Relationship between chronic kidney disease and cerebral white matter hyperintensities: a systematic review
Introduction
The kidneys can be affected by a wide range of disease conditions including oncological, infectious, traumatic, and degenerative disorders (1-4).
Chronic kidney disease (CKD), also known as chronic kidney failure, is characterized by progressive tissue damage and gradual loss of kidney function. CKD is a common pathological condition and is most frequently encountered in elderly individuals. Diabetes and hypertension are the main causes of CKD in both developed and developing countries; glomerulonephritis and unknown causes are more common in countries of sub-Saharan Africa and Asia (5).
In the early stages, there may be few signs or symptoms, but when the condition becomes more advanced, CKD can exhibit systemic effects beyond the kidneys. Intriguingly, a relationship between renal function and brain structural alterations has been suggested (6). Indeed, CKD-related indicators such as albuminuria and reduced estimated glomerular filtration rate (eGFR) are considered risk factors for stroke, dementia, and death (7,8).
Computed tomography (CT) and magnetic resonance imaging (MRI) are non-invasive tools for qualitative and quantitative assessment of tissues (9-13). On T2-weighted turbo spin echo (TSE) or fluid-attenuated inversion recovery (FLAIR) cerebral MRI scans, scattered or confluent white matter hyperintensities (WMH) are commonly observed, especially in elderly individuals (14-16). These are known as a marker of cerebral small vessel disease and have been linked to an increased risk of stroke, dementia, and death (14-16).
A recent meta-analysis of 16 case-control studies found that subjects with an eGFR <60 mL/min/1.73 m2 (stage 3 CKD or moderate CKD) show an increased incidence of WMH compared to subjects with an eGFR >60 mL/min/1.73 m2 (17). Moreover, cross-sectional observational studies have found a correlation between WMH volumes, quantified using automated techniques, and urinary albumin levels (18,19).
Many studies have assessed WMH using the Fazekas scale, a semi-quantitative, non-volumetric method, proposed by Fazekas et al. in 1987, to simply describe the amount of cerebral white matter T2 hyperintense lesions (20). The Fazekas scale divides the white matter into the two regions, periventricular and deep white matter; each region is graded according to the size and confluence of the lesions (Figure 1) (20).
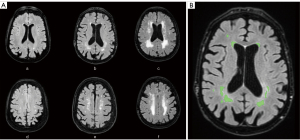
Another approach to measure WMH is the volumetric quantification, which tends to be less subjective, more sensitive, and effective (21-23).
The present systematic review focuses on WMH distribution and volumetric quantification in patients with chronic renal failure. The aim of this paper is to evaluate the available evidence about the impact of chronic renal failure on brain health as evaluated by means of MRI-based volumetric analysis of WMH.
Methods
This systematic review was performed in accordance with the Preferred Reporting Items for Systematic Reviews and Meta-Analyses (PRISMA) guidelines (24,25), the PRISMA reporting checklist is available at https://qims.amegroups.com/article/view/10.21037/qims-22-707/rc.
Search strategy
The literature search was carried out in March 2022 using MEDLINE PubMed Central, Scopus, and Web of Science – Publons. Only articles written in English, without limits of time span, were included. The keywords used for articles search were: “renal failure white matter hyperintensities”, “chronic renal failure white matter hyperintensities”, “chronic kidney disease white matter hyperintensities”, “end stage renal disease white matter hyperintensities”, “renal insufficiency white matter hyperintensities” and “renal disfunction white matter hyperintensities”.
Eligibility criteria
The eligibility criteria were based on study design and on the method of analysis of WMH. Articles included were focused on the association between chronic renal failure and WMH performed by volumetric analysis. Therefore, studies assessing chronic renal failure and non-volumetric WMH analysis (e.g., Fazekas scale), were excluded. Cross-sectional, prospective cohort and retrospective studies were included. No limit was set on the aim of the studies.
Data extraction and risk of bias assessment
Two reviewers independently performed the search (FG and CAM). All peer-reviewed journals were screened, and all relevant studies were explored. The first step was to rule out duplicates. All the titles and abstracts were then evaluated considering the inclusion criteria by two authors (FG and CAM). Full text was evaluated when the title and abstract did not provide adequate information regarding the inclusion criteria.
Disagreement regarding the inclusion or exclusion of an article was assessed through discussion between the two reviewers (FG and CAM), until a consensus was reached. In no cases was there a need for the opinion of a third reviewer.
The estimation of the potential bias was performed using assessment criteria established by the Quality Assessment of Diagnostic Accuracy Studies (QUADAS-2) (26).
To avoid inaccuracies and inconsistencies, selected articles were evaluated independently by two reviewers (FG and CAM).
Results
The database search strategy yielded 987 articles (Figure 2). After removal of duplicates, the titles, and abstracts of the remaining 320 articles were scrutinized. Consequently, 276 articles were excluded as the abstract and title were not relevant to the topic of this systematic review. Of the 44 articles assessed for eligibility, 36 were excluded as the quantitative analysis of WMH was not a volumetric analysis. Finally, 8 articles were included in the systematic review. Most of these studies showed a level of evidence of 1. For the results summary of the individual studies please see Table 1.
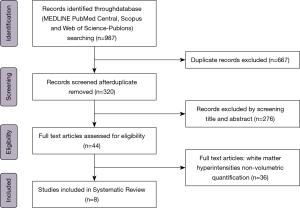
Table 1
Authors | CKD parameters | WMH data | Brain MRI sequence | WMH quantification software | Number of subjects |
Results | Level of evidence* |
---|---|---|---|---|---|---|---|
Khatri et al., 2007 (27) | Serum creatinine; creatinine clearance; eGFR | Log-WMH volume | FLAIR | Quantum 6.2 package on a Sun Microsystems Ultra 5 workstation | 615 | Creatinine clearance: P<0.001; eGFR: P<0.001 | 3 |
Turner et al., 2011 (28) | Serum creatinine; UACR | WMH volume | FLAIR | Not specified | 1,585 | Serum creatinine correlation coefficient: 0.54; UACR correlation coefficient: 0.52 | 3 |
Vemuri et al., 2017 (29) | eGFR; UACR | WMH volume | FLAIR | Statistical Parametric Mapping | 240 | eGFR: P=0.002; UACR: P<0.001 | 1 |
Bronas et al., 2019 (30) | eGFR | WMH volume | BRAVO; FLAIR | Support vector machine classifier | 94 | eGFR: b=0.009 | 1 |
Marini et al., 2020 (31) | eGFR; UACR; pleiotropic locus 2q33; other pleiotropic loci | WMH volume | T1 FLAIR; T2 FLAIR |
Summary statistics of GWAS analysis for total volume of WMH derived by the UK Biobank study | 10,597 | eGFR: P=0.177; UACR: P=0.020; pleiotropic locus 2q33 posterior probabilities of association of 0.80; no statistically significant correlations with other pleiotropic loci | 3 |
Yamasaki et al., 2020 (32) |
eGFR; UACR | IC volume-corrected WMH volume | T1-weighted FLAIR | LST for SPM12 | 1,214 | No statistically significant correlation for eGFR; UACR: P=0.01 | 1 |
Vemuri et al., 2021 (33) | eGFR; UACR | WMH volume | FLAIR | Statistical Parametric Mapping | 242 | No statistically significant correlations for eGFR and UACR | 1 |
Bijkerk et al., 2022 (34) | Angiopoietin-2 Asymmetric dimethylarginine miR-27a; miR-29a; miR-126; miR-132; miR-137; miR-192; miR-223; miR-326 | WMH volume | Not specified | Quantib brain, Rotterdam, the Netherlands | 70 | Angiopoietin-2: P=0.016; no statistically significant correlations with the remaining data | 1 |
*, SIGN100: Scottish Intercollegiate Guidelines Network 2019. CKD, chronic kidney disease; WMH, white matter hyperintensities; MRI, magnetic resonance imaging; eGFR, estimated glomerular filtration rate; FLAIR, fluid-attenuated inversion; UACR, urine albumin to creatinine ratio; BRAVO, axial MRI 3D brain volume; LST, Lesion Segmentation Toolbox.
The estimation of potential risk of bias is summarized in Figure 3.
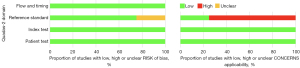
Discussion
The (potential) link between cerebral WMH volume and chronic renal failure has been an interesting research target in recent years. Several studies have attempted to fill this knowledge gap. The major indicators of CKD applied in such studies were creatinine clearance, serum creatinine, eGFR, and UACR.
Creatinine clearance and white matter hyperintensities volume
Of note is the Northern Manhattan Study in a community-based cohort of stroke-free subjects undergoing MRI (26). In this study, published in 2007, the authors performed volumetric analysis of cerebral WMH through an MRI-based approach. The relationship between renal function and log-WMH volume adjusted for gender, age, race-ethnicity, cardiac disease, homocysteine, hypertension, diabetes, and education was assessed using linear regression. A cohort of 615 subjects with an average age of 70 years were enrolled. Multivariate analysis showed a relationship between creatinine clearance 15 to 60 mL/min and increased log-WMH volume (β =0.322; 95% CI: 0.095 to 0.550). Interestingly, increased creatinine per 1-mg/dL showed a positive correlation with log-WMH volume (β =1,479; 95% CI: 1,067 to 2,050). This association highlights the importance of kidney disease as a determinant of cerebrovascular damage and/or as a biomarker for microangiopathy (27).
Serum creatinine, glomerular filtration rate and white matter hyperintensities volume
Another large study by Turner et al. investigated the relationship between serum creatinine and cerebral small vessel arteriosclerosis assessed by quantifying the WMH volume on brain MRI and with peripheral large vessel arteriosclerosis determined by the ankle-brachial index (28). Sibling members (804 Caucasians and 781 Afro-Americans) of whom at least two siblings had primary hypertension, were enrolled in the study. After adjusting for age, the authors found an increase in serum creatinine with greater WMH volume (correlation coefficient of 0.5) and with decreased ankle-brachial index (0.50; P<0.001) (28).
The relationship between biomarkers of renal function and gray matter volume, cerebrovascular conditions (i.e., WMH, cerebral infarctions, micro-hemorrhages), and microstructural changes assessed by brain MRI in CKD patients was investigated by Vemuri et al. (29). Volumetric quantification of WMH was performed using an automated method on FLAIR images. Diffusion tensor imaging was used for the evaluation of white matter microstructural changes. Urine albumin to creatinine ratio (UACR) and eGFR joint models including both predictors were adjusted for vascular risk factors by linear regression. The 240 brain MRI scans analyzed showed higher WMH volume, a greater likelihood of cortical infarcts, and worsening of diffusion changes throughout the brain in subjects with lower eGFR. In the analysis adjusted with the regression model a 10% decrease in and GFR was associated with a 2.8% increase in WMH volume, in the joint model was detected at trend level effects of eGFR (P=0.09) (29).
Bronas et al. analyzed indicators of impaired renal function, sedentary time and WMH in 94 healthy non-demented and non-depressed adults (30). Renal function was evaluated with the epi-CKD formula for eGFR. WMH volumes quantification was performed by affine registration of the T1-weighted axial MRI 3D brain volume (BRAVO) data to the T2-weighted FLAIR data. WMH volumes were segmented automatically and corrected by the participant’s corresponding intracranial volume to account for individual differences in head size. This led to a measurement of the WMH volumes as a percentage of the intracranial volume.
Adjusting for age, sex, education, Framingham stroke risk 10-year prediction score, sedentary attitude was related to increased WMH volume, but this effect depended upon eGFR (sedentary time * eGFR interaction b=−0.0005, P=0.022). At eGFR 25th, 50th, and 75th percentiles, sedentary time b coefficients were b=0.021 (95% CI: 0.011–0.031), b=0.015 (95% CI: 0.008–0.022), and b=0.009 (95% CI: 0.003–0.016). The results of this study suggest that sedentary time is associated with WMH volumes in persons with an eGFR ≤96 mL/min/1.73 m2 and that this relation is stronger with lower levels of renal function (30). Sedentary time may impair vascular brain function by increasing inflammation and oxidative stress and reducing angiogenesis, neurogenesis and/or synaptogenesis (35).
Marini et al. reported genetic data aimed to identify a correlation between impaired renal function and phenotypes of cerebrovascular diseases (31). Summary statistics from genome-wide association studies of renal function traits (CKD diagnosis, eGFR, UACR) and cerebrovascular disease phenotypes (i.e., ischemic stroke and respective subtypes, intracerebral hemorrhage, and WMH on brain MRI) were used. WMH volume was analyzed using summary statistics of genome-wide association studies analysis for WMH volume derived by the UK Biobank study. Volumetric analysis was performed on a very large sample of 10597 participants of European ancestry using T1 and T2 FLAIR images. Sex, age and principal components were used for adjusting linear regression model of the genome-wide association studies. Poligenic risk score for lower eGFR was related with higher large artery stroke risk (P=1×10−4). Pleiotropic locus 12q24 was associated with eGFR and both large artery stroke and small vessel stroke. Moreover, mendelian randomization demonstrated a correlation with lower eGFR [odds ratio for 1-log decrement (1-log decrease the value to the power of 1), 2.10; 95% CI: 1.38–3.21] and higher UACR [odds ratio per 1-log increment (1-log increment the value to the power of 1), 2.35; 95% CI: 1.12–4.94], with a higher risk of large artery stroke. It should be highlighted that this study showed, on a very large sample, the genetic relationship between impaired renal function and cerebrovascular pathologies across the genome and pleiotropic loci. Impaired hemofiltration and glomerular function appear to play a causal role in stroke, possibly contributing to the greater atherosclerosis. The authors also showed that the pleiotropic locus 2q33 may play a role in pathologies of the small vessels of the kidney and brain through microalbuminuria, small vessel stroke and WMH. This locus encodes the ICA1L, WDR12, and NBEAL1 genes and have been linked to both small vessel stroke risk and WMH (31). This study suggested a common genetic fingerprint between CKD and WMH volume. Pleiotropic analysis across the 12q24.12 locus showed an association between eGFR and the risk of large artery stroke. In particular, the mutation of the SH2B3 gene, found in this locus, has been associated with high blood pressure, a known risk factor for both stroke and kidney disease, which could explain part of the shared gene predisposition between the two entities (36).
Yamasaki et al. investigated the association between albuminuria and WMH in elderly Japanese (32). Japanese subjects (n=1,214) aged ≥65 years underwent brain MRI to evaluate WMH volume together intracranial volume ratio (i.e., IC volume-corrected WMH volume). T1-weighted and FLAIR images were used for the quantification of WMH volume. IC volume-corrected WMH volume was associated with eGFR without detecting any correlation between the two parameters (32).
A further study by Vemuri and colleagues assessed the impact that CKD can have on brain health through correlations between eGFR and cortical thickness, ventricular volume, WMH volume and fractional anisotropy of the corpus callosum on 242 subjects using longitudinal imaging (33). Cortical thickness and ventricular volume were quantified on MPRAGE images, while WMH volume was measured on FLAIR images by semi-automatic segmentation. The authors considered fraction anisotropy in the corpus callosum as diffusor tensor imaging metric because it is an important anatomical structure for cognitive performance and is influenced by vascular health. The results showed an increased WMH volume and a decreased anisotropy fraction in corpus callosum in CKD participants (both P<0.001). Once again, another study demonstrating the relationship between renal function (i.e., eGFR) and white matter damage in CKD patients (33). These results support the hypothesis that CKD progression affects cognitive function at least partially by means of small vessel disease-related mechanisms. White matter changes found in aging and dementia are usually due to small vessel disease in the elderly. Relative causes are variable and include hypoperfusion, blood-brain barrier dysfunction, altered water content, myelin damage, and axonal disruption (37).
Lastly, a very recent study by Bijkerk et al. investigated the relationship between levels of Angiopoietin-2, asymmetric dimethylarginine, circulating angiogenic miRNAs (i.e., miR-27a, miR-29a, miR-126, miR-132, miR-137, miR-192, miR-223 and miR-326) and small vessel disease together with cognitive impairment in 129 end stage renal disease older patients (mean age 75.3 years; mean eGFR 16.4 mL/min). Changes in small vessel disease (WMH volume, microbleeds and lacunes) were evaluated by brain MRI, while neuropsychological tests were used to evaluate cognition in domains of memory, executive functions, and psychomotor speed (34). Brain MRI was available on 70 of 129 enrolled patients, WMH volume was quantified using an automated method after manually segmenting other pathologies. Furthermore, WMH were assessed using the Scheltens scale, which is considered a more detailed visual scale than the Fazekas scale, while lacunes and microbleeds were evaluated using the STRIVE criteria (38,39). Angiopoietin-2 showed a correlation with small vessel disease, both with WMH volume (P=0.016) and with the Scheltens score (P=0.08). Furthermore, angiopoietin-2 showed a statistically significant correlation with executive function and psychomotor speed, while miR-223 and miR-29a showed an association with memory functions. These angiogenic markers could be used to identify the risk of cerebral small vessel disease and cognitive decline in patients with end stage renal disease (34).
Urine albumin to creatinine ratio and white matter hyperintensities volume
Turner et al. evaluated the relationship between UACR and cerebral small vessel arteriosclerosis, finding a correlation between the increase in UACR with the increase in WMH volume (correlation coefficient of 0.52) and with decreased ankle-brachial index (0.54; P<0.001) (28). This study offered additional evidence on the link between CKD and WMH volume (28).
The correlation between the WMH volume calculated on 240 brain MRI scans and the UACR performed by Vemuri et al. showed a link between these two parameters. In particular in the analysis adjusted with the regression model a 10% increase in UACR was associated with a 0.32% increase in WMH volume, In the joint model the effect of UACR was significant (P=0.09) (29). The importance of this study is also highlighted by the higher number of MRI-based brain variables evaluated and by the demonstration of the relationship between CKD and cerebrovascular pathologies together with microstructural brain changes (29).
The analysis performed by Marini et al. between UACR and cerebrovascular disease phenotypes found a correlation between the Pleiotropic locus 2q33 with UACR and both small vessel stroke and WMH volume. While mendelian randomization demonstrated a correlation with higher UACR (odds ratio per 1-log increment, 2.35; 95% CI: 1.12–4.94), with a higher risk of large artery stroke and greater risk of intracerebral hemorrhage (31).
The correlation between IC volume-corrected WMH volume with UACR performed by Yamasaki et al. was associated with cognitive decline and mortality risk. The whole population showed a geometric mean of IC volume-corrected WMH volume ratio of 0.223%. This geometric mean increased significantly with greater UACR after adjusting for confounding factors (0.213% with normoalbuminuria, 0.248% with microalbuminuria, and 0.332% with macroalbuminuria; Ptrend=0.01). Moreover, subjects with albuminuria and higher IC volume-corrected WMH volume [≥0.257% (median)] were more likely to develop cognitive decline at baseline or all-cause death during follow-up than subjects with normal albuminuria and low IC volume-corrected WMH volume [<0.257% (median)]. This evidence highlighted that elevated albuminuria levels are associated with larger WMH volume in an elderly Japanese population. Albuminuria levels were related to WMH enlargement with the increased risk of cognitive decline and all-cause mortality suggesting that urinary albumin levels may be useful for WMH risk assessment, and can be critical in the prevention of stroke, dementia, end-stage renal failure and death (32).
