Impaired cardiac pump function assessment with normalized cardiac power using cardiac magnetic resonance in patients with hypertrophic cardiomyopathy
Introduction
The heart plays an essential role in blood circulation and meeting the metabolic requirements of the individual. Similar to a power generator, the left ventricle (LV) can eject blood and provide sufficient pressure to overcome vascular resistance and then deliver sufficient volume to perfuse the organs throughout the body (1). Cardiac power (CP) output, which reflects the cardiac pump ability, is calculated using cardiac output (CO) and mean systemic blood pressure (BP) (2). It integrates all of these parameters to assess cardiac performance as a single entity and calculates the energy release of the LV myocardium (3). The general resting CP is about 1 watt (W), assuming a normal arterial pressure of 120/80 mmHg and a CO of 5 L/min (2). Since this power mainly depends on the quantity of muscle producing that power, normalizing it to ventricular mass would be optimal for the evaluation of myocardial performance. Normalized cardiac power (NCP) represents the ability to generate energy with 100 g of myocardium and facilitates comparisons between individuals (4,5).
Hypertrophic cardiomyopathy (HCM) has been identified as the most common hereditary cardiomyopathy, with an incidence of about 1/500 in the general population (6). Pathological studies argue that the inefficient shortening and impaired systolic and diastolic function of HCM is caused by an abnormally thickened myocardium and chaotic myocardial fiber (7). The impaired heart muscle cannot contract efficiently, leading to regional and global dysfunction, which is fairly difficult to detect (8). Owing to the varied cardiac remodeling, patients with HCM usually have a normal or reduced left ventricular cavity along with a preserved or high ejection fraction (EF) (9). These patients often neglect appropriate treatment due to the satisfactory EF value. However, previous studies have reported that some patients with HCM with preserved EF have adverse outcomes, such as cardiac insufficiency, the requirement of implantable defibrillator surgery, and sudden cardiac death (10,11). Therefore, identifying impaired cardiac function in patients with HCM with preserved EF is a key clinical need.
Although echocardiography is widely used to assess cardiac structure and function, cardiac magnetic resonance (CMR) has been recommended as the gold standard in the assessment of HCM due to its high spatial resolution, good image quality, and ability to identify the morphological variants of HCM (12). CMR is also a reference technique for measuring LV volume, EF, and LV mass (13). Therefore, the results of CP and NCP calculated by CMR are accurate and reliable.
Although NCP has been well-studied and confirmed to be an indicator providing valuable prognostic information in patients with heart failure (HF) and preserved or decreased EF (4,5,14), its role in assessing cardiac pump function in patients with HCM remains unclear. Therefore, the present study aimed to evaluate the role of NCP in assessing the cardiac pump function of patients with HCM with preserved EF. We present this article in accordance with the STROBE reporting checklist (available at https://qims.amegroups.com/article/view/10.21037/qims-22-1119/rc).
Methods
Study population
We performed this retrospective cross-sectional study in March 2022 and screened 129 patients with HCM who underwent CMR from December 2020 to January 2022 at Beijing Anzhen Hospital. The following diagnostic criteria of HCM were used according to the recommendations of the European Society of Cardiology (ESC) guidelines (15): myocardial wall thickness ≥15 mm anywhere in the LV or >13 mm and a family history of HCM as measured by CMR at end-diastole. Cases of secondary ventricle hypertrophy due to hypertension (n=2), aortic disease (n=3), or amyloidosis (n=1) were excluded prudently by combining the clinical history with image information. Patients with HCM with decreased EF (<50%) at rest (n=9), serious rheumatic valve disease (n=4), or myocardial surgery (n=11) were also excluded. Ultimately, 99 patients with HCM (median age 50 years) were enrolled in this study (Figure 1).
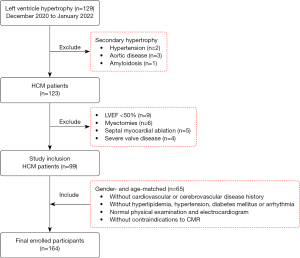
Patients with HCM were diagnosed with HF according to the following criteria (16): (I) symptoms and signs of HF; (II) females with LV mass index ≥95 g/m2 and males with LV mass index ≥115 g/m2; (III) left atrial volume index >34 mL/m2 (>40 mL/m2 for atrial fibrillation); (IV) N-terminal pro-B-type natriuretic peptide (NT-proBNP) >125 pg/mL (>365 pg/mL for atrial fibrillation). Meanwhile, 65 gender- and age-matched healthy volunteers (median age 42 years) were enrolled as the control group and were screened according the following criteria: (I) without cardiovascular or cerebrovascular disease history; (II) without a diagnosis of hyperlipidemia, hypertension, diabetes mellitus, or arrhythmia; (III) normal physical examination and electrocardiogram; (IV) without contraindications to CMR. We calculated the sample size using the mean ± standard error of the CP value, and the statistical power and alpha value were set as 0.9 and 0.05, respectively. The number of healthy volunteers was defined as 0.89±0.33 W according to the published research (17), while that of patients with HCM was calculated from a subgroup of 30 randomly selected participants. The minimum sample size was 57 for both patients with HCM and volunteers. In total, the study cohort consisted of 164 participants with a median age of 47 years (range, 12–73 years), and patients with HCM exhibited similar clinical features to those of the controls (Table 1).
Table 1
Parameter | HCM participants (N=99) | Control participants (N=65) | P value |
---|---|---|---|
Age (years) | 50 (37 to 59) | 42 (33 to 57) | 0.06 |
Male | 65 [66] | 43 [66] | 0.94 |
BSA (m2) | 1.81±0.23 | 1.84±0.21 | 0.44 |
BMI (kg/m2) | 25.83±4.01 | 25.25±3.72 | 0.35 |
Systolic BP (mmHg) | 128±17 | 125±11 | 0.18 |
Diastolic BP (mmHg) | 77±10 | 77±10 | 0.85 |
Mean BP (mmHg) | 94±11 | 93±9 | 0.63 |
Heart rate (beats/min) | 75±14 | 75±15 | 0.94 |
Smoking | 37 [37] | 15 [23] | 0.05 |
Drinking | 6 [6] | 8 [12] | 0.16 |
Diabetes | 24 [24] | – | – |
Hyperlipidemia | 31 [31] | – | – |
Hypertension | 46 [47] | – | – |
Arrhythmia | 29 [29] | – | – |
CAD | 18 [18] | – | – |
Syncope | 8 [8] | – | – |
Normal distribution values are expressed as the mean ± standard error; abnormal distribution values are presented as the median (quartile 1 to quartile 3); categorical variables are displayed as the number [%]. HCM, hypertrophic cardiomyopathy; BSA, body surface area; BMI, body mass index; BP, blood pressure; CAD, coronary artery disease.
The research protocol of this study complied with the Declaration of Helsinki (as revised in 2013) and was approved by the Human Subjects Review Committee at Beijing Anzhen Hospital (No. 2013007X). All participants signed informed consent permitting the use of their data for research purposes.
Arterial blood pressure measurement
The study design is illustrated in Figure 2. A standardized measurement method was applied to acquire arterial BP 20–30 min routinely before CMR scanning. The participants were asked to sit and stay calm for 5–10 min before the measurements were taken from the right brachial artery, and the average value of 3 measurements was recorded.
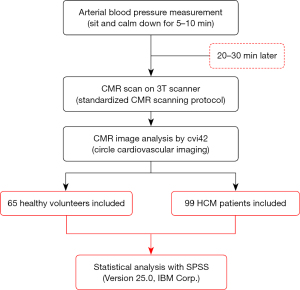
CMR scanning protocol
All participants underwent a standardized CMR scan with breath holding on a 3T CMR scanner (Ingenia CX, Philips Healthcare, Amsterdam, The Netherlands). the steady-state free procession (SSFP) sequence was used to generate 4-chamber, 2-chamber, and short-axis cine imaging series. The other parameters were set as follows: time of repetition (TR)/time of echo (TE) = 3.0/1.52 ms, flip angle (FA) = 45°, voxel size = 1.8×1.8×8 mm3, and field of view (FOV) = 270×270 mm2. A total of 10–12 slices were set in short-axis cine images, ranging from 10 mm above the mitral valve level to the apex of the heart, with a slice thickness of 8 mm and a 2 mm gap between the slices. A contrast agent (0.2 mmoL/kg of Magnevist gadopentetate dimeglumine; Bayer, Berlin, Germany) was administered intravenously. After 10 min, late gadolinium enhancement (LGE) was performed using the phase-sensitive inversion-recovery (PSIR) turbo field echo (TFE) sequence under the following parameters: TR/TE = 6.1/3.0 ms, voxel size = 1.6×1.9×8 mm3, FOV = 350×350 mm2, FA = 25°/5°, and acceleration factor = 2.
CMR image analysis for LV function
Commercial software cvi42 (Circle Cardiovascular Imaging, Calgary, AB, Canada) was used to analyze the CMR images. A 16-segment method proposed by the American Heart Association (AHA), the bull’s-eye plot, was adopted. The basal and middle ventricle in the short-axis cine images were divided into 6 regions, and the apical part was divided into 4 regions. A series comprising the 2-chamber view on the vertical long axis, 4-chamber view on the long horizontal axis, and short-axis (performed based on four-chamber images in the ventricular long-axis plane) slices were loaded into the cardiac function analysis module. Both the endocardial and epicardial contours on the end-systolic and end-diastolic phases of the LV were drawn semiautomatically and modified manually with the papillary muscles and intertrabecular blood pools being excluded (Figure 3).
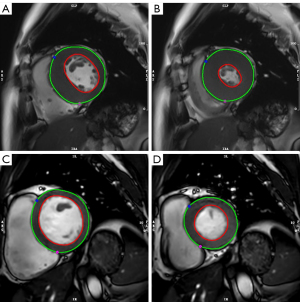
The LV cardiac functional parameters, including end-diastolic volume (EDV), end-systolic volume (ESV), CO, EF, and LV mass were estimated semiautomatically using the short-3D module. The LV maximal wall thickness (LVMWT) of the 16 segments was acquired by measuring the maximal wall thickness at the end-diastole directly. The quantitative measurement of LGE extent (%) was performed on the apex, mid-ventricular, and basal levels using the LGE images, and 5 standard deviations were set as the threshold compared to the normal area. The maximum left atrial volume (LAV) was measured in systole by drawing the endocardial contour of the left atrium in both 2- and 4-chamber images manually. The measurement work was performed by observer A (with 5 years of experience in CMR image interpretation) who was blinded to both the grouping of the participants and the grouping information of the CMR images.
Power-to-mass ratio calculation
The formula of NCP at rest was as follows: power-to-mass ratio (power/mass) = 0.22 × CO × mean BP/LV mass, where 0.222 is the transform constant to W/100 g of the LV myocardium (2). The CO and LV mass were acquired through CMR image analysis.
Statistical analysis
Statistical analysis was performed using SPSS (version 25.0, IBM Corp., Armonk, NY, USA). There were no missing data in the current study, and the chi-squared test, Student t-test, and Wilcoxon rank-sum test were used as appropriate. Multivariable linear regression analysis was performed to examine the correlation between the relevant factors and NCP. An elementary model (model 1) was designed by controlling for baseline parameters [age, male sex, and body mass index (BMI)], several common cardiovascular comorbidities [hypertension, diabetes, hyperlipidemia, arrhythmia, and coronary artery disease (CAD)], and relevant cardiac functional parameters (LV mass index, EDV, and EF). Variables for inclusion were carefully selected to ensure the efficiency of the final model. An optimized model (model 2) was established using the backward procedure (excluding the least significant parameters step by step until the most optimal model was established).
Further, hemodynamic characteristics and differences between patients with HCM with and without HF were explored. In patients with HCM, Spearman correlation coefficient was used to evaluate the correlation of the cardiac functional parameters with CP and NCP. The correlation was considered very strong if R was 0.80–1.00, strong if R was 0.60–0.79, moderate if R was 0.40–0.59, weak if R was 0.20–0.39, and very weak if R was 0–0.19. The exponential function was also used to observe the tendency of CP with increasing LV mass. The cardiac volumes and LV mass were indexed to the body surface area (BSA), and significance was set as a 2-sided P value of <0.05.
In a subgroup of 30 randomly selected participants, the interobserver reproducibility of several key LV functional parameters [LVEF (%), CO (L/min), LV mass (g), and EDV (mL)] was assessed with intraclass correlation coefficients (ICCs). Observer B (with 6 years of experience in CMR image interpretation), who was also blinded to the grouping information, assessed these participants to evaluate the interobserver reproducibility. The reproducibility was considered excellent if the ICC was 0.7–1.0, moderate if the ICC was 0.3–0.7, and poor if the ICC was 0–0.3.
Results
Cardiac function and morphological parameters
Compared to the controls, the patients with HCM had a higher CP (1.19 vs. 1.01 W; P=0.03) but a significantly lower NCP (0.73 vs. 1.12 W/100 g; P<0.001; Table 2 and Figure 4). Patients with HCM also had a higher EF (71.6% vs. 61.5%; P<0.001), greater LV mass (161.6 vs. 97.0 g; P<0.001) and LV mass/BSA (91.1 vs. 52.6 g/m2; P<0.001), and higher LVMWT (21.0 vs. 9.8 mm; P<0.001). About 86% of patients presented with myocardial fibrosis, as assessed by LGE (13.71%±2.40%).
Table 2
Parameter | HCM participants (N=99) | Control participants (N=65) | P value |
---|---|---|---|
CP (W) | 1.19 (0.88–1.57) | 1.01 (0.87–1.29) | 0.03 |
NCP (W/100 g) | 0.73 (0.57–0.90) | 1.12 (0.91–1.36) | <0.001 |
EF (%) | 71.6 (63.3–74.9) | 61.5 (57.1–67.6) | <0.001 |
SV (mL) | 88.7 (71.8–115.7) | 74.4 (63.0–90.4) | 0.002 |
SV/BSA (mL/m2) | 50.1 (40.8–60.2) | 41.6 (35.6–49.4) | <0.001 |
CO (L/min) | 6.01 (4.62–7.43) | 5.17 (4.41–6.19) | 0.01 |
CO/BSA (L/m2/min) | 3.29 (2.68–3.98) | 2.90 (2.45–3.25) | 0.003 |
EDV (mL) | 126.9 (105.8–160.5) | 125.6 (101.7–150.7) | 0.34 |
EDV/BSA (mL/m2) | 72.5 (62.1–84.7) | 68.9 (58.1–78.3) | 0.11 |
ESV (mL) | 40.7 (28.0–53.4) | 45.6 (37.2–57.4) | 0.01 |
ESV/BSA (mL/m2) | 21.7 (16.0–28.4) | 25.6 (20.8–30.3) | 0.01 |
LV mass (g) | 161.6 (126.2–210.5) | 97.0 (73.7–119.0) | <0.001 |
Mass/BSA (g/m2) | 91.1 (75.8–108.7) | 52.6 (43.5–62.7) | <0.001 |
LVMWT (mm) | 21.0 (16.0–28.4) | 9.8 (8.4–10.9) | <0.001 |
LAV (mL) | 68.3 (50.9–88.0) | 45.2 (33.4–57.5) | <0.001 |
LAV/BSA (mL/m2) | 40.5 (29.4–51.3) | 24.7 (18.0–31.1) | <0.001 |
Obstruction | 61 [62] | – | – |
Myocardial fibrosis | 85 [86] | – | – |
LVMWT ≥30 mm | 10 [10] | – | – |
NT-proBNP (pg/mL) | 196.3±37.6 | – | – |
LGE (%) | 13.71±2.40 | – | – |
Normal distribution values are expressed as the mean ± standard error; abnormal distribution values are presented as the median (quartile 1 to quartile 3); categorical variables are displayed as the number [percentage]. HCM, hypertrophic cardiomyopathy; CP, cardiac power; NCP, normalized cardiac power; EF, ejection fraction; SV, stroke volume; BSA, body surface area; CO, cardiac output; EDV, end-diastole volume; ESV, end-systolic volume; LV, left ventricle; LVMWT, left ventricular maximal wall thickness; LAV, left atrium volume; NT-proBNP, N-terminal pro-B-type natriuretic peptide; LGE, late gadolinium enhanced.
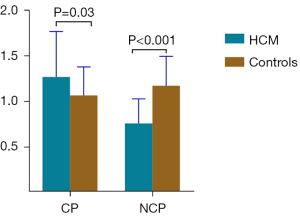
Multivariable analysis
Multivariable linear regression analysis was performed to examine the correlations between the relative factors and NCP (Table 3). The coefficient value of β and 95% confidence interval (CI) were reported. The extent of LGE was excluded because of the collinearity relationship with LV mass/BSA. In the final minimally adjusted model, HCM (β=−0.235; 95% CI: −0.341 to −0.129; P<0.001), LV mass/BSA (β=−0.014; 95% CI: −0.016 to −0.013; P<0.001), EDV (β=0.012; 95% CI: 0.011–0.013; P<0.001), BMI (β=−0.017; 95% CI: −0.026 to −0.007; P<0.001), EF (β=0.010; 95% CI: 0.005–0.014; P<0.001), and hypertension (β=0.149; 95% CI: 0.059–0.240; P=0.001) were significantly associated with NCP (Figure 5).
Table 3
Factor | Model 1 | Model 2 | |||
---|---|---|---|---|---|
β* (95% CI) | P value | β* (95% CI) | P value | ||
HCM | −0.213 (−0.325 to −0.102) | <0.001 | −0.235 (−0.341 to −0.129) | <0.001 | |
LV mass/BSA (g/m2) | −0.016 (−0.018 to −0.015) | <0.001 | −0.014 (−0.016 to −0.013) | <0.001 | |
EDV (mL) | 0.014 (0.013 to 0.015) | <0.001 | 0.012 (0.011 to 0.013) | <0.001 | |
BMI (kg/m2) | −0.014 (−0.024 to −0.004) | 0.006 | −0.017 (−0.026 to −0.007) | <0.001 | |
EF (%) | 0.009 (0.005 to 0.014) | <0.001 | 0.010 (0.005 to 0.014) | <0.001 | |
Hypertension (%) | 0.168 (0.068 to 0.268) | 0.001 | 0.149 (0.059 to 0.240) | 0.001 | |
Arrhythmia (%) | −0.085 (−0.186 to 0.016) | 0.10 | −0.090 (−0.186 to 0.006) | 0.06 | |
Male (%) | 0.057 (−0.024 to 0.138) | 0.16 | |||
Age (years) | −0.001 (−0.003 to 0.002) | 0.86 | |||
Diabetes (%) | −0.037 (−0.154 to 0.081) | 0.53 | |||
Hyperlipidemia (%) | −0.026 (−0.135 to 0.082) | 0.48 | |||
CAD (%) | −0.048 (−0.178 to 0.083) | 0.47 |
β* indicates the changed value of NCP with a 1 unit increase of the determinant. NCP, normalized cardiac power; CI, confidence interval; HCM, hypertrophic cardiomyopathy; LV, left ventricle; BSA, body surface area; EDV, end-diastole volume; BMI, body mass index; EF, ejection fraction; CAD, coronary artery disease.
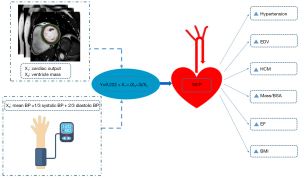
Hemodynamic characteristics in patients with HCM with HF
Compared to the patients without HF, the patients with HCM with HF had higher LV mass (186.2 vs. 157.2 g; P=0.02) and LV mass/BSA (118.6 vs. 86.8 g/m2; P=0.01) (Table 4), significantly lower NCP (0.54 vs. 0.78 W/100 g; P<0.001), and a similar CP (1.14 vs. 1.24 W; P=0.06). The patients with HF and HCM also had a higher mean BP (98±14 vs. 91±9 mmHg; P=0.01) and the LGE extent (16.32%±6.51% vs. 11.97%±3.64%; P=0.001). In addition, 65% of the patients with HF had obstructive HCM.
Table 4
Parameter | HF-patients (N=34) | Non-HF patients (N=65) | P value |
---|---|---|---|
Age (years) | 50 (36 to 60) | 49 (38 to 58) | 0.59 |
Male | 22 [65] | 43 [66] | 0.88 |
BSA (m2) | 1.80±0.31 | 1.82±0.18 | 0.77 |
Systolic BP (mmHg) | 136±20 | 123±13 | 0.002 |
Diastolic BP (mmHg) | 79±12 | 75±9 | 0.07 |
Mean BP (mmHg) | 98±14 | 91±9 | 0.01 |
Hypertension | 21 [62] | 25 [39] | 0.02 |
CP (W) | 1.14 (0.70–1.57) | 1.24 (0.95–1.58) | 0.06 |
NCP (W/100 g) | 0.54 (0.45–0.84) | 0.78 (0.64–0.92) | <0.001 |
EF (%) | 65.4 (58.2–72.0) | 73.5 (66.9–75.9) | <0.001 |
CO (L/min) | 4.95 (3.73–6.78) | 6.39 (5.21–7.63) | 0.004 |
CO/BSA (L/m2/min) | 2.86 (2.34–3.49) | 3.47 (2.87–4.22) | 0.001 |
EDV (mL) | 119.8 (87.5–160.6) | 128.1 (110.4–161.2) | 0.32 |
EDV/BSA (mL/m2) | 69.9 (53.7–86.4) | 72.8 (63.5–83.7) | 0.39 |
LV mass (g) | 186.2 (165.1–224.2) | 157.2 (122.1–187.3) | 0.02 |
Mass/BSA (g/m2) | 118.6 (98.9–135.3) | 86.8 (71.5–99.7) | 0.01 |
LAV (mL) | 87.0 (69.9–103.0) | 53.7 (34.0–70.1) | 0.02 |
LAV/BSA (mL/m2) | 51.5 (40.4–61.3) | 30.3 (20.9–38.4) | 0.008 |
NT-proBNP (pg/mL) | 410.6±59.3 | 89.3±13.6 | <0.001 |
LGE (%) | 16.32±6.51 | 11.97±3.64 | 0.001 |
Obstruction | 22 [65] | 39 [60] | 0.64 |
Normal distribution values are presented as the mean ± standard error; abnormal distribution values are expressed as the median (quartile 1 to quartile 3); categorical variables are displayed as the number [percentage]. HCM, hypertrophic cardiomyopathy; HF, heart failure; BSA, body surface area; BP, blood pressure; CP, cardiac power; NCP, normalized cardiac power; EF, ejection fraction; CO, cardiac output; EDV, end-diastole volume; LV, left ventricle; LAV, left atrium volume; NT-proBNP, N-terminal pro-B-type natriuretic peptide; LGE, late gadolinium enhanced.
