Changes in brain structure and related functional connectivity during menstruation in women with primary dysmenorrhea
Introduction
Primary dysmenorrhea (PDM) is defined as painful menstrual cramps that cannot be accounted for by any pelvic pathology; PDM can radiate to the inner thighs and lower back and can be accompanied by symptoms such as nausea, vomiting, and diarrhea (1). In the past, the pathophysiological studies on PDM mainly focused on prostaglandins, antidiuretic hormones, and uterine blood flow, but none of these studies could fully explain the mechanism of PDM (2,3). With the development of imaging, neuroimaging studies of PDM have gradually been performed. The changes in brain structure, function, and metabolism in patients with PDM have been confirmed, providing another perspective to understanding PDM (4-7). Neuronal cell death or synapse density changes can result in changes in brain structure (8), while altered structure can further lead to abnormal brain function (9). Hence, multimodal approaches combined with structural and functional analyses are indispensable to understanding brain changes in cases of PDM. However, few studies on changes in the structure and structure-related function in women with PDM have been performed, and these existing studies have focused on regions of interest, such as the amygdala and insula, rather than on the whole brain (6,7). Thus, our study aimed to explore the structural changes based on the whole brain and associated FC changes during menstruation in women with PDM.
Voxel-based morphometry (VBM) and surface-based morphometry (SBM) are the 2 most commonly used morphological analysis methods in detecting gray matter (GM) changes based on high-resolution T1-weighted magnetic resonance images. VBM measures brain volume, while SBM detects different aspects of cortical morphology, including cortical thickness, gyrification index, sulcus depth, and fractal dimension. Since these methods reflect different aspects of brain structure, a combination of the 2 will enable a more complete assessment of changes in brain structure than is possible when either method is used alone. Functional connectivity (FC) analysis assesses brain activity by calculating the correlation between the temporal patterns of spontaneous neural activity in remote brain regions based on resting-state functional magnetic resonance imaging (rs-fMRI) data (10). FC analysis makes it possible to detect functional variation along with structural changes.
Principally, the present study aimed to (I) identify alterations of brain structure in women with PDM during menstruation, (II) investigate whether there are structure-related functional changes, and (III) determine whether these structural and functional changes are related to the clinical measures of PDM. We hypothesized that women with PDM have both structural and structure-related functional changes compared to healthy individuals. We present the following article in accordance with the STROBE reporting checklist (available at https://qims.amegroups.com/article/view/10.21037/qims-22-683/rc).
Methods
Participants
This was a prospective cross-sectional study, for which 31 right-handed patients with PDM and 31 age-matched right-handed healthy controls (HCs) were recruited from December 2016 to January 2018. They were all recruited from institutions of higher learning in Beijing. All participants were fully informed of the project plan and signed an informed consent form. Patients with PDM were included if they (I) met the diagnostic criteria for PDM issued by the Canadian Society of Obstetrics and Gynecology in 2005 (11); (II) were childless women aged 16 to 30 years, considering that the peak age of patients with PDM is 24 years (12); (III) had regular menstrual cycles of 28±7 days; (IV) had a course of disease that was more than 6 months and did not take any medicine or other treatment for PDM in the previous 3 months; and (V) reported a visual analogue scale for pain (VAS-P) score of ≥40 mm for their last 3 menstrual cycles. Inclusion criteria for HCs were the same as those for the PDM group except the menstrual pain. Patients and HCs were excluded if (I) their pelvic ultrasound or menstrual questionnaire suggested secondary dysmenorrhea caused by factors such as endometriosis, adenomyosis, or pelvic inflammation; (II) they had visceral pain and other pain-related diseases; (III) they had psychopathology; (IV) they had used oral contraceptives, analgesics, or antidepressants in the last 6 months; (V) they were preparing for pregnancy or had a positive pregnancy test result; or (VI) they had any magnetic resonance imaging (MRI) contraindications or intracranial organic lesions. Inclusion into the study was jointly decided by 2 attending physicians with more than 3 years of experience, and only those who were confirmed by both for enrollment could be included in the study.
The study was conducted in accordance with the Declaration of Helsinki (as revised in 2013). The study was approved by the Medical and Experimental Animal Ethics Committee of Beijing University of Chinese Medicine (ethics No. 2015BZHYLL0112), and informed consent was obtained from all the participants.
Questionnaires
The visual analogue scale (VAS) (13) was used to evaluate the pain (VAS-P) and anxiety accompanied with pain (VAS-A). The Cox Menstrual Symptom Scale (CMSS) was used to evaluate duration (COX1) and severity (COX2) of dysmenorrhea symptoms, including cramps, nausea, vomiting, loss of appetite, headaches, backaches, leg aches, dizziness, weakness, diarrhea, facial blemishes, abdominal pain, flushing, sleeplessness, general aching, depression, irritability, and nervousness (14). The questionnaires were completed half an hour to 1 hour before the MRI examination in the Department of Radiology, Beijing Hospital of Traditional Chinese Medicine, Capital Medical University.
MRI data acquisition
All participants underwent an MRI examination on the one of the first to third day of their menstrual cycle (menstrual phase) using a Siemens Skyra 3-Tesla scanner in the Department of Radiology, Beijing Hospital of Traditional Chinese Medicine, Capital Medical University. The coil was a head-and-neck-combined 20-channel coil. During the scan, the participants closed their eyes, stayed awake, and avoided systematic thinking. Earplugs and restraining foam pads were used to reduce noise and head movement. After the scan, the patients were asked if they were asleep, and only those who remained awake were included in the study.
T2-weighted images were first performed to exclude intracranial lesions, and then high-resolution T1-weighted images and functional images (echo-planar imaging sequence, EPI; 250 measurements) were collected. The parameters of the MRI scan are shown in Table 1.
Table 1
Parameters | T1 | EPI | T2 |
---|---|---|---|
TE (ms) | 2.32 | 30.00 | 99 |
TR (ms) | 2,300 | 3,000 | 4,000 |
FA (°) | 8 | 90 | 150 |
MZ | 256×256 | 94×94 | 384×384 |
FOV (mm²) | 240×240 | 220×220 | 220×220 |
VZ (mm³) | 0.9×0.9×0.9 | 2.3×2.3×3.0 | 0.6×0.6×5 |
Slices | 192 | 40 | 20 |
EPI, echo-planar imaging; TE, echo time; TR, repetition time; FA, flip angle; MZ, matrix size; FOV, field of view; VZ, voxel size.
VBM and SBM analysis of T1-weighted images
VBM and SBM for structure analysis were performed with the Computational Anatomy Toolbox (CAT12; http://dbm.neuro.unijena.de/cat12/) within Statistical Parametric Mapping software (SPM12; http://www.fil.ion.ucl.ac.uk/spm/software/spm12/; accessed on October 9, 2021). The CAT12 pipeline with default parameters extracted 1 VBM metric, which was gray matter volume (GMV), and 4 SBM metrics, which were cortical thickness, gyrification index, sulcus depth, and fractal dimension.
First, we checked the T1 data quality and reoriented the origin to the anterior commissure. For the whole brain VBM preprocessing, the data were normalized to the Montreal Neurological Institute (MNI) space using the DARTEL (Difeomorphic Anatomical Registration Through Exponentiated Lie Algebra) method and were segmented into GM, white matter (WM), and cerebrospinal fluid (CSF) according to the tissue probability map (TPM). The segmented GM images were modulated and then smoothed with an 8-mm full width at half maximum (FWHM) Gaussian kernel. For the whole brain SBM preprocessing, the default procedure included segmentation, with the cortical thickness and central surface estimation method including projection-based thickness (PBT) measurement (15), topological correction, spherical mapping, and registration (16,17). Then, cortical thickness and the other 3 SBM metrics were resampled and smoothed with a 15- and 25-mm surface-based kernel, respectively. Hemispheres were not merged prior to the second level of analysis.
FC analysis of resting state functional images
Data preprocessing for FC analysis was conducted on MATLAB platform 2021 (MathWorks) using Data Processing Assistant for Resting-State fMRI (DPARSF) v.4.4 with SPM12 (18). The preprocessing included the following standardized processing steps: first, the first 10 time points were removed; second, slice-timing and realignment were carried out for the remaining 240 time points with the exclusion criteria of 3 mm and 3 degrees in any direction with the middle slice used as a reference, and all the 62 data were eligible; third, data were normalized to MNI space using the DARTEL methods and resampled to 3-mm voxels; fourth, Friston 24 head motion parameters, cerebral spinal fluid signal, WM signal, and global signal were removed as nuisance covariates; fifth, linear drift was removed; sixth, spatial smoothing was completed with a Gaussian kernel of 6×6×6 mm3 FWHM; and finally, the data were filtered with a 0.01–0.08 Hz temporal band-pass.
FC analysis was performed using the method of seed-to-voxel FC analysis. We specified 4 spherical clusters as a seed with a radius of 6 mm centered on the peak coordinates of the right superior temporal gyrus (STG), bilateral orbitofrontal cortex (OFC), left postcentral gyrus (PoCG), and left superior occipital gyrus (SOG) showing group differences in VBM and SBM analysis. For each participant, Pearson correlation analysis was performed between the mean time series of the seed and the time series of every voxel of the whole brain. Fisher’s r-to-z transformation was used to improve the normality of the correlation coefficients.
Statistical analysis
Demographics and clinical symptoms
SPSS 22.0 (IBM Corp.) was used to analyze the demographic and clinical data, including age, menstruation days, menstrual cycle, history of PDM, onset age of PDM, VAS-P, VAS-A, COX1, and COX2. All the data are expressed as mean ± standard deviation (normal distribution), except the menstruation days, which are described as minimum and maximum. A two-sample t-test was used for comparison between groups, and a P value less than 0.05 was considered statistically significant.
Brain imaging
As for the brain imaging data, a two-tailed two-sample t-test (cluster-level family-wise error corrected; voxel P<0.001; cluster P<0.05) was performed to identify the structural and functional differences between the 2 groups.
Correlation between neuroimaging alterations and clinical measures in women with PDM
Correlation analysis was applied using SPSS with the threshold set at a significance level of P<0.05 to detect the underlying relationship between neuroimaging data showing group differences and clinical measures, including duration, onset age of PDM, VAS-A, VAS-P, COX1, and COX2 in patients with PDM by calculating the Pearson correlation coefficient.
Results
Demographics and clinical symptoms
The demographics and clinical data of the 2 groups are shown in Table 2. There were no significant differences in age, menstrual cycle, or menstruation days between the 2 groups (P>0.05).
Table 2
Characteristics | Patients with PDM (n=31) | Healthy controls (n=31) | P value (t-test) |
---|---|---|---|
Age (years) | 24.39±2.486 | 23.97±2.168 | 0.482 |
Menstrual cycle (days) | 29.84±2.830 | 29.00±1.461 | 0.148 |
Menstrual days (days) | 4–7 | 3–8 | 0.115 |
Onset age of PDM (years) | 16.35±2.973 | – | – |
History of PDM (years) | 8.03±3.381 | – | – |
VAS-P | 64.55±16.643 | – | – |
VAS-A | 55.97±21.696 | – | – |
COX1 | 19.13±9.594 | – | – |
COX2 | 16.84±8.430 | – | – |
Values are mean ± standard deviation; the menstrual days are described as a minimum and maximum. PDM, primary dysmenorrhea; VAS-P, visual analogue scale for pain; VAS-A, visual analogue scale for anxiety; COX1, the Cox menstrual symptom scale for duration score of dysmenorrhea symptoms; COX2, the Cox menstrual symptom scale for severity score of dysmenorrhea symptoms.
Brain structural changes
The group comparison analysis of the brain structure between the PDM and HC groups showed a significantly reduced GMV in the right STG and decreased cortical thickness mainly in the bilateral OFC, postcentral PoCG, and left SOG. There were no group differences in the gyrification index, sulcus depth, or fractal dimension. Results are shown in Figure 1 and Table 3.
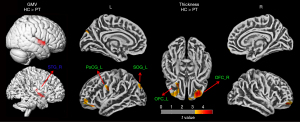
Table 3
Morphology index | Brain region | MNI coordinates | Size (voxel/vertex) | Maximum t value | ||
---|---|---|---|---|---|---|
x | y | z | ||||
GMV | HC > PT | |||||
Right superior temporal gyrus (STG_R) | 54 | 6 | –3 | 1,379 | 5.20 | |
Thickness | HC > PT | |||||
Right orbitofrontal cortex (OFC_R) | 26 | 43 | 5 | 970 | 4.47 | |
Left orbitofrontal cortex (OFC_L) | –24 | 29 | 8 | 1,059 | 4.20 | |
Left postcentral gyrus (PoCG_L) | –56 | –9 | 39 | 1,044 | 4.29 | |
Left superior occipital gyrus (SOG_L) | –12 | –77 | 49 | 542 | 3.83 |
PDM, primary dysmenorrhea; FWE, family-wise error; PT, primary dysmenorrhea patient; HC, healthy control; GMV, gray matter volume; MNI, Montreal Neurological Institute.
FC alterations related to the structural changes
As shown in Figure 2 and Table 4, seed-based FC analysis showed that women with PDM had decreased FCs between the right STG and the left cerebellar posterior lobe (poCb), the right dorsolateral prefrontal cortex (DLPFC), and the left precentral gyrus (PrCG). Women with PMD also had decreased FCs between the left PoCG and the right precuneus and the right DLPFC. Women with PMD had greater FCs between the left PoCG and the bilateral poCb, the left middle temporal gyrus (MTG), and the left angular gyrus.
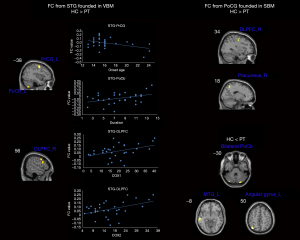
Table 4
Brain region | MNI coordinates | Size (voxel) | Maximum t value | ||
---|---|---|---|---|---|
x | y | z | |||
Seed: STG_R | |||||
HC > PT | |||||
Left cerebellar posterior lobe | –39 | –63 | –54 | 51 | 4.5917 |
Right dorsolateral prefrontal cortex | 57 | 6 | 42 | 64 | 4.9202 |
Left precentral gyrus | –39 | –3 | 54 | 46 | 5.9071 |
Seed: PoCG_L | |||||
HC > PT | |||||
Right precuneus | 21 | –60 | 51 | 44 | 4.5001 |
Right dorsolateral prefrontal cortex | 30 | –12 | 45 | 44 | 4.5226 |
HC < PT | |||||
Left cerebellar posterior lobe | –24 | –84 | –51 | 50 | 4.1695 |
Right cerebellar posterior lobe | 27 | –84 | –48 | 48 | 4.2173 |
Left middle temporal gyrus | –57 | –21 | –12 | 44 | 4.6641 |
Left angular gyrus | –39 | –69 | 51 | 78 | 4.6872 |
PT, primary dysmenorrhea patients; PDM, primary dysmenorrhea; HC, healthy control; FWE, family-wise error; MNI, Montreal Neurological Institute; STG_R, right superior temporal gyrus; PoCG_L, left postcentral gyrus.
Correlation between neuroimage alterations and clinical measures in women with PDM
In women with PDM, the FCs between STG and poCb were positively correlated with history (r=0.388; P=0.031), and FCs between STG and DLPFC were positively correlated with COX1 (r=0.407; P=0.023) and COX2 (r=0.437; P=0.014), while the FCs between STG and PrCG were negatively correlated with the onset age of PDM (r=–0.413; P=0.021). The results are shown in Figure 2.
Discussion
To our knowledge, this study is the first to explore the abnormalities of brain structure and related functional changes in women with PDM. We found morphological changes in STG, OFC, PoCG, and SOG that were mainly involved in the emotional and sensory aspects of pain. The related functional changes were observed mainly in the senior cortex (DLPFC, precuneus), cognitive cerebellum (poCb), and sensorimotor cortex (PrCG and PoCG).
Reduced GMV in STG and related changes in FC
We found reduced GMV in the right STG, mainly in the temporal pole region. The temporal pole is involved in pain processing by regulating the emotional response to pain and is a multisensory integration region responsible for processing pain as well as visual and auditory stimuli (19,20). Additionally, the superior temporal region is generally considered the core region involved in emotional prosody processing and participates in social cognition on account of interaction with the prefrontal cortex (21). Pain is a complex experience, often accompanied by negative emotional states such as anxiety and fear, which can enhance the perception of pain, while women with high emotional regulation ability tend to be less disturbed by pain (22). In addition, structural and functional changes in the temporal cortex have been demonstrated in brain morphology studies on different pain disorders (23-25). In particular, GM features within the PoCG and STG during the periovulatory phase exhibit good performance in discriminating patients with PDM from HCs (23). Therefore, although we did not find a relationship between GMV in STG and VAS-A score, considering the deficiency of VAS-A in assessing pain-related negative emotions, it is still possible that reduced GMV in STG may be a consequence of prolonged negative emotional experiences induced by menstrual pain. Moreover, the disruption of emotional regulation circuits may also mediate the abnormal perception of pain due to the decreased FCs between STG and the left poCb, right DLPFC, and left PrCG found in our study.
The cerebellum not only plays an important role in motor processes but also in cognition (26,27). Abnormalities in the structure and function of the cerebellum have been found in various pain-related diseases, and the cerebellum seems to be involved in every process of pain (28). The poCb is part of the cortical-cerebellar network, which influences the cortical processing of affective-motivational components of pain (29), especially CrusI and CrusII, which are related to aversive stimuli in the limbic system and pain-related fear (30,31). Children with posterior cerebellar tumor resection have been shown to have significantly lower pain tolerances but no difference in pain thresholds (29). Given that pain tolerance is influenced by affective, motivational, and cognitive factors, as well as the emotional function of the STG, the reduction in the STG and poCb FC may be a result of long-term pain-related affective factors. The positive correlation with the history of PDM may reflect cumulative emotional, motivational, or cognitive outcomes over time.
We also found FC reduction between the STG and DLPFC, which was positively correlated with the duration and severity of menstrual pain-related symptoms. The DLPFC actively controls pain perception by regulating subcortical and cortical pathway (32), and plays a role of the inhibition of pain in processing (33). Tu et al. (5) reported decreased regional metabolism in the DLPFC and STG at the onset of cramping menstrual pain. Studies have also indicated that the decreased FC between the DLPFC and periaqueductal gray (PAG) during menstruation reflects the brain response disorder (34). The PAG is known as the critical hub of the descending pain modulatory systems. Furthermore, cognitive-behavioral therapy (CBT) that aims to reduce the negative effect of chronic pain has been used to demonstrate the top-down control of the brain over pain (35). Additionally, the DLPFC can be activated after effective treatment (36). This is consistent with our results, which showed that the FC between the DLPFC and STG increased with the aggravation of pain-related symptoms and was more likely a positive response to reduce negative effects. The decreased FC between the 2 regions could be a disrupted change, representing abnormality in the emotional aspect of pain. The prCG is the motor area. Decreased FC between the prCG and the amygdala has been reported during menstruation (7). Processes involving motor systems may also contribute to inhibitory control (37). Interestingly, our study found that the younger the onset age of PDM was, the more disconnection between the 2 regions. This may suggest a developmental possibility, but caution and more research are required to explain this finding.
Reduced thickness in the left poCG and related changes in FC
The poCG includes the primary somatosensory (S1) cortex, which is a critical component of the nociceptive pathway and is known to receive and process afference to encode sensory identification of nociceptive stimuli, including body location and intensity (38). Some authors have suggested that excessive excitatory input brought by menstrual pain might induce compensatory inhibition of multiple somatosensory motor areas, including the poCG and preCG(5). Furthermore, GMV of several brain regions, including the poCG and preCG, have shown higher accuracy in identifying women with PDM (23). As a region of the pain pathway, finding the functional changes along with the poCG’s structural changes might imply that pain conduction and sensation are changed in PDM. In this study, the increased FC of the bilateral poCb, STG, and angular gyrus—which belong to the default mode network (DMN) and are involved in pain-related emotion and cognition (39)—may serve as a neuroplastic mechanism that compensates for limiting the influence of structural damage, although this idea remains controversial (40). It is worth mentioning that we found decreased FC between the poCG and precuneus. The precuneus is the core region of DMN. DMN abnormalities in PDM and other pain-related diseases have been confirmed in previous studies (4,41,42). Therefore, the changes in FC between the poCG and precuneus found in our study may also be evidence of DMN destruction in PDM. Furthermore, the precuneus is associated with the cognition of pain sensitivity, and the activity of the left precuneus has been negatively correlated with pain sensitivity (43). The precuneus is functionally connected with the supplementary motor area, posterior cingulate cortex, and other central areas (44). Through these areas, it continuously collects information, constructs representations of self and external environment (45), assesses self-related feelings to visceral stimuli (46), and may be involved in internal awareness and adaptation to environmental changes. Studies have shown that the higher the cerebral blood flow of the precuneus in patients with PDM at the baseline is, the worse the response to acupuncture treatment (47). The increased activity may indicate individual difficulty in diverting attention from pain. The observed decreased FC between the precuneus and the S1 cortex may indicate that the information exchange between the precuneus and the S1 cortex is disrupted, leading to the deviation of pain sensitivity in patients with PDM. Consistent with our results, reductions of FCs between the precuneus and several regions involved in pain processing, reward, and higher executive functions were also found in patients with chronic pelvic pain (48).
Reduced thickness in the bilateral OFC and the left SOG
The OFC is involved in sensory integration in representing the affective value of reinforcers and is of vital importance for the adaptive regulation of emotional states and pain (46). Damage to the OFC can impair emotion-related learning, emotional behavior, and subjective emotional states (49,50). In addition to the DLPFC, the OFC may also be the key brain region involved in the intervention of pain during CBT (36). Wu et al. (51) reported activation of the OFC and increased regional homogeneity (ReHo) during menstruation compared with ovulation in patients with PDM, suggesting an adaptation to menstrual pain and related emotions. Another study (52) found an increase in OFC cortical thickness during nonmenstrual periods and a positive correlation with the course of PDM. In their study, the cortical thickness of STG also increased, which was inconsistent with our results. This might be due to the difference in the history of PDM (8.03±3.381) in our samples, which was generally longer than that found in their study (7.0±3.6). The differences in these results suggest that increased OFC and STG cortical thickness or GMV may be a compensation in the short-term over input, while long-term pain as an excessive injurious input may lead to excitotoxicity-related neuronal loss and gray matter structural damage, thereby causing GMV or thickness decline in these 2 brain regions. Meanwhile, both brain regions were involved in emotions and showed a consistent morphological change trend, suggesting that long-term dysmenorrhea might cause abnormal sensory integration and emotional processing in PDM.
The occipital lobe region is well-known as the visual region. However, researchers have found reduced GMV in the occipital lobe in migraine patients without aura (53). Moreover, neuroimaging studies have indicated that visual perception is related to differences in DLPFC activation (54). Furthermore, sensory information originating in the occipital lobe becomes consciousness when it is represented in the DLPFC (55). These findings suggest that there may be an integration of higher regions of the visual system and pain structure in pain processing, which needs to be further explored.
We did not find changes in brain regions, such as the anterior cingulate cortex, thalamus, or insula, which seem to be consistent with those of acute pain (56,57). A significant portion of the brain regions identified in our study were associated with the emotional and sensory aspects of pain. PDM is a type of chronic pain. Chronic pain is often associated with abnormal psychology, and the cognitive and emotional states are powerful regulators of the pain experience (58-60). Our research examined the structural changes in the brain. As mentioned above, structural changes are most commonly caused by neuronal cell death or changes in synaptic density, and these situations do not arise in the short-term. Therefore, the results of our study tend to reflect the emotional consequences of prolonged menstrual pain and help to understand, from a neuroimaging perspective, that the later stages of chronic pain are often accompanied by psychological abnormalities.
Although we found some structural and functional brain changes in patients with PDM during menstruation, several limitations should be noted. First, our study only involved patients from universities, and the conclusions may not be universal or generalizable to all patients with PDM. In the next step, we will expand the sample size to include different populations and age groups involved in PDM. Second, different scales may result in different research results. Other scales, such as the Hamilton Depression Rating Scale (HAM-D) and Hamilton Anxious Rating Scale (HAM-A) or the Beck Depression Inventory (BDI) and Beck Anxiety Inventory (BAI) should be used to assess the presence and severity of depressive and anxious symptomatology for further verification. In addition, considering the interpretations of maladaptive or adaptive reorganizations in response to the long-term pain of PDM identified in this paper, we still need to undertake a longitudinal study to confirm this mechanism.
Conclusions
Our research was in line with the previous studies and revealed that structural and functional brain alterations occurred in women with PDM. These alterations occurred in brain regions mainly associated with the emotional and sensory aspects of pain. The findings of our study can provide insight into the occurrence of PDM from a neuroimaging perspective
Acknowledgments
Funding: This study was supported by the National Natural Science Found of China (No. 81503652) and the University-Level Longitudinal Research Development Foundation of Beijing University of Chinese Medicine (No. 2020-ZXFZJJ-035).
Footnote
Reporting Checklist: The authors have completed the STROBE reporting checklist. Available at https://qims.amegroups.com/article/view/10.21037/qims-22-683/rc
Conflicts of Interest: All authors have completed the ICMJE uniform disclosure form (available at https://qims.amegroups.com/article/view/10.21037/qims-22-683/coif). The authors have no conflicts of interest to declare.
Ethical Statement: The authors are accountable for all aspects of the work in ensuring that questions related to the accuracy or integrity of any part of the work are appropriately investigated and resolved. The study was conducted in accordance with the Declaration of Helsinki (as revised in 2013). The study was approved by the Medical and Experimental Animal Ethics Committee of Beijing University of Chinese Medicine (No. 2015BZHYLL0112), and informed consent was obtained from all the patients.
Open Access Statement: This is an Open Access article distributed in accordance with the Creative Commons Attribution-NonCommercial-NoDerivs 4.0 International License (CC BY-NC-ND 4.0), which permits the non-commercial replication and distribution of the article with the strict proviso that no changes or edits are made and the original work is properly cited (including links to both the formal publication through the relevant DOI and the license). See: https://creativecommons.org/licenses/by-nc-nd/4.0/.
References
- Kho KA, Shields JK. Diagnosis and Management of Primary Dysmenorrhea. JAMA 2020;323:268-9. [Crossref] [PubMed]
- Barcikowska Z, Rajkowska-Labon E, Grzybowska ME, Hansdorfer-Korzon R, Zorena K. Inflammatory Markers in Dysmenorrhea and Therapeutic Options. Int J Environ Res Public Health 2020; [Crossref] [PubMed]
- Tu F, Hellman K. Primary Dysmenorrhea: Diagnosis and Therapy. Obstet Gynecol 2021;137:752. [Crossref] [PubMed]
- Liu P, Liu Y, Wang G, Yang X, Jin L, Sun J, Qin W. Aberrant default mode network in patients with primary dysmenorrhea: a fMRI study. Brain Imaging Behav 2017;11:1479-85. [Crossref] [PubMed]
- Tu CH, Niddam DM, Chao HT, Liu RS, Hwang RJ, Yeh TC, Hsieh JC. Abnormal cerebral metabolism during menstrual pain in primary dysmenorrhea. Neuroimage 2009;47:28-35. [Crossref] [PubMed]
- Dun WH, Yang J, Yang L, Ding D, Ma XY, Liang FL, von Deneen KM, Ma SH, Xu XL, Liu J, Zhang M. Abnormal structure and functional connectivity of the anterior insula at pain-free periovulation is associated with perceived pain during menstruation. Brain Imaging Behav 2017;11:1787-95. [Crossref] [PubMed]
- Yang L, Dun W, Li K, Yang J, Wang K, Liu H, Liu J, Zhang M. Altered amygdalar volume and functional connectivity in primary dysmenorrhoea during the menstrual cycle. Eur J Pain 2019;23:994-1005. [Crossref] [PubMed]
- May A. Experience-dependent structural plasticity in the adult human brain. Trends Cogn Sci 2011;15:475-82. [Crossref] [PubMed]
- Park SE, Kim BC, Yang JC, Jeong GW. MRI-Based Multimodal Approach to the Assessment of Clinical Symptom Severity of Obsessive-Compulsive Disorder. Psychiatry Investig 2020;17:777-85. [Crossref] [PubMed]
- Fox MD, Raichle ME. Spontaneous fluctuations in brain activity observed with functional magnetic resonance imaging. Nat Rev Neurosci 2007;8:700-11. [Crossref] [PubMed]
- Lefebvre G, Pinsonneault O, Antao V, Black A, Burnett M, Feldman K, Lea R, Robert M. Primary dysmenorrhea consensus guideline. J Obstet Gynaecol Can 2005;27:1117-46. [Crossref] [PubMed]
- Sundell G, Milsom I, Andersch B. Factors influencing the prevalence and severity of dysmenorrhoea in young women. Br J Obstet Gynaecol 1990;97:588-94. [Crossref] [PubMed]
- Health measurement scales: a practical guide to their development and use (5th edition). Aust N Z J Public Health 2016;40:294-5.
- Cox DJ, Meyer RG. Behavioral treatment parameters with primary dysmenorrhea. J Behav Med 1978;1:297-310. [Crossref] [PubMed]
- Dahnke R, Yotter RA, Gaser C. Cortical thickness and central surface estimation. Neuroimage 2013;65:336-48. [Crossref] [PubMed]
- Yotter RA, Nenadic I, Ziegler G, Thompson PM, Gaser C. Local cortical surface complexity maps from spherical harmonic reconstructions. Neuroimage 2011;56:961-73. [Crossref] [PubMed]
- Yotter RA, Thompson PM, Gaser C. Algorithms to improve the reparameterization of spherical mappings of brain surface meshes. J Neuroimaging 2011;21:e134-47. [Crossref] [PubMed]
- Yan CG, Wang XD, Zuo XN, Zang YF. DPABI: Data Processing & Analysis for (Resting-State) Brain Imaging. Neuroinformatics 2016;14:339-51. [Crossref] [PubMed]
- Schwedt TJ. Multisensory integration in migraine. Curr Opin Neurol 2013;26:248-53. [Crossref] [PubMed]
- Moulton EA, Becerra L, Maleki N, Pendse G, Tully S, Hargreaves R, Burstein R, Borsook D. Painful heat reveals hyperexcitability of the temporal pole in interictal and ictal migraine States. Cereb Cortex 2011;21:435-48. [Crossref] [PubMed]
- Bigler ED, Mortensen S, Neeley ES, Ozonoff S, Krasny L, Johnson M, Lu J, Provencal SL, McMahon W, Lainhart JE. Superior temporal gyrus, language function, and autism. Dev Neuropsychol 2007;31:217-38. [Crossref] [PubMed]
- Ruiz-Aranda D, Salguero JM, Fernández-Berrocal P. Emotional regulation and acute pain perception in women. J Pain 2010;11:564-9. [Crossref] [PubMed]
- Chen T, Mu J, Xue Q, Yang L, Dun W, Zhang M, Liu J. Whole-brain structural magnetic resonance imaging-based classification of primary dysmenorrhea in pain-free phase: a machine learning study. Pain 2019;160:734-41. [Crossref] [PubMed]
- Li M, Yan J, Li S, Wang T, Zhan W, Wen H, Ma X, Zhang Y, Tian J, Jiang G. Reduced volume of gray matter in patients with trigeminal neuralgia. Brain Imaging Behav 2017;11:486-92. [Crossref] [PubMed]
- Amin FM, De Icco R, Al-Karagholi MA, Raghava J, Wolfram F, Larsson HBW, Ashina M. Investigation of cortical thickness and volume during spontaneous attacks of migraine without aura: a 3-Tesla MRI study. J Headache Pain 2021;22:98. [Crossref] [PubMed]
- Leiner HC, Leiner AL, Dow RS. Cognitive and language functions of the human cerebellum. Trends Neurosci 1993;16:444-7. [Crossref] [PubMed]
- Maiti B, Koller JM, Snyder AZ, Tanenbaum AB, Norris SA, Campbell MC, Perlmutter JS. Cognitive correlates of cerebellar resting-state functional connectivity in Parkinson disease. Neurology 2020;94:e384-96. [Crossref] [PubMed]
- Diano M, D'Agata F, Cauda F, Costa T, Geda E, Sacco K, Duca S, Torta DM, Geminiani GC. Cerebellar Clustering and Functional Connectivity During Pain Processing. Cerebellum 2016;15:343-56. [Crossref] [PubMed]
- Silva KE, Rosner J, Ullrich NJ, Chordas C, Manley PE, Moulton EA. Pain affect disrupted in children with posterior cerebellar tumor resection. Ann Clin Transl Neurol 2019;6:344-54. [Crossref] [PubMed]
- Moulton EA, Elman I, Pendse G, Schmahmann J, Becerra L, Borsook D. Aversion-related circuitry in the cerebellum: responses to noxious heat and unpleasant images. J Neurosci 2011;31:3795-804. [Crossref] [PubMed]
- Labrenz F, Spisák T, Ernst TM, Gomes CA, Quick HH, Axmacher N, Elsenbruch S, Timmann D. Temporal dynamics of fMRI signal changes during conditioned interoceptive pain-related fear and safety acquisition and extinction. Behav Brain Res 2022;427:113868. [Crossref] [PubMed]
- Lorenz J, Minoshima S, Casey KL. Keeping pain out of mind: the role of the dorsolateral prefrontal cortex in pain modulation. Brain 2003;126:1079-91. [Crossref] [PubMed]
- Tracey I. Neuroimaging of pain mechanisms. Curr Opin Support Palliat Care 2007;1:109-16. [Crossref] [PubMed]
- Wei SY, Chao HT, Tu CH, Li WC, Low I, Chuang CY, Chen LF, Hsieh JC. Changes in functional connectivity of pain modulatory systems in women with primary dysmenorrhea. Pain 2016;157:92-102. [Crossref] [PubMed]
- Gilliam WP, Schumann ME, Cunningham JL, Evans MM, Luedtke CA, Morrison EJ, Sperry JA, Vowles KE. Pain catastrophizing as a treatment process variable in cognitive behavioural therapy for adults with chronic pain. Eur J Pain 2021;25:339-47. [Crossref] [PubMed]
- Bao S, Qiao M, Lu Y, Jiang Y. Neuroimaging Mechanism of Cognitive Behavioral Therapy in Pain Management. Pain Res Manag 2022;2022:6266619. [Crossref] [PubMed]
- Duque J, Greenhouse I, Labruna L, Ivry RB. Physiological Markers of Motor Inhibition during Human Behavior. Trends Neurosci 2017;40:219-36. [Crossref] [PubMed]
- Peyron R, Laurent B, García-Larrea L. Functional imaging of brain responses to pain. A review and meta-analysis (2000). Neurophysiol Clin 2000;30:263-88. [Crossref] [PubMed]
- Garza-Villarreal EA, Wilson AD, Vase L, Brattico E, Barrios FA, Jensen TS, Romero-Romo JI, Vuust P. Music reduces pain and increases functional mobility in fibromyalgia. Front Psychol 2014;5:90. [Crossref] [PubMed]
- Penner IK, Aktas O. Functional reorganization is a maladaptive response to injury - NO. Mult Scler 2017;23:193-4. [Crossref] [PubMed]
- Zou Y, Tang W, Qiao X, Li J. Aberrant modulations of static functional connectivity and dynamic functional network connectivity in chronic migraine. Quant Imaging Med Surg 2021;11:2253-64. [Crossref] [PubMed]
- Zhang Y, Huang Y, Liu N, Wang Z, Wu J, Li W, Xia J, Liu Z, Li Y, Hao Y, Huo J. Abnormal interhemispheric functional connectivity in patients with primary dysmenorrhea: a resting-state functional MRI study. Quant Imaging Med Surg 2022;12:1958-67. [Crossref] [PubMed]
- Goffaux P, Girard-Tremblay L, Marchand S, Daigle K, Whittingstall K. Individual differences in pain sensitivity vary as a function of precuneus reactivity. Brain Topogr 2014;27:366-74. [Crossref] [PubMed]
- Yang Y, Wang H, Zhou W, Qian T, Sun W, Zhao G. Electroclinical characteristics of seizures arising from the precuneus based on stereoelectroencephalography (SEEG). BMC Neurol 2018;18:110. [Crossref] [PubMed]
- Cavanna AE, Trimble MR. The precuneus: a review of its functional anatomy and behavioural correlates. Brain 2006;129:564-83. [Crossref] [PubMed]
- Hu C, Liu L, Liu L, Zhang J, Hu Y, Zhang W, Ding Y, Wang Y, Zhang Z, von Deneen KM, Qian L, Wang H, Duan S, Wang F, Cui G, Nie Y, Zhang Y. Cortical morphometry alterations in brain regions involved in emotional, motor-control and self-referential processing in patients with functional constipation. Brain Imaging Behav 2020;14:1899-907. [Crossref] [PubMed]
- Peng SL, Yang HC, Lee YC, Chen CM, Chen YY, Tu CH. Analgesia Effect of Verum and Sham Acupuncture Treatments in Primary Dysmenorrhea: A MRI Pilot Study. J Pers Med 2021; [Crossref] [PubMed]
- Martucci KT, Shirer WR, Bagarinao E, Johnson KA, Farmer MA, Labus JS, Apkarian AV, Deutsch G, Harris RE, Mayer EA, Clauw DJ, Greicius MD, Mackey SC. The posterior medial cortex in urologic chronic pelvic pain syndrome: detachment from default mode network-a resting-state study from the MAPP Research Network. Pain 2015;156:1755-64. [Crossref] [PubMed]
- Rolls ET. The orbitofrontal cortex and emotion in health and disease, including depression. Neuropsychologia 2019;128:14-43. [Crossref] [PubMed]
- Rolls ET, Grabenhorst F. The orbitofrontal cortex and beyond: from affect to decision-making. Prog Neurobiol 2008;86:216-44. [Crossref] [PubMed]
- Wu TH, Tu CH, Chao HT, Li WC, Low I, Chuang CY, Yeh TC, Cheng CM, Chou CC, Chen LF, Hsieh JC. Dynamic Changes of Functional Pain Connectome in Women with Primary Dysmenorrhea. Sci Rep 2016;6:24543. [Crossref] [PubMed]
- Liu P, Yang J, Wang G, Liu Y, Liu X, Jin L, Liang F, Qin W, Calhoun VD. Altered regional cortical thickness and subcortical volume in women with primary dysmenorrhoea. Eur J Pain 2016;20:512-20. [Crossref] [PubMed]
- Soheili-Nezhad S, Sedghi A, Schweser F, Eslami Shahr Babaki A, Jahanshad N, Thompson PM, Beckmann CF, Sprooten E, Toghae M. Structural and Functional Reorganization of the Brain in Migraine Without Aura. Front Neurol 2019;10:442. [Crossref] [PubMed]
- Dehaene S, Changeux JP. Experimental and theoretical approaches to conscious processing. Neuron 2011;70:200-27. [Crossref] [PubMed]
- Lau H, Rosenthal D. Empirical support for higher-order theories of conscious awareness. Trends Cogn Sci 2011;15:365-73. [Crossref] [PubMed]
- Da Silva JT, Seminowicz DA. Neuroimaging of pain in animal models: a review of recent literature. Pain Rep 2019;4:e732. [Crossref] [PubMed]
- Apkarian AV. A brain signature for acute pain. Trends Cogn Sci 2013;17:309-10. [Crossref] [PubMed]
- Amaro-Díaz L, Montoro CI, Fischer-Jbali LR, Galvez-Sánchez CM. Chronic Pain and Emotional Stroop: A Systematic Review. J Clin Med 2022; [Crossref] [PubMed]
- Koechlin H, Coakley R, Schechter N, Werner C, Kossowsky J. The role of emotion regulation in chronic pain: A systematic literature review. J Psychosom Res 2018;107:38-45. [Crossref] [PubMed]
- Maulitz L, Stickeler E, Stickel S, Habel U, Tchaikovski SN, Chechko N. Endometriosis, psychiatric comorbidities and neuroimaging: Estimating the odds of an endometriosis brain. Front Neuroendocrinol 2022;65:100988. [Crossref] [PubMed]