Albuminuria can be a marker of risk factors for WMH such as hypertension and diabetes, but also of endothelial dysfunction in the kidney (40). The brain and kidney are hemodynamically similar since they are perfused by small vessels directly arising from large blood vessels and thus are perfused by large amounts of blood with low vascular resistance (41,42). In this way, these two organs are vulnerable to vascular damage when exposed to high pressure (41,42).
The study conducted by Vemuri et al. found no correlation between the increase in UACR and changes in WMH volume (33).
Future perspectives
A novel and interesting concept that is being studied is that the brain can be affected by systemic conditions or disorders, such as in the case of CKD. The literature is still sparse and available studies on the topic are extremely heterogeneous in terms of methodology and samples. However, evidence is accumulating that abnormal function of the abdominal organs, such as the kidney, can affect the brain and be related to cerebral small vessel disease and be associated to an increased WMH volume load. This is likely mediated by accumulation of circulating substances possibly exhibiting toxic effects on small vessels and white matter of the brain. However, additional effort in needed to improve standardization of methodologies across studies, to increase the accuracy with different techniques to analyze brain activity such as functional MRI and electroencephalography, and to understand the impact of CKD on the risk of developing various brain diseases such as neurogenerative disorders (43-45).
Moreover, hypertension and diabetes, risk factors for CKD, can also cause microstructural and macrostructural damage to the white matter. Hypertension is considered a classic risk factor for WMH. Indeed, a Biobank cohort study demonstrated a linear association between neuroimaging biomarkers (i.e., WMH volume and diffusion imaging indices) and mean arterial and pulse pressures (46). In addition to hypertension, many studies suggest that diabetes mellitus may be associated with WMH progression and, furthermore, insulin resistance is thought to be an exacerbating factor along with other risk factors such as dyslipidemia, smoking, or other vascular risk factors (47,48).
This systematic review found no studies that performed the volumetric analysis of WMH in CKD dialysis patients. The link between WMH and CKD in dialysis patients compared to healthy subjects is known (49). Further studies would be helpful to quantify WMH in dialysis patients by means of volumetric analysis and better define the relationship among CKD, dialysis and WMH. Furthermore, it would be interesting to assess with future systematic reviews and meta-analyses studies dealing with the relationship between CKD and the full spectrum of cerebral small vessel diseases in order to better understand the underlying pathogenetic mechanisms.
Conclusions
Our systematic review reveals that the literature on the topic of CKD and WMH volume is quite heterogeneous. Despite this heterogeneity, we found evidence that there is a clear link between CKD and cerebral WMH volume. Our finding implies that, in all studies regarding cerebral WMH imaging findings, it is of great importance to take renal function into consideration. Data such as eGFR and UACR should be included as variables to better understand the pathogenesis of cerebral WMH, and to identify patients with CKD at the risk of cerebrovascular disease. The progressive decline in glomerular filtration rate in CKD patients causes a wide range of physiological, biochemical and hematological changes which may impact the cerebral blood vessels and particularly the microvascular environment in the white matter. These biological and molecular mechanisms underlying cerebrovascular damage in patients with chronic renal failure deserve to be further explored.
Acknowledgments
Funding: None.
Footnote
Provenance and Peer Review: With the arrangement by the Guest Editors and the editorial office, this article has been reviewed by external peers.
Reporting Checklist: The authors have completed the PRISMA reporting checklist. Available at https://qims.amegroups.com/article/view/10.21037/qims-22-707/rc
Conflicts of Interest: All authors have completed the ICMJE uniform disclosure form (available at https://qims.amegroups.com/article/view/10.21037/qims-22-707/coif). The special issue “Imaging of Aging and Age-Related Disorders” was commissioned by the editorial office without any funding or sponsorship. CAM served as the unpaid Guest Editor of the issue and serves as an unpaid editorial board member of Quantitative Imaging in Medicine and Surgery. The authors have no other conflicts of interest to declare.
Ethical Statement: The authors are accountable for all aspects of the work in ensuring that questions related to the accuracy or integrity of any part of the work are appropriately investigated and resolved.
Open Access Statement: This is an Open Access article distributed in accordance with the Creative Commons Attribution-NonCommercial-NoDerivs 4.0 International License (CC BY-NC-ND 4.0), which permits the non-commercial replication and distribution of the article with the strict proviso that no changes or edits are made and the original work is properly cited (including links to both the formal publication through the relevant DOI and the license). See: https://creativecommons.org/licenses/by-nc-nd/4.0/.
References
- Greco F, Mallio CA, Grippo R, Messina L, Vallese S, Rabitti C, Quarta LG, Grasso RF, Beomonte Zobel B. Increased visceral adipose tissue in male patients with non-clear cell renal cell carcinoma. Radiol Med 2020;125:538-43. [Crossref] [PubMed]
- Greco F, Cirimele V, Mallio CA, Beomonte Zobel B, Grasso RF. Increased visceral adipose tissue in male patients with clear cell renal cell carcinoma. Clin Cancer Investig J 2018;7:132-6.
- Yip SWY, Li YL, Chu YLE, Mak JYH, Li JYY, Lee KH, Lee R. Adrenal and renal abscesses following glue embolization of gastric varices: a case description. Quant Imaging Med Surg 2020;10:1566-9. [Crossref] [PubMed]
- Loffroy R, Chevallier O, Gehin S, Midulla M, Berthod PE, Galland C, Briche P, Duperron C, Majbri N, Mousson C, Falvo N. Endovascular management of arterial injuries after blunt or iatrogenic renal trauma. Quant Imaging Med Surg 2017;7:434-42. [Crossref] [PubMed]
- Engelgau MM, El-Saharty S, Kudesia P, Rajan V, Rosenhouse S, Okamoto K. Regional aging and disease burden. In: Capitalizing on the demographic transition: tackling noncommunicable diseases in South Asia. Washington, DC: World Bank, 2011:15-40.
- Cho EB, Shin HY, Park SE, Chun P, Jang HR, Yang JJ, Kim HJ, Kim YJ, Jung NY, Lee JS, Lee J, Jang YK, Jang EY, Kang M, Lee JM, Kim C, Min JH, Ryu S, Na DL, Seo SW. Albuminuria, Cerebrovascular Disease and Cortical Atrophy: among Cognitively Normal Elderly Individuals. Sci Rep 2016;6:20692. [Crossref] [PubMed]
- Vogels SC, Emmelot-Vonk MH, Verhaar HJ, Koek HL. The association of chronic kidney disease with brain lesions on MRI or CT: a systematic review. Maturitas 2012;71:331-6. [Crossref] [PubMed]
- Abramson JL, Jurkovitz CT, Vaccarino V, Weintraub WS, McClellan W. Chronic kidney disease, anemia, and incident stroke in a middle-aged, community-based population: the ARIC Study. Kidney Int 2003;64:610-5. [Crossref] [PubMed]
- Gabin JM, Romundstad S, Saltvedt I, Holmen J. Moderately increased albuminuria, chronic kidney disease and incident dementia: the HUNT study. BMC Nephrol 2019;20:261. [Crossref] [PubMed]
- Greco F, Mallio CA, Cirimele V, Grasso RF, Zobel BB. Subcutaneous adipose tissue as a biomarker of pancreatic cancer: A pilot study in male patients. Clin Cancer Investig J 2019;8:114-8.
- Greco F, Mallio CA. Artificial intelligence and abdominal adipose tissue analysis: a literature review. Quant Imaging Med Surg 2021;11:4461-74. [Crossref] [PubMed]
- Wang YX, Ng CK. The impact of quantitative imaging in medicine and surgery: Charting our course for the future. Quant Imaging Med Surg 2011;1:1-3. [Crossref] [PubMed]
- Del Buono R, Sabatino L, Greco F. Neck fat volume as a potential indicator of difficult intubation: A pilot study. Saudi J Anaesth 2018;12:67-71. [Crossref] [PubMed]
- Vermeer SE, Hollander M, van Dijk EJ, Hofman A, Koudstaal PJ, Breteler MM. Silent brain infarcts and white matter lesions increase stroke risk in the general population: the Rotterdam Scan Study. Stroke 2003;34:1126-9. [Crossref] [PubMed]
- Vermeer SE, Prins ND, den Heijer T, Hofman A, Koudstaal PJ, Breteler MM. Silent brain infarcts and the risk of dementia and cognitive decline. N Engl J Med 2003;348:1215-22. [Crossref] [PubMed]
- Yilmaz P, Ikram MK, Niessen WJ, Ikram MA, Vernooij MW. Practical Small Vessel Disease Score Relates to Stroke, Dementia, and Death. Stroke 2018;49:2857-65. [Crossref] [PubMed]
- Liu Y, Lv P, Jin H, Cui W, Niu C, Zhao M, Fan C, Teng Y, Pan B, Peng Q, Luo J, Zheng L, Huang Y. Association between Low Estimated Glomerular Filtration Rate and Risk of Cerebral Small-Vessel Diseases: A Meta-Analysis. J Stroke Cerebrovasc Dis 2016;25:710-6. [Crossref] [PubMed]
- Strickland AL, Rossetti HC, Peshock RM, Weiner MF, Nakonezny PA, McColl RW, Hulsey KM, Das SR, King KS. Urinary albumin to creatinine ratio as potential biomarker for cerebral microvascular disease. Curr Neurovasc Res 2014;11:242-7. [Crossref] [PubMed]
- Akoudad S, Sedaghat S, Hofman A, Koudstaal PJ, van der Lugt A, Ikram MA, Vernooij MW. Kidney function and cerebral small vessel disease in the general population. Int J Stroke 2015;10:603-8. [Crossref] [PubMed]
- Fazekas F, Chawluk JB, Alavi A, Hurtig HI, Zimmerman RA. MR signal abnormalities at 1.5 T in Alzheimer's dementia and normal aging. AJR Am J Roentgenol 1987;149:351-6. [Crossref] [PubMed]
- Davis Garrett K, Cohen RA, Paul RH, Moser DJ, Malloy PF, Shah P, Haque O. Computer-mediated measurement and subjective ratings of white matter hyperintensities in vascular dementia: relationships to neuropsychological performance. Clin Neuropsychol 2004;18:50-62. [Crossref] [PubMed]
- van Straaten EC, Fazekas F, Rostrup E, Scheltens P, Schmidt R, Pantoni L, Inzitari D, Waldemar G, Erkinjuntti T, Mäntylä R, Wahlund LO, Barkhof F. Impact of white matter hyperintensities scoring method on correlations with clinical data: the LADIS study. Stroke 2006;37:836-40. [Crossref] [PubMed]
- Andere A, Jindal G, Molino J, Collins S, Merck D, Burton T, Stretz C, Yaghi S, Sacchetti DC, Jamal SE, Reznik ME, Furie K, Cutting S. Volumetric White Matter Hyperintensity Ranges Correspond to Fazekas Scores on Brain MRI. J Stroke Cerebrovasc Dis 2022;31:106333. [Crossref] [PubMed]
- Liberati A, Altman DG, Tetzlaff J, Mulrow C, Gøtzsche PC, Ioannidis JP, Clarke M, Devereaux PJ, Kleijnen J, Moher D. The PRISMA statement for reporting systematic reviews and meta-analyses of studies that evaluate healthcare interventions: explanation and elaboration. BMJ 2009;339:b2700. [Crossref] [PubMed]
- Moher D, Liberati A, Tetzlaff J, Altman DG; . Preferred reporting items for systematic reviews and meta-analyses: the PRISMA statement. Ann Intern Med 2009;151:264-9, W64.
- Whiting PF, Rutjes AW, Westwood ME, Mallett S, Deeks JJ, Reitsma JB, Leeflang MM, Sterne JA, Bossuyt PM. QUADAS-2: a revised tool for the quality assessment of diagnostic accuracy studies. Ann Intern Med 2011;155:529-36. [Crossref] [PubMed]
- Khatri M, Wright CB, Nickolas TL, Yoshita M, Paik MC, Kranwinkel G, Sacco RL, DeCarli C. Chronic kidney disease is associated with white matter hyperintensity volume: the Northern Manhattan Study (NOMAS). Stroke 2007;38:3121-6. [Crossref] [PubMed]
- Turner ST, Rule AD, Schwartz GL, Kullo IJ, Mosley TH, Jack CR, Kardia SL, Boerwinkle E, Bailey KR. Risk factor profile for chronic kidney disease is similar to risk factor profile for small artery disease. J Hypertens 2011;29:1796-801. [Crossref] [PubMed]
- Vemuri P, Knopman DS, Jack CR Jr, Lundt ES, Weigand SD, Zuk SM, Thostenson KB, Reid RI, Kantarci K, Slinin Y, Lakshminarayan K, Davey CS, Murray A. Association of Kidney Function Biomarkers with Brain MRI Findings: The BRINK Study. J Alzheimers Dis 2017;55:1069-82. [Crossref] [PubMed]
- Bronas UG, Steffen A, Dion C, Boots EA, Arfanakis K, Marquez DX, Lamar M. Sedentary Time and White Matter Hyperintensity Volume in Older Adults. Med Sci Sports Exerc 2019;51:1613-8. [Crossref] [PubMed]
- Marini S, Georgakis MK, Chung J, Henry JQA, Dichgans M, Rosand J, Malik R, Anderson CD. Genetic overlap and causal inferences between kidney function and cerebrovascular disease. Neurology 2020;94:e2581-91. [Crossref] [PubMed]
- Yamasaki K, Hata J, Furuta Y, Hirabayashi N, Ohara T, Yoshida D, Hirakawa Y, Nakano T, Kitazono T, Ninomiya T. Association of Albuminuria With White Matter Hyperintensities Volume on Brain Magnetic Resonance Imaging in Elderly Japanese - The Hisayama Study. Circ J 2020;84:935-42. [Crossref] [PubMed]
- Vemuri P, Davey C, Johansen KL, Zuk SM, Reid RI, Thostenson KB, Reddy AL, Jack CR, Knopman DS, Murray AM. Chronic Kidney Disease Associated with Worsening White Matter Disease and Ventricular Enlargement. J Alzheimers Dis 2021;83:1729-40. [Crossref] [PubMed]
- Bijkerk R, Kallenberg MH, Zijlstra LE, van den Berg BM, de Bresser J, Hammer S, Bron EE, Achterberg H, van Buchem MA, Berkhout-Byrne NC, Bos WJW, van Heemst D, Rabelink TJ, van Zonneveld AJ, van Buren M, Mooijaart S. Circulating angiopoietin-2 and angiogenic microRNAs associate with cerebral small vessel disease and cognitive decline in older patients reaching end-stage renal disease. Nephrol Dial Transplant 2022;37:498-506. [Crossref] [PubMed]
- Bronas UG, Puzantian H, Hannan M. Cognitive Impairment in Chronic Kidney Disease: Vascular Milieu and the Potential Therapeutic Role of Exercise. Biomed Res Int 2017;2017:2726369. [Crossref] [PubMed]
- Evangelou E, Warren HR, Mosen-Ansorena D, Mifsud B, Pazoki R, Gao H, et al. Genetic analysis of over 1 million people identifies 535 new loci associated with blood pressure traits. Nat Genet 2018;50:1412-25. [Crossref] [PubMed]
- Alber J, Alladi S, Bae HJ, Barton DA, Beckett LA, Bell JM, et al. White matter hyperintensities in vascular contributions to cognitive impairment and dementia (VCID): Knowledge gaps and opportunities. Alzheimers Dement (N Y) 2019;5:107-17. [Crossref] [PubMed]
- Scheltens P, Barkhof F, Leys D, Pruvo JP, Nauta JJ, Vermersch P, Steinling M, Valk J. A semiquantative rating scale for the assessment of signal hyperintensities on magnetic resonance imaging. J Neurol Sci 1993;114:7-12. [Crossref] [PubMed]
- Wardlaw JM, Smith EE, Biessels GJ, Cordonnier C, Fazekas F, Frayne R, et al. Neuroimaging standards for research into small vessel disease and its contribution to ageing and neurodegeneration. Lancet Neurol 2013;12:822-38. [Crossref] [PubMed]
- Ito S, Nagasawa T, Abe M, Mori T. Strain vessel hypothesis: a viewpoint for linkage of albuminuria and cerebro-cardiovascular risk. Hypertens Res 2009;32:115-21. [Crossref] [PubMed]
- Azar S, Tobian L, Johnson MA. Glomerular, efferent arteriolar, peritubular capillary, and tubular pressures in hypertension. Am J Physiol 1974;227:1045-50. [Crossref] [PubMed]
- Greenberg SM. Small vessels, big problems. N Engl J Med 2006;354:1451-3. [Crossref] [PubMed]
- Marano M, Vespasiani Gentilucci U, Altamura C, Siotto M, Squitti R, Bucossi S, Quintiliani L, Migliore S, Greco F, Scarciolla L, Quattrocchi CC, Picardi A, Vernieri F. Altered metal metabolism in patients with HCV-related cirrhosis and hepatic encephalopathy. Metab Brain Dis 2015;30:1445-52. [Crossref] [PubMed]
- Mallio CA, Lo Vullo G, Messina L, Beomonte Zobel B, Parizel PM, Quattrocchi CC. Increased T1 Signal Intensity of the Anterior Pituitary Gland on Unenhanced Magnetic Resonance Images After Chronic Exposure to Gadodiamide. Invest Radiol 2020;55:25-9. [Crossref] [PubMed]
- Lasaponara S, Mauro F, Carducci F, Paoletti P, Tombini M, Quattrocchi CC, Mallio CA, Errante Y, Scarciolla L, Ben-Soussan TD. Increased Alpha Band Functional Connectivity Following the Quadrato Motor Training: A Longitudinal Study. Front Hum Neurosci 2017;11:282. [Crossref] [PubMed]
- Wartolowska KA, Webb AJS. Blood Pressure Determinants of Cerebral White Matter Hyperintensities and Microstructural Injury: UK Biobank Cohort Study. Hypertension 2021;78:532-9. [Crossref] [PubMed]
- Tamura Y, Araki A. Diabetes mellitus and white matter hyperintensity. Geriatr Gerontol Int 2015;15:34-42. [Crossref] [PubMed]
- Power MC, Deal JA, Sharrett AR, Jack CR Jr, Knopman D, Mosley TH, Gottesman RF. Smoking and white matter hyperintensity progression: the ARIC-MRI Study. Neurology 2015;84:841-8. [Crossref] [PubMed]
- Naganuma T, Takemoto Y. New aspects of cerebrovascular diseases in dialysis patients. Contrib Nephrol 2015;185:138-46. [Crossref] [PubMed]