Correlation of CP and NCP with CMR parameters in patients with HCM
In patients with HCM, CP was associated with LV mass (R=0.551; 95% CI: 0.391–0.679; P<0.001), LV mass/BSA (R=0.386; 95% CI: 0.198–0.547; P<0.001) (Table 5) and the extent of LGE (R=−0.563; 95% CI: −0.664 to −0.427; P<0.001). On the other hand, NCP was positively correlated with CO (R=0.406, 95% CI: 0.222–0.563; P<0.001) and CO/BSA (R=0.412; 95% CI: 0.228–0.567; P<0.001) (Figure 5), and inversely correlated with the extent of LGE (R=−0.479; 95% CI: −0.632 to −0.291; P<0.001) as well as LV mass (R=−0.395; 95% CI: −0.554 to −0.208; P<0.001) and LV mass/BSA (R=−0.509; 95% CI: −0.646 to −0.341; P<0.001). CP increased with increasing LV mass, while the slope decreased when fit in an exponential function (Figure 6).
Table 5
Parameter | CP (W) | NCP (W/100 g) | |||
---|---|---|---|---|---|
R (95% CI) | P value | R (95% CI) | P value | ||
CO (L/min) | 0.944 (0.916 to 0.963) | <0.001 | 0.406 (0.222 to 0.563) | <0.001 | |
CO/BSA (L/m2/min) | 0.846 (0.777 to 0.895) | <0.001 | 0.412 (0.228 to 0.567) | <0.001 | |
LV mass (g) | 0.551 (0.391 to 0.679) | <0.001 | −0.395 (−0.554 to −0.208) | <0.001 | |
LV mass/BSA (g/m2) | 0.386 (0.198 to 0.547) | <0.001 | −0.509 (−0.646 to −0.341) | <0.001 | |
SV (mL) | 0.789 (0.698 to 0.855) | <0.001 | 0.184 (−0.019 to 0.372) | 0.06 | |
SV/BSA (mL/m2) | 0.648 (0.513 to 0.752) | <0.001 | 0.158 (−0.016,0.349) | 0.11 | |
EDV (mL) | 0.760 (0.658 to 0.834) | <0.001 | 0.238 (0.036 to 0.423) | 0.02 | |
EDV/BSA (mL/m2) | 0.617 (0.473 to 0.729) | <0.001 | 0.049 (−0.155 to 0.249) | 0.63 | |
ESV (mL) | 0.422 (0.239 to 0.576) | <0.001 | −0.139 (−0.333 to 0.065) | 0.17 | |
ESV/BSA (mL/m2) | 0.303 (0.106 to 0.476) | 0.002 | −0.184 (−0.373 to 0.019) | 0.06 | |
EF (%) | 0.124 (−0.080 to 0.319) | 0.22 | 0.334 (0.140 to 0.503) | 0.001 | |
LGE (%) | −0.563 (−0.664 to −0.427) | <0.001 | −0.479 (−0.632 to −0.291) | <0.001 | |
LVMWT (mm) | −0.045 (−0.245 to 0.159) | 0.65 | 0.004 (−0.198 to 0.207) | 0.96 |
CP, cardiac power; NCP, normalized cardiac power; CMR, cardiac magnetic resonance; HCM, hypertrophic cardiomyopathy; CI, confidence interval; CO, cardiac output; BSA, body surface area; LV, left ventricle; SV, stroke volume; EDV, end-diastole volume; ESV, end-systolic volume; EF, ejection fraction; LGE, late gadolinium enhanced; LVMWT, left ventricular maximal wall thickness.
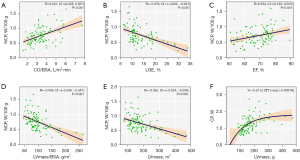
The reproducibility between observers of cardiac functional parameters was excellent for LVEF (ICC =0.922; 95% CI: 0.844–0.962), CO (ICC =0.915; 95% CI: 0.830–0.959), LV mass (ICC =0.927; 95% CI: 0.755–0.971), and EDV (ICC =0.884; 95% CI: 0.769–0.943).
Discussion
To the best of our knowledge, this is the first study to explore the role of NCP in a cohort of patients with HCM with preserved EF. Two key findings improved our understanding of the cardiac pump function in patients with HCM. First, patients with HCM with normal EF had a higher CP but lower NCP than did the controls, which reflects the impaired work efficiency of the myocardium. Second, NCP was negatively correlated with ventricle mass in patients with HCM. These findings confirmed the potential role of NCP in detecting impaired cardiac pump function in patients with HCM with preserved EF.
Since CP depends on the muscle volume and its work efficiency, normalizing it using the ventricular mass has been proven to be a better index of myocardial performance, facilitating comparisons between individuals (5). This is especially true for patients with obviously abnormal ventricle hypertrophy because NCP encompasses the ventricle mass and reflects the true myocardial work efficiency. NCP has been proven to be more predictive than CP in cardiac mortality and HF (5). Therefore, compared to CP and EF, NCP might be a better choice to assess cardiac pump function in patients with HCM.
In the current study, we found that although patients with HCM had a higher CP than did the controls, their NCP was already impaired. Furthermore, CP was still similar between HCM patients with and without HF, while NCP decreased significantly in patients with HF, which also indicated that NCP was more sensitive than CP. The work efficiency, represented by NCP, was impacted by myocardial fibrosis, cardiomyocyte hypertrophy, and disorganized myocardial fiber, which are the most common characteristics in patients with HCM according to previous pathological studies (18-20). In the early stage of HCM, the increase in muscle mass compensates for the decrease in work efficiency, which resulted in a higher CP in patients with HCM compared to controls. In the advanced stage, the increased muscle mass cannot compensate for the impaired work efficiency, which resulted in a higher ventricle mass but similar CP in patients with HCM with HF.
Surprisingly, the NCP was negatively correlated with ventricle mass in patients with HCM. Although hypertrophy is usually considered a positive compensation to a changed load, increased ventricular mass might result in an imbalance in the myocardial oxygen demand and supply because of the relative reduction of capillaries, increased intercapillary distance, and relative reduction in energy-producing mitochondria (21). In patients with HCM, myocyte hypertrophy, disarray, and myocardial fibrosis might exacerbate the decrease in work efficiency. Pathologic hypertrophy is associated with increased collagen deposition in the extracellular matrix and around the intramyocardial coronary arteries (22,23). The resulting interstitial and perivascular fibrosis have been linked to increased LV stiffness and impaired coronary reserve (24), both of which may worsen heart failure and ischemia. A previous study also considered that when compromised contractile function coexists with an increased mass, it likely means that the work efficiency of the myocardium is impaired as a result of the maladaptive feature of LV remodeling (25).
We also analyzed the hemodynamic characteristics of patients with HCM and HF. In the study cohort, about 65% of patients with HCM and HF had obstructive HCM. Patients with HCM and HF had a higher mean BP (especially systolic BP) than did those without, suggesting pressure overload. These findings were consistent with previous research reporting that about 70% of patients with HCM and HF have obstructive HCM and they tend to exhibit pressure overload caused by subaortic obstruction and increased wall stress (26). On the contrary, decreased EF and volume overload was more common in conventional patients with congestive HF, whereas obstruction and pressure overload were relatively infrequent.
In patients with HCM, patchy or regional myocardial fibrosis was reported to be major pathological change and could be assessed by LGE directly and precisely (27). In the current study, 86% of patients with HCM exhibited myocardial fibrosis. A previous study found that the systolic function and deformation of the fibrosis myocardium were impaired in patients with HCM (20). We also found that NCP was negatively correlated with the extent of LGE. Therefore, we speculated that the myocardial fibrosis in our study might explain the impaired NCP in patients with HCM to some extent.
LV global longitudinal strain (GLS) has also been shown to be a sensitive parameter to detect ventricular dysfunction (8,28). The GLS mainly measures the longitudinal shortening of subendocardial myofibers. Meanwhile, in patients with HCM, the circumferential myofibers in the midwall and vertical outer fibers are also impaired, but this cannot be quantified accurately using global circumferential or radial strain due to relatively low repeatability. Similar to EF, another limitation of GLS is the marked afterload dependency (1). Moreover, reduced GLS is detected in only about 50% of patients with HF and preserved EF (29,30), indicating that GLS cannot provide valuable information for other patients. Thus, as a valid assessment of cardiac work efficiency, normalized cardiac power could be an effective supplementary method to cardiac pump function assessment. Moreover, compared to myocardial strain, NCP is easier to acquire for clinicians, as an additional analysis module is required for myocardial strain. However, the potential added prognostic value of NCP compared to myocardial strain needs to be clarified in future studies.
Despite promising findings, some limitations to this study should be noted. First, we did not evaluate the peak NCP during strenuous exercise because it is dangerous for patients with HCM. Peak NCP may be more sensitive in detecting cardiac dysfunction, as it might decline to a point when the NCP at rest starts to decline (31). Second, no long-term follow-up was carried out because HCM is a chronic progressive disease with a low risk of adverse cardiovascular events (rate 0.5%/year) (11), and thus, the predictive values of NCP could not be obtained. Third, we only analyzed the patients with HCM whose EF was ≥50% in the current study, so the role of NCP in patients with HCM with impaired EF needs to be investigated further.
Conclusions
As it is a slowly progressive disease with poor clinical outcomes, there is still no efficient parameter to assess the cardiac work efficiency of HCM. Our study indicated that NCP, as a concise and noninvasive quantitative metric parameter of cardiac function, might play a critical role in detecting and evaluating impaired cardiac pump function in patients with HCM and preserved EF. Future studies analyzing the relationship between NCP and clinical outcomes are warranted.
Acknowledgments
Funding: This work was supported by the National Natural Science Foundation of China (No. U1908211) and the Capital’s Funds for Health Improvement and Research Foundation of China (No. 2020-1-1052).
Footnote
Reporting Checklist: The authors have completed the STROBE reporting checklist. Available at https://qims.amegroups.com/article/view/10.21037/qims-22-1119/rc
Conflicts of Interest: All authors have completed the ICMJE uniform disclosure form (available at https://qims.amegroups.com/article/view/10.21037/qims-22-1119/coif). ZS serves as an unpaid deputy editor of Quantitative Imaging in Medicine and Surgery. The other authors have no conflicts of interest to declare.
Ethical Statement: The authors are accountable for all aspects of the work in ensuring that questions related to the accuracy or integrity of any part of the work are appropriately investigated and resolved. This study was conducted in accordance with the Declaration of Helsinki (as revised in 2013) and was approved by the Human Subjects Review Committee at Beijing Anzhen Hospital (approval No. 2013007X). All participants signed the informed consent to allow the use of their data for research purposes.
Open Access Statement: This is an Open Access article distributed in accordance with the Creative Commons Attribution-NonCommercial-NoDerivs 4.0 International License (CC BY-NC-ND 4.0), which permits the non-commercial replication and distribution of the article with the strict proviso that no changes or edits are made and the original work is properly cited (including links to both the formal publication through the relevant DOI and the license). See: https://creativecommons.org/licenses/by-nc-nd/4.0/.
References
- Smiseth OA, Aalen JM, Skulstad H. Heart failure and systolic function: time to leave diagnostics based on ejection fraction? Eur Heart J 2021;42:786-8. [Crossref] [PubMed]
- Lim HS. Cardiac Power Output Revisited. Circ Heart Fail 2020;13:e007393. [Crossref] [PubMed]
- Harada T, Yamaguchi M, Omote K, Iwano H, Mizuguchi Y, Amanai S, Yoshida K, Kato T, Kurosawa K, Nagai T, Negishi K, Anzai T, Obokata M. Cardiac Power Output Is Independently and Incrementally Associated With Adverse Outcomes in Heart Failure With Preserved Ejection Fraction. Circ Cardiovasc Imaging 2022;15:e013495. [Crossref] [PubMed]
- Anand V, Kane GC, Scott CG, Pislaru SV, Adigun RO, McCully RB, Pellikka PA, Pislaru C. Prognostic value of peak stress cardiac power in patients with normal ejection fraction undergoing exercise stress echocardiography. Eur Heart J 2021;42:776-85. [Crossref] [PubMed]
- Cortigiani L, Sorbo S, Miccoli M, Scali MC, Simioniuc A, Morrone D, Bovenzi F, Marzilli M, Dini FL. Prognostic value of cardiac power output to left ventricular mass in patients with left ventricular dysfunction and dobutamine stress echo negative by wall motion criteria. Eur Heart J Cardiovasc Imaging 2017;18:153-8. [Crossref] [PubMed]
- Semsarian C, Ingles J, Maron MS, Maron BJ. New perspectives on the prevalence of hypertrophic cardiomyopathy. J Am Coll Cardiol 2015;65:1249-54. [Crossref] [PubMed]
- Bluemke DA, Lima JAC. Using MRI to Probe the Heart in Hypertrophic Cardiomyopathy. Radiology 2020;294:287-8. [Crossref] [PubMed]
- Vigneault DM, Yang E, Jensen PJ, Tee MW, Farhad H, Chu L, Noble JA, Day SM, Colan SD, Russell MW, Towbin J, Sherrid MV, Canter CE, Shi L, Ho CY, Bluemke DA. Left Ventricular Strain Is Abnormal in Preclinical and Overt Hypertrophic Cardiomyopathy: Cardiac MR Feature Tracking. Radiology 2019;290:640-8. [Crossref] [PubMed]
- Ozawa K, Funabashi N, Takaoka H, Kamata T, Kanaeda A, Saito M, Nomura F, Kobayashi Y. Characteristic myocardial strain identified in hypertrophic cardiomyopathy subjects with preserved left ventricular ejection fraction using a novel multi-layer transthoracic echocardiography technique. Int J Cardiol 2015;184:237-43. [Crossref] [PubMed]
- Karabinowska-Małocha A, Dziewięcka E, Banyś P, Urbańczyk-Zawadzka M, Krupiński M, Mielnik M, Łach J, Budkiewicz A, Podolec P, Żydzik Ł, Wiśniowska-Śmiałek S, Holcman K, Kostkiewicz M, Rubiś P. The Relationship between Cardiac Magnetic Resonance-Assessed Replacement and Interstitial Fibrosis and Ventricular Arrhythmias in Hypertrophic Cardiomyopathy. J Pers Med 2022; [Crossref] [PubMed]
- Maron BJ, Rowin EJ, Casey SA, Maron MS. How Hypertrophic Cardiomyopathy Became a Contemporary Treatable Genetic Disease With Low Mortality: Shaped by 50 Years of Clinical Research and Practice. JAMA Cardiol 2016;1:98-105. [Crossref] [PubMed]
- Sivalokanathan S. The Role of Cardiovascular Magnetic Resonance Imaging in the Evaluation of Hypertrophic Cardiomyopathy. Diagnostics (Basel) 2022.
- Liu J, Li J, Pu H, He W, Zhou X, Tong N, Peng L. Cardiac remodeling and subclinical left ventricular dysfunction in adults with uncomplicated obesity: a cardiovascular magnetic resonance study. Quant Imaging Med Surg 2022;12:2035-50. [Crossref] [PubMed]
- Chen C, Zhao J, Xue R, Liu X, Zhu W, Ye M. Prognostic significance of resting cardiac power to left ventricular mass and E/e' ratio in heart failure with preserved ejection fraction. Front Cardiovasc Med 2022;9:961837. [Crossref] [PubMed]
- Elliott PM, Anastasakis A, Borger MA, Borggrefe M, Cecchi F, Charron P, et al. 2014 ESC Guidelines on diagnosis and management of hypertrophic cardiomyopathy: the Task Force for the Diagnosis and Management of Hypertrophic Cardiomyopathy of the European Society of Cardiology (ESC). Eur Heart J 2014;35:2733-79. [Crossref] [PubMed]
- McDonagh TA, Metra M, Adamo M, Gardner RS, Baumbach A, Böhm M, et al. 2021 ESC Guidelines for the diagnosis and treatment of acute and chronic heart failure. Eur Heart J 2021;42:3599-726. [Crossref] [PubMed]
- Dini FL, Mele D, Conti U, Ballo P, Citro R, Menichetti F, Marzilli M. Peak power output to left ventricular mass: an index to predict ventricular pumping performance and morbidity in advanced heart failure. J Am Soc Echocardiogr 2010;23:1259-65. [Crossref] [PubMed]
- Reant P, Barbot L, Touche C, Dijos M, Arsac F, Pillois X, Landelle M, Roudaut R, Lafitte S. Evaluation of global left ventricular systolic function using three-dimensional echocardiography speckle-tracking strain parameters. J Am Soc Echocardiogr 2012;25:68-79. [Crossref] [PubMed]
- Cameli M, Mondillo S, Solari M, Righini FM, Andrei V, Contaldi C, De Marco E, Di Mauro M, Esposito R, Gallina S, Montisci R, Rossi A, Galderisi M, Nistri S, Agricola E, Mele D. Echocardiographic assessment of left ventricular systolic function: from ejection fraction to torsion. Heart Fail Rev 2016;21:77-94. [Crossref] [PubMed]
- Xu HY, Chen J, Yang ZG, Li R, Shi K, Zhang Q, Liu X, Xie LJ, Jiang L, Guo YK. Early marker of regional left ventricular deformation in patients with hypertrophic cardiomyopathy evaluated by MRI tissue tracking: The effects of myocardial hypertrophy and fibrosis. J Magn Reson Imaging 2017;46:1368-76. [Crossref] [PubMed]
- Devereux RB, Bang CN, Roman MJ, Palmieri V, Boman K, Gerdts E, Nieminen MS, Papademetriou V, Wachtell K, Hille DA, Dahlöf B. Left Ventricular Wall Stress-Mass-Heart Rate Product and Cardiovascular Events in Treated Hypertensive Patients: LIFE Study. Hypertension 2015;66:945-53. [Crossref] [PubMed]
- Kruszewska J, Cudnoch-Jedrzejewska A, Czarzasta K. Remodeling and Fibrosis of the Cardiac Muscle in the Course of Obesity-Pathogenesis and Involvement of the Extracellular Matrix. Int J Mol Sci 2022; [Crossref] [PubMed]
- Li L, Zhao Q, Kong W. Extracellular matrix remodeling and cardiac fibrosis. Matrix Biol 2018;68-69:490-506. [Crossref] [PubMed]
- Zhuang R, Chen J, Cheng HS, Assa C, Jamaiyar A, Pandey AK, Pérez-Cremades D, Zhang B, Tzani A, Khyrul Wara A, Plutzky J, Barrera V, Bhetariya P, Mitchell RN, Liu Z, Feinberg MW. Perivascular Fibrosis Is Mediated by a KLF10-IL-9 Signaling Axis in CD4+ T Cells. Circ Res 2022;130:1662-81. [Crossref] [PubMed]
- Russell K, Eriksen M, Aaberge L, Wilhelmsen N, Skulstad H, Gjesdal O, Edvardsen T, Smiseth OA. Assessment of wasted myocardial work: a novel method to quantify energy loss due to uncoordinated left ventricular contractions. Am J Physiol Heart Circ Physiol 2013;305:H996-1003. [Crossref] [PubMed]
- Maron BJ, Rowin EJ, Udelson JE, Maron MS. Clinical Spectrum and Management of Heart Failure in Hypertrophic Cardiomyopathy. JACC Heart Fail 2018;6:353-63. [Crossref] [PubMed]
- Briasoulis A, Mallikethi-Reddy S, Palla M, Alesh I, Afonso L. Myocardial fibrosis on cardiac magnetic resonance and cardiac outcomes in hypertrophic cardiomyopathy: a meta-analysis. Heart 2015;101:1406-11. [Crossref] [PubMed]
- Wang F, Xu X, Wang Q, Yu D, Lv L, Wang Q. Comparison of left ventricular global and segmental strain parameters by cardiovascular magnetic resonance tissue tracking in light-chain cardiac amyloidosis and hypertrophic cardiomyopathy. Quant Imaging Med Surg 2023;13:449-61. [Crossref] [PubMed]
- Morris DA, Ma XX, Belyavskiy E, Aravind Kumar R, Kropf M, Kraft R, Frydas A, Osmanoglou E, Marquez E, Donal E, Edelmann F, Tschöpe C, Pieske B, Pieske-Kraigher E. Left ventricular longitudinal systolic function analysed by 2D speckle-tracking echocardiography in heart failure with preserved ejection fraction: a meta-analysis. Open Heart 2017;4:e000630. [Crossref] [PubMed]
- Shah AM, Claggett B, Sweitzer NK, Shah SJ, Anand IS, Liu L, Pitt B, Pfeffer MA, Solomon SD. Prognostic Importance of Impaired Systolic Function in Heart Failure With Preserved Ejection Fraction and the Impact of Spironolactone. Circulation 2015;132:402-14. [Crossref] [PubMed]
- Cotter G, Williams SG, Vered Z, Tan LB. Role of cardiac power in heart failure. Curr Opin Cardiol 2003;18:215-22. [Crossref] [PubMed]