 1993;24:35-41. [Crossref] [PubMed]
- Flach C, Muruet W, Wolfe CDA, Bhalla A, Douiri A. Risk and Secondary Prevention of Stroke Recurrence: A Population-Base Cohort Study. Stroke 2020;51:2435-44. [Crossref] [PubMed]
- Guglielmi V, LeCouffe NE, Zinkstok SM, Compagne KCJ, Eker R, Treurniet KM, Tolhuisen ML, van der Worp HB, Jansen IGH, van Oostenbrugge RJ, Marquering HA, Dippel DWJ, Emmer BJ, Majoie CBLM, Roos YBWEM, Coutinho JMMR-CLEAN Registry Investigators. Collateral Circulation and Outcome in Atherosclerotic Versus Cardioembolic Cerebral Large Vessel Occlusion. Stroke 2019;50:3360-8. [Crossref] [PubMed]
- Tiedt S, Herzberg M, Küpper C, Feil K, Kellert L, Dorn F, Liebig T, Alegiani A, Dichgans M, Wollenweber FA, Investigators GSR. Stroke Etiology Modifies the Effect of Endovascular Treatment in Acute Stroke. Stroke 2020;51:1014-6. [Crossref] [PubMed]
- Kernan WN, Ovbiagele B, Black HR, Bravata DM, Chimowitz MI, Ezekowitz MD, Fang MC, Fisher M, Furie KL, Heck DV, Johnston SC, Kasner SE, Kittner SJ, Mitchell PH, Rich MW, Richardson D, Schwamm LH, Wilson JAAmerican Heart Association Stroke Council, Council on Cardiovascular and Stroke Nursing, Council on Clinical Cardiology, and Council on Peripheral Vascular Disease. Guidelines for the prevention of stroke in patients with stroke and transient ischemic attack: a guideline for healthcare professionals from the American Heart Association/American Stroke Association. Stroke 2014;45:2160-236. [Crossref] [PubMed]
- Brinjikji W, Duffy S, Burrows A, Hacke W, Liebeskind D, Majoie CBLM, Dippel DWJ, Siddiqui AH, Khatri P, Baxter B, Nogeuira R, Gounis M, Jovin T, Kallmes DF. Correlation of imaging and histopathology of thrombi in acute ischemic stroke with etiology and outcome: a systematic review. J Neurointerv Surg 2017;9:529-34. [Crossref] [PubMed]
- Patel TR, Fricano S, Waqas M, Tso M, Dmytriw AA, Mokin M, Kolega J, Tomaszewski J, Levy EI, Davies JM, Snyder KV, Siddiqui AH, Tutino VM. Increased Perviousness on CT for Acute Ischemic Stroke is Associated with Fibrin/Platelet-Rich Clots. AJNR Am J Neuroradiol 2021;42:57-64. [Crossref] [PubMed]
- Fitzgerald S, Wang S, Dai D, Murphree DH Jr, Pandit A, Douglas A, Rizvi A, Kadirvel R, Gilvarry M, McCarthy R, Stritt M, Gounis MJ, Brinjikji W, Kallmes DF, Doyle KM. Orbit image analysis machine learning software can be used for the histological quantification of acute ischemic stroke blood clots. PLoS One 2019;14:e0225841. [Crossref] [PubMed]
- Boodt N, Compagne KCJ, Dutra BG, Samuels N, Tolhuisen ML, Alves HCBR, Kappelhof M, Lycklama À, Nijeholt GJ, Marquering HA. Majoie CBLM, Lingsma HF, Dippel DWJ, van der Lugt A; Coinvestigators MR CLEAN Registry. Stroke Etiology and Thrombus Computed Tomography Characteristics in Patients With Acute Ischemic Stroke: A MR CLEAN Registry Substudy. Stroke 2020;51:1727-35. [Crossref] [PubMed]
- Rebello LC, Bouslama M, Haussen DC, Grossberg JA, Dehkharghani S, Anderson A, Belagaje SR, Bianchi NA, Grigoryan M, Frankel MR, Nogueira RG. Stroke etiology and collaterals: atheroembolic strokes have greater collateral recruitment than cardioembolic strokes. Eur J Neurol 2017;24:762-7. [Crossref] [PubMed]
- Chen Z, Shi F, Zhang M, Gong X, Lin L, Lou M. Prediction of the Multisegment Clot Sign on Dynamic CT Angiography of Cardioembolic Stroke. AJNR Am J Neuroradiol 2018;39:663-8. [Crossref] [PubMed]
- Murayama K, Suzuki S, Matsukiyo R, Takenaka A, Hayakawa M, Tsutsumi T, Fujii K, Katada K, Toyama H. Preliminary study of time maximum intensity projection computed tomography imaging for the detection of early ischemic change in patient with acute ischemic stroke. Medicine (Baltimore) 2018;97:e9906. [Crossref] [PubMed]
- Cao R, Jiang Y, Lu J, Wu G, Zhang L, Chen J. Evaluation of Intracranial Vascular Status in Patients with Acute Ischemic Stroke by Time Maximum Intensity Projection CT Angiography: A Preliminary Study. Acad Radiol 2020;27:696-703. [Crossref] [PubMed]
- Puetz V, Dzialowski I, Hill MD, Subramaniam S, Sylaja PN, Krol A, O'Reilly C, Hudon ME, Hu WY, Coutts SB, Barber PA, Watson T, Roy J, Demchuk AMCalgary CTA Study Group. Intracranial thrombus extent predicts clinical outcome, final infarct size and hemorrhagic transformation in ischemic stroke: the clot burden score. Int J Stroke 2008;3:230-6. [Crossref] [PubMed]
- Derraz I, Bourcier R, Soudant M, Soize S, Hassen WB, Hossu G, Clarencon F, Derelle AL, Tisserand M, Raoult H, Legrand L, Bracard S, Oppenheim C, Naggara O. THRACE Investigators. Does Clot Burden Score on Baseline T2*-MRI Impact Clinical Outcome in Acute Ischemic Stroke Treated with Mechanical Thrombectomy? J Stroke 2019;21:91-100. [Crossref] [PubMed]
- Tan BY, Wan-Yee K, Paliwal P, Gopinathan A, Nadarajah M, Ting E, Venketasubramanian N, Seet RC, Chan BP, Teoh HL, Rathakrishnan R, Sharma VK, Yeo LL. Good Intracranial Collaterals Trump Poor ASPECTS (Alberta Stroke Program Early CT Score) for Intravenous Thrombolysis in Anterior Circulation Acute Ischemic Stroke. Stroke 2016;47:2292-8. [Crossref] [PubMed]
- Kufner A, Erdur H, Endres M, Nolte CH, Scheel M, Schlemm L. Association Between Thrombus Perviousness Assessed on Computed Tomography and Stroke Cause. Stroke 2020;51:3613-22. [Crossref] [PubMed]
- Shi F, Zeng Q, Gong X, Zhong W, Chen Z, Yan S, Lou M. Quantitative Collateral Assessment on CTP in the Prediction of Stroke Etiology. AJNR Am J Neuroradiol 2022;43:966-71. [Crossref] [PubMed]
- Hashimoto T, Kunieda T, Honda T, Scalzo F, Ali L, Hinman JD, Rao NM, Nour M, Bahr-Hosseini M, Saver JL, Raychev R, Liebeskind D. Reduced Leukoaraiosis, Noncardiac Embolic Stroke Etiology, and Shorter Thrombus Length Indicate Good Leptomeningeal Collateral Flow in Embolic Large-Vessel Occlusion. AJNR Am J Neuroradiol 2022;43:63-9. [Crossref] [PubMed]
- Zhou Y, Xu C, Zhang R, Shi F, Liu C, Yan S, Ding X, Zhang M, Lou M. Longer Length of Delayed-Contrast Filling of Clot on 4-Dimensional Computed Tomographic Angiography Predicts Cardiogenic Embolism. Stroke 2019;50:2568-70. [Crossref] [PubMed]
- Hofmeister J, Bernava G, Rosi A, Vargas MI, Carrera E, Montet X, Burgermeister S, Poletti PA, Platon A, Lovblad KO, Machi P. Clot-Based Radiomics Predict a Mechanical Thrombectomy Strategy for Successful Recanalization in Acute Ischemic Stroke. Stroke 2020;51:2488-94. [Crossref] [PubMed]
- Rossi R, Fitzgerald S, Gil SM, Mereuta OM, Douglas A, Pandit A, et al. Correlation between acute ischaemic stroke clot length before mechanical thrombectomy and extracted clot area: Impact of thrombus size on number of passes for clot removal and final recanalization. Eur Stroke J 2021;6:254-61. [Crossref] [PubMed]
- Baek JH, Kim BM, Yoo J, Nam HS, Kim YD, Kim DJ, Heo JH, Bang OY. Predictive Value of Computed Tomography Angiography-Determined Occlusion Type in Stent Retriever Thrombectomy. Stroke 2017;48:2746-52. [Crossref] [PubMed]
- Riou-Comte N, Gory B, Soudant M, Zhu F, Xie Y, Humbertjean L, Mione G, Oppenheim C, Guillemin F, Bracard S, Richard STHRACE investigators. Clinical imaging factors of excellent outcome after thrombolysis in large-vessel stroke: a THRACE subgroup analysis. Stroke Vasc Neurol 2021;6:631-9. [Crossref] [PubMed]
- Dutra BG, Tolhuisen ML, Alves HCBR, Treurniet KM, Kappelhof M, Yoo AJ, Jansen IGH, Dippel DWJ, van Zwam WH, van Oostenbrugge RJ, da Rocha AJ, Lingsma HF, van der Lugt A, Roos YBWEM, Marquering HA, Majoie CBLM. MR CLEAN Registry Investigators. Thrombus Imaging Characteristics and Outcomes in Acute Ischemic Stroke Patients Undergoing Endovascular Treatment. Stroke 2019;50:2057-64. [Crossref] [PubMed]
- Qazi EM, Sohn SI, Mishra S, Almekhlafi MA, Eesa M, d'Esterre CD, Qazi AA, Puig J, Goyal M, Demchuk AM, Menon BK. Thrombus Characteristics Are Related to Collaterals and Angioarchitecture in Acute Stroke. Can J Neurol Sci 2015;42:381-8. [Crossref] [PubMed]
- Jiang J, Wei J, Zhu Y, Wei L, Wei X, Tian H, Zhang L, Wang T, Cheng Y, Zhao Q, Sun Z, Du H, Huang Y, Liu H, Li Y. Clot-based radiomics model for cardioembolic stroke prediction with CT imaging before recanalization: a multicenter study. Eur Radiol 2023;33:970-80. [Crossref] [PubMed]