Left ventricular strain in patients with Takayasu arteritis with preserved ejection fraction: an analysis using cardiac magnetic resonance imaging feature tracking
Introduction
Takayasu arteritis (TAK), a chronic granulomatous arteritis which mainly affects the aorta and its major branches, sometimes involves the pulmonary artery. The major cause of death in patients with TAK is cardiovascular disease, especially heart failure (1). The most common causes include ischemic coronary artery disease, aortic regurgitation, hypertension (HTN), and pulmonary hypertension (PH) (2-4). The inflammatory etiology is concerned for left ventricular (LV) dysfunction in patients with TAK (5), and endomyocardial biopsy indicates a high incidence of subclinical myocarditis in patients with TAK (6,7). However, myocardial abnormalities in patients with TAK have received little attention, especially in patients with normal left ventricular ejection fraction (LVEF) (8,9). Zhang et al. reported that myocardial deformation impairment was present in the majority of patients with TAK (10); however, whether the decline of regional myocardial deformation occurs in patients with TAK and preserved LVEF (pLVEF) as well as the risk factors for impairment of LV strain and their prognostic value remains unclear.
The cardiac magnetic resonance imaging feature tracking (CMR-FT) technique provides useful information to quantitatively evaluate subclinical cardiac dysfunction in many diseases (11). Myocardial strain and strain rate have been measured by conventional cine images with excellent intra- and interobserver agreement and high interstudy reproducibility (12,13). However, subtle alterations of LV function evaluated with CMR-FT have not been reported in patients with TAK.
Thus, the aims of this study were the following: (I) to explore the global and regional strain abnormalities of LV using CMR-FT in patients with TAK and pLVEF, (II) to analyze the risk factors for the altered LV strain in patients with TAK, (III) and to use transthoracic echocardiography (TTE) as a reference during the 6-month follow up to compare the baseline deference of LV strain between patients with TAK with reduced and nonreduced LVEF. We present the following article in accordance with the STROBE reporting checklist (available at https://qims.amegroups.com/article/view/10.21037/qims-22-82/rc).
Methods
Study population
The study was conducted in accordance with the Declaration of Helsinki (as revised in 2013). This study was approved by our hospital ethics committee (approval No. 2017-K-127), and all participants provided signed informed consent. Sixty patients who were diagnosed with TAK and underwent CMR in our hospital between October 2017 and December 2019 were prospectively enrolled in this case-control study. Inclusion criteria were the diagnostic criteria of the modified Ishikawa’s diagnostic criteria for TAK by Sharma et al. (14). Exclusion criteria were the following: (I) poor image quality of CMR; (II) a baseline LVEF on CMR less than 50%; or (III) proven or suspected ongoing infection, congenital heart disease, or malignant tumor. A detailed flow diagram of eligible patients is shown in Figure 1. The 1994 International TAK Conference in Tokyo angiographic classification criteria were used to classify TAK types (15). Patients were divided into 2 groups, the active and inactive patient groups, based on TAK activity criteria at the time of the CMR scan according to the National Institutes of Health (NIH) (16). Erythrocyte sedimentation rate (ESR) was measured using the Westergren method (17) at first admission. Other clinical data, including gender, age, disease duration, disease activity, TAK classification, and drug treatment, were collected. Thirty age- and gender-matched health volunteers were included as controls.
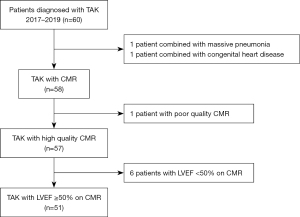
CMR imaging
All participants underwent CMR using a 3.0-T scanner (Prisma, Siemens Healthcare, Erlangen, Germany) with a body-flex receiver coil following an electrocardiogram (ECG) examination and employing the breath-hold technique. After scout imaging, 8–12 continuous CMR cine images with a true fast imaging with steady-state precession sequence were acquired based on the short-axis plane to cover the whole ventricle [repetition time (TR) 3.0–3.2 ms, echo time (TE) 1.4 ms, flip angle 70°, field of view 320 mm × 360 mm, matrix size 256 mm × 256 mm, slice thickness 8 mm, phases per cardiac cycle 25]. In addition, images of 2-, 3-, and 4-chamber views were acquired based on the LV long-axis plane.
Late gadolinium enhancement (LGE) was scanned with a phase-sensitive inversion recovery sequence under the following parameters: TE 1.94 ms, TR 684 ms, flip angle 20°, and spatial resolution 1.4 mm × 1.4 mm × 8 mm. A stack of images was obtained with the same slice position as cine imaging after 15 minutes of intravenous administration of gadopentetate dimeglumine (Bayer, Leverkusen, Germany) with a dose of 0.1 mmol/kg body weight. The injection was performed at a rate of 3 mL/s and was followed by a 20-mL saline flush at a rate of 2 mL/s.
CMR data analysis
Image analysis was performed offline by 2 observers with 5 and 10 years’ experience using the commercial software CVI42 (version 5.11, Circle Cardiovascular Imaging Inc., Calgary, AB, Canada). Endocardial and epicardial traces were processed automatically based on the serial short-axis plane images at the end-diastolic and end-systolic phases. The majority results from the automatic software analysis were used, which were derived from excluding the papillary muscles and moderator bands. LV functional metrics, including end-diastolic volume (EDV), end-systolic volume (ESV), and LVEF, were calculated using the cardiac function analysis model, and then myocardial strain was analyzed using the tissue-tracking model by importing all short-axis and long 4-chamber plane images. Global and regional (apical, midventricular, and basal) feature-tracking parameters were performed automatically, including myocardial longitudinal peak strain (LPS), circumferential peak strain (CPS), and radial peak strain (RPS), systolic peak strain rate (SPSR), and diastolic peak strain rate (DPSR). Circumferential strain (CS) and radial strain (RS) were analyzed in short-axis images, and longitudinal strain (LS) analysis used long-axis 4-chamber images. Peak strain (PS) was defined as the maximum absolute value of the strain in the entire cardiac cycle; SPSR was defined as the maximum absolute value of the systolic strain rate in 1 cardiac cycle; DPSR was defined as the maximum absolute value of the diastolic strain rate in 1 cardiac cycle.
LGE was assessed by XJ Guo, who has 10 years’ experience in imaging diagnosis of cardiovascular diseases and who was blinded to all clinical information. The expert provided a visual judgement, including positive LGE vs. negative LGE, position, and LGE patterns. When the high signal in the myocardium was detected in 2 orthogonal planes, it was defined as positive LGE.
Reproducibility
Interobserver variability was evaluated by 2 independent double-blinded observers based on a comparison of measurements of myocardial deformation parameters of 20 randomly selected cases.
Follow-up
Patients were followed up after 6 months using TTE performed at our hospital as a reference. During this period, they received the appropriate treatment as recommended by the clinician. Based on the baseline and follow-up TTE, patients were grouped as either those with reduced LVEF or those with nonreduced LVEF.
Statistics analysis
All statistical data were analyzed using SPSS 19.0 software (IBM Corp., Armonk, NY, USA). Quantitative data are expressed as mean ± standard deviation or median with a range based on the distribution of data. Values for qualitative variables are expressed as a percentage. The Shapiro-Wilk test was performed to determine normality. An unpaired Student t-test or Mann-Whitney test was used to compare myocardial deformation parameters between patients with TAK and normal controls. The receiver operating curve (ROC) analysis was performed to assess the suitable diagnostic thresholds for the area under the curve (AUC) for deformation parameters. Patients with TAK were divided into 3 groups based on comorbidities, and a 1-way analysis of variance (ANOVA) was used to determine subgroup differences and followed by multiple comparisons using the least significant difference t-test. Pearson r or Spearman rho correlation analysis, as appropriate, was performed to assess the correlations between strain parameters and clinical indicators. Bland-Altman plots were used to assess reproducibility. A 2-tailed P value <0.05 was considered to indicate a statistically significant difference.
Results
Patient characteristics
Fifty-one patients with TAK were enrolled in this study. Table 1 shows the baseline clinical and CMR data of all patients with TAK and normal controls. Ultimately, 30 patients were followed up after 6 months with TTE, while the other 21 patients were lost to follow-up. Moreover, the baseline LVEF measured with TTE at admission was significantly larger than that measured with CMR (0.68±0.06 and 0.62±0.06, respectively; t=4.897; P<0.001), and values were positively correlated (r=0.626; P<0.001).
Table 1
Variable | Patients with TAK | Normal controls (n=30) | P |
---|---|---|---|
Clinical characteristics (n=51) | |||
Female | 33 (64.71) | 13 (43.33) | 0.06 |
Age (years) | 38±12 | 42±16 | 0.05 |
BMI (kg/m2) | 22.88±3.60 | 23.48±3.05 | 0.45 |
Heart rate (beats/min) | 69±12 | 72±13 | 0.29 |
Disease duration (months) | 11 [1–432] | ||
Active disease at admission time (NIH criteria) | 28 (54.90) | ||
Cardiovascular complications (n=51) | |||
HTN | 10 (19.60) | 3 (10.00) | <0.001 |
PH | 10 (19.60) | 0 | |
Aortic regurgitation | 6 (11.74) | ||
HTN and PH | 4 (7.84) | 0 | |
TAK type (n=51) | |||
Pulmonary artery type | 35 (68.62) | ||
Other type with pulmonary artery involvement | 16 (31.38) | ||
IIb | 7 (13.72) | ||
IIa | 4 (7.85) | ||
III | 1 (1.96) | ||
IV | 1 (1.96) | ||
V | 1 (1.96) | ||
Other type without pulmonary artery involvement | |||
I | 2 (3.93) | ||
Treatment at admission time (n=51) | |||
Steroid therapy | 14 (27.45) | ||
Steroid and immunosuppressive therapy | 11 (21.57) | ||
ESR (mm/h) | 10 [2–96] | ||
CMR (n=51) | |||
LVEF (%) | 0.59±0.10 | 0.64±0.05 | 0.39 |
LVEDV (mL) | 117.59±20.71 | 124.25±30.47 | 0.55 |
LVESV (mL) | 48.14±19.01 | 47.64±11.37 | 0.82 |
Cardiac output (L/min) | 4.94±1.68 | 5.10±1.73 | 0.94 |
Diastolic myocadiac mass (g) | 74.91±22.40 | 85.32±32.37 | <0.05 |
LGE pattern on CMR | 24 (47.05) | ||
1 | 16 (32.37) | ||
2 | 6 (11.76) | ||
3 | 1 (1.96) | ||
4 | 1 (1.96) | ||
LVEF change at 6-month follow-up using TTE (n=30, EF decline ≥5% as reduced LVEF) | |||
LVEF in admission (%) | 0.68±0.06 | ||
LVEF in a follow-up (%) | 0.65 (0.35–0.76) | ||
Reduced LVEF (%) (n=10) | 0.72 (0.46–0.83) | ||
Nonreduced LVEF (%) (n=20) | 0.69 (0.60–0.72) |
Data with skewed distribution are represented by median, maximum, and minimum values, others presented as number (percentage) or mean ± standard deviation. 1, the pattern of LGE located on ventricular insert point; 2, the pattern of LGE located on ventricular insert point and septum; 3, the pattern of LGE located on ventricular insert point and septum, right ventricular free wall; 4, the pattern of LGE located on ventricular insert point and septum, left ventricular. TAK, Takayasu arteritis; BMI, body mass index; NIH, National Institutes of Health; HTN, hypertension; PH, pulmonary hypertension; ESR, erythrocyte sedimentation rate; CMR, cardiac magnetic resonance imaging; LV, left ventricular; EF, ejection fraction; EDV, end-diastolic volume; ESV, end-systolic volume; LGE, late gadolinium enhancement; TTE, transthoracic echocardiography.
Table S1 shows the clinical information of patients with TAK who were followed up and lost to follow-up. There were no significant differences in age (t=–1.46; P=0.15); gender (χ2=0.06; P=0.81); disease duration (Z=–1.81; P=0.07); disease status (χ2=0.77; P=0.38); or cardiovascular complications of PH, HTN (χ2=0.005, P=0.943; P>0.99), and ESR (Z=–0.71; P=0.48) between the 2 groups. Although the BMI of the patients who were followed up was lower than that of those who were lost to follow-up, baseline LV function parameters (EDV index: t=1.25, P=0.217; ESV index: t=–0.47, P=0.640) and diastolic myocadiac mass index (t=0.983; P=0.331) between the 2 groups were similar. Furthermore, the baseline LVEF based on TTE (t=0.62; P=0.53) and LVEF based on CMR (t=–1.62; P=0.11) between the 2 groups were comparable. LGEs based on CMR for the 2 groups were also similar (χ2=5.28; P=0.26).
LV global and regional strain metrics in patients and heathy controls
Table 2 indicates that LV global longitudinal PS (GLS: t=2.36; P=0.021) and global circumferential PS (GCS: t=2.25; P=0.027) in patients with TAK were significantly lower than those in controls. Moreover, GLS had an AUC of 0.64 (95% CI: 0.52–0.76) for distinguishing patients with TAK and pLVEF from healthy controls. The best diagnostic threshold was –14.74% with a sensitivity of 66.70% and a specificity of 50.00%. GCS had an AUC of 0.63 (95% CI: 0.51–0.76), showing a sensitivity of 66.70% and a specificity of 56.70% (Figure 2), corresponding to a diagnostic threshold of –22.38%.
Table 2
Global strain metrics | Normal controls (n=30) | Patients with TAK with pLVEF (n=51) |
---|---|---|
Longitudinal | ||
PS (%) | −14.77±1.74 | −13.35±3.11* |
SPSR (1/s) | −0.71±0.45 | −0.81±0.19 |
DPSR (1/s) | 0.94±0.24 | 0.86±0.38 |
Circumferential | ||
PS (%) | −22.75±2.57 | −21.46±2.66* |
SPSR (1/s) | −1.13±0.18 | −1.12±0.20 |
DPSR (1/s) | 1.41±0.35 | 1.34±0.36 |
Radial | ||
PS (%) | 40.70±8.84 | 38.24±8.64 |
SPSR (1/s) | 2.32±0.58 | 2.15±0.61 |
DPSR (1/s) | −2.92±0.98 | −2.51±1.03 |
The values are the mean ± SD. *, P<0.05. TAK, Takayasu arteritis; pLVEF, preserved left ventricular ejection fraction; PS, peak strain; SPSR, systolic peak strain rate; DPSR, diastolic peak strain rate.
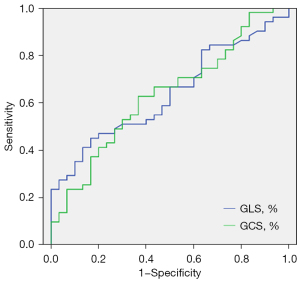
As is shown in Table 3, the LPS apical (t=−3.11; P=0.003) and midventricular regions (t=−2.25; P=0.027) in the TAK group decreased in comparison with controls, and the CPS in basal (t=–2.36; P=0.021) and midventricular regions (t=−2.71; P=0.008) were significantly lower in the TAK group compared with normal controls.
Table 3
Variable | Basal | Midventricular | Apical | |||||
---|---|---|---|---|---|---|---|---|
Normal controls (n=30) | Patients with TAK and pLVEF (n=51) | Normal controls (n=30) | Patients with TAK and pLVEF (n=51) | Normal controls (n=30) | Patients with TAK and pLVEF (n=51) | |||
Longitudinal | ||||||||
PS (%) | −12.05±2.99 | −10.66±3.53 | −14.20±1.98 | −12.34±3.43* | −17.82±1.91 | −16.45±3.71* | ||
SPSR (1/s) | −0.58±0.42 | −0.67±0.40 | −0.78±0.37 | −0.82±0.25 | −0.85±0.43 | −0.92±0.17 | ||
DPSR (1/s) | 0.88±0.31 | 0.85±0.39 | 0.95±0.24 | 0.87±0.45 | 1.11±0.25 | 1.06±0.37 | ||
Circumferential | ||||||||
PS (%) | −19.25±1.88 | −17.07±3.45* | −23.27±2.88 | −21.50±3.07* | −26.86±3.64 | −26.17±4.05 | ||
SPSR (1/s) | −1.02±0.14 | −0.94±0.22 | −1.18±0.21 | 1.04±0.21 | −1.44±0.25 | −1.37±0.32 | ||
DPSR (1/s) | 1.27±0.30 | 1.11±0.29* | 1.48±0.40 | 1.19±0.38 | 1.84±0.59 | 1.75±0.60 | ||
Radial | ||||||||
PS (%) | 47.18±10.07 | 43.86±12.24 | 37.35±8.06 | 35.61±9.41 | 43.22±13.79 | 40.45±13.74 | ||
SPSR (1/s) | 2.17±0.54 | 2.14±0.66 | 2.02±0.51 | 2.03±0.69 | 3.24±1.51 | 2.72±1.06 | ||
DPSR (1/s) | −3.61±0.94 | −3.47±1.38 | −2.67±0.86 | −2.58±0.87 | −3.24±2.48 | −3.22±1.34 |
Values presented are mean ± SD. *, P<0.05. TAK, Takayasu arteritis; pLVEF, preserved left ventricular ejection fraction; PS, peak strain; SPSR, systolic peak strain rate; DPSR, diastolic peak strain rate.
Based on the subgroup analysis shown in Table 4, LV global strain and strain rate of patients with TAK showed significant differences among patients without comorbidities, patients with HTN, and patients with PH, in addition to global longitudinal SPSR. According to 1-way ANOVA of the comorbidities, there was a significant difference between the PH group and the no-comorbidities group for GLS (t=−2.47; P=0.01; Figure 3A). Statistically significant differences were observed between the HTN and PH group (t=−3.11; P=0.007) and the PH and no-comorbidities groups for GCS (t=−3.44; P<0.001; Figures 3B,4,5). Furthermore, longitudinal and circumferential strain parameters were decreased in patients with TAK and LGE compared with patients without LGE (Figure 6). The differences in strain between the groups was greatest for the following values: basal LPS (t=−2.62; P=0.011) and CPS (t=−2.05; P=0.04), midventricular LPS (Z=−2.60; P=0.009) and CPS (t=−2.78; P=0.008), apical LPS (t=−3.43; P=0.001) and CPS (t=−2.32; P=0.025), and GLS (t=−3.30; P=0.002) and GCS (t=−3.02; P=0.004).
Table 4
Subgroup in patients with TAK | Longitudinal (%) | Circumferential (%) | Radial (%) | Longitudinal (1/s) | Circumferential (1/s) | Radial (1/s) | ||||||||
---|---|---|---|---|---|---|---|---|---|---|---|---|---|---|
PS | PS | PS | SPSR | DPSR | SPSR | DPSR | SPSR | DPSR | ||||||
No-comorbidities group (n=27) | −14.27±2.16 | −22.35±2.06 | 40.06±8.15 | −0.80±0.05 | 0.98±0.05 | −1.19±0.03 | 1.43±0.28 | 2.35±0.10 | −2.86±0.13 | |||||
HTN group (n=10) | −14.06±1.20 | −22.86±2.63 | 38.31±2.52 | −0.78±0.08 | 0.58±0.13 | −1.06±0.06 | 1.15±0.23 | 2.09±0.16 | −2.13±0.43 | |||||
PH group (n=10) | −11.81±1.07 | −19.14±1.01 | 31.54±2.63 | −0.66±0.05 | 0.62±0.17 | −0.99±0.05 | 1.16±0.10 | 1.70±0.14 | −2.08±0.26 | |||||
F | 3.55 | 7.75 | 4.407 | 1.22 | 7.04 | 6.16 | 7.29 | 5.47 | 4.50 | |||||
P | 0.035 | 0.001 | 0.016 | 0.302 | 0.002 | 0.004 | 0.001 | 0.006 | 0.015 |
The values are the mean ± SD. Four patients with PH and HTN were excluded in subgroup analysis. LV, left ventricular; TAK, Takayasu arteritis; HTN, hypertension; PH, pulmonary hypertension; PS, peak strain; SPSR, systolic peak strain rate; DPSR, diastolic peak strain rate.
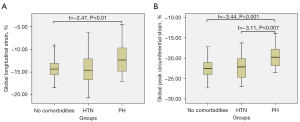
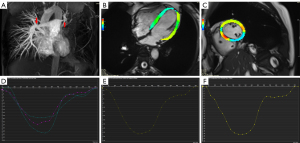
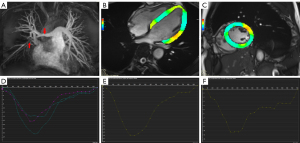
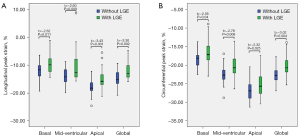
Moreover, interobserver agreement showed good reproducibility based on the Bland-Altman plots (Figure 7).
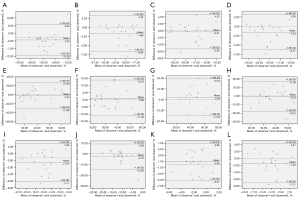
Correlation of LV strain and clinical data in patients with TAK
As shown in Figure 8, both GLS and GCS were significantly different between male and female patients. GCS showed a significant positive association with disease duration (r=0.37; P=0.008), and GLS showed a significant negative association with ESR (r=–0.30; P=0.03). There were no statistically significant associations between GLS and GCS with age, disease activity, TAK classification, or drug treatment in patients with TAK (all P values >0.05).
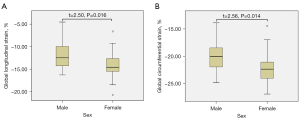
Relationship between baseline myocardial strain and reduced LVEF at follow-up
In total, 30 patients were followed up with TTE after 6 months. Among them, 21 (70%) showed a decline in LVEF (range, 1–12%); 1 patient exhibited no change of LVEF, and 8 patients showed increased LVEF (range, 1–7%). According to a comparison to the baseline LVEF, 33% (10/30) patients with a decline in LVEF ≥5% during follow-up were classified into the group with reduced LVEF. The baseline LV EDV index (50.21±9.69 mL/m2) was larger in patients with reduced LVEF compared with those (43.84±9.37 mL/m2) with nonreduced LVEF, this difference was not statistically significant (t=–1.74; P=0.093).
The baseline global circumferential DPSR {TAK with reduced LVEF [1.45±0.39 (1/s)] vs. TAK without reduced LVEF [1.18±0.25 (1/s)]; Z=–2.25; P=0.03} and the baseline apical circumferential DPSR {TAK with reduced LVEF [2.20±0.80 (1/s)] vs. TAK without reduced LVEF [1.61±0.39 (1/s)]; Z=–2.74; P=0.01} were significantly different. The baseline global and apical circumferential DPSR in patients with reduced LVEF were higher than those in patients without a reduced LVEF at the 6-month follow-up (Figure 9).
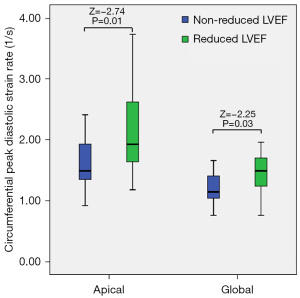
Discussion
In the current study, we first analyzed impaired LV strain and risk factors in patients with TAK and pLVEF using CMR-FT, and there were several findings: (I) reduced global and segmental LPS and CPS were found in patients with TAK and pLVEF; (II) patients with PH had a decreased LV strain compared with patients with HTN; (III) patients with LGE had significant reduction of LV strain metrics compared with those without LGE; and (IV) male gender, long disease duration, and elevated ESR were risk factors of reduced GLS and GCS. (V) LVEF during the follow-up might decrease in patients who have elevated circumferential DPSR at baseline.
Myocardial involvement is one of the important causes of a long-term poor outcomes in patients with TAK (18). Although the possible underlying mechanism of LV dysfunction in TAK is not completely clarified, identification of the subtle changes of myocardial strain in patients with TAK is important. Grotenhuis et al. reported that pediatric patients with TAK demonstrated altered LV mechanics, LV hypertrophy, and impaired diastolic function (19). Moreover, Yurdakul et al. used velocity vector imaging and reported that LV longitudinal peak systolic strain and strain rate were reduced in patients with TAK (20).
CMR can simultaneously visualize the morphology, function, flow, and tissue characteristics of the heart. CMR-FT studies have been widely used to measure altered myocardial strain in cardiovascular diseases (21-23). Our study found that GLS and GCS decreased in patients with TAK and pLVEF in comparison with healthy volunteers. In contrast, Yurdakul et al. reported that LV longitudinal peak systolic strain and strain rate were reduced in patients with TAK (20). GLS and GCS showed similar AUCs when discriminating patients with TAK and pLVEF from healthy controls, and both GLS and GCS showed higher sensitivity and lower specificity. This suggests that FT-derived strain metrics is a sensitive method to detect subtle myocardial dysfunction in patients with TAK and pLVEF.
Regional strain analysis indicated that the reduction of LPS and CPS was mainly in the midventricular myocardium. One possible reason might be that greater stress is present in the lateral myocardium compared with that in other regions (24). Meanwhile, spiral fibers in the middle layer and longitudinal fibers in the subendocardium determine myocardial circumferential and longitudinal deformation stress, respectively (22).
Our study suggests that both GLS and GCS in patients with PH was more reduced than that in controls and patients with HTN. Further analysis showed that almost all LV strain and strain rate metrics decreased in patients with PH. The main reason for this might be that ventricular interdependence in PH patients aggravates abnormal LV function (25). Keenan et al. suggested that inflammatory involvement of myocardium in patients with TAK can be confirmed by mid-wall fibrosis derived from CMR, which results in the reduction of myocardial deformation (5). Our results indicate that patients with LGE have significantly decreased myocardial strain metrics.
ESR is one of the best predictors for long-term follow-up results of patients with TAK, and preclinical reduction of GLS was found to be independently related to a worse clinical prognosis (26). The negative correlation between ESR and GLS enhances the prognostic value of both in patients with TAK. A possible explanation is that ESR accompanying arteritis and myocardial inflammation reduces the deformation of LV. This is consistent with Eleftheriou’s findings (27). In our study, LV strain in female patients was slightly reduced compared to that of male patients. Previous studies have reported that females are better able to maintain normal EF due to a greater magnitude of deformation in females compared with males in a healthy population (28,29). A long disease duration often leads to ventricle remodeling characterized by myocardial fibrosis (9), which in turn affects myocardia distortion, especially CPS.
A decrease in LVEF ≥10% was used as a threshold to indicate reduced LVEF. However, only 2 patients had reduced LVEF ≥10%, and the small number of cases prevented further analysis. When we used a decrease of ≥5% according to Seidman et al. (30) as the threshold, 33% patients with TAK showed reductions in LVEF during the follow-up. Thus, we used the decline in LVEF of at least 5% to group patients with reduced EF and patients with nonreduced EF at the 6-month follow-up. These patients had higher baseline circumferential DPSR compared with those with nonreduced LVEF during the follow-up. The population differences among our young patients and older obese patients with LV diastolic dysfunction (31) and pediatric patients with TAK (19) make explaining the possible mechanisms difficult. Nonetheless, we speculate that the possible reasons may be following: (I) the increase in preload due to ventricular dilatation led to an increase in LV diastolic strain rate (32), for the baseline LV EDV index in our study was larger in patients with reduced LVEF than that in patients with nonreduced LVEF; and (II) there was an early adaptive mechanism in response to increased LV afterload due to the large artery stiffness of TAK (19) in patients with reduced LVEF. However, the details of mechanism remain unknown.
Limitations
Our study has several limitations. (I) This was a single-center study with a relatively small number of patients with TAK. (II) Although we did not find significant differences of baseline variables between the follow-up and lost-to-follow-up groups, the small number of the follow-up patients might have led to selection bias. Future research should include long-term follow-up data involving larger sample sizes. (III) Most of our participants received different immunosuppressive drugs; however, the effect of the treatments was not evaluated. (IV) There are other possible important confounders such as aortic regurgitation, which have not been assessed thus far. (V) Myocardial strain analysis has excellent reproducibility, and thus only interobserver agreement was assessed. (VI) We used a decline in LVEF of at least 5% as the threshold of the reduced LVEF. This might have affected the accuracy of patient categorization and led to an increased probability of type II statistical errors. Future work should seek to confirm and extend our findings in larger patient samples.
Conclusions
In patients with TAK with pLVEF, both global and segmental myocardial strain decreased. Patients with TAK and PH have greater reduction of LV strain than do those without PH. Male gender, long disease duration, elevated ESR, and myocardial LGE are associated with reduction of LV strain. A baseline increase of circumferential DPSR may be associated with the decline in LVEF during the follow-up. CMR-FT assists with the detection of subtle alterations of myocardial strain in patients with TAK and pLVEF. Identification of early myocardial abnormal deformation and risk factors will assist physicians with optimizing the management of patients with TAK.
Acknowledgments
We thank Dr. Yi Ren (Capital Medical University, Beijing, China) and Dr. Zhanhong Ma (Department of Radiology, Beijing Chaoyang Hospital, Capital Medical University, Beijing, China) for their invaluable support of our study.
Funding: This work was supported by Chinese Academy of Medical Sciences Innovation Fund for Medical Sciences (No. 2021-I2M-1-049), the National High Level Hospital Clinical Research Funding and the Elite Medical Professionals Project of China-Japan Friendship Hospital (No. 2022-NHLHCRF-LX-01 and No. ZRJY2021-BJ02), and the National Natural Science Foundation of China (No. 81871328).
Footnote
Reporting Checklist: The authors have completed the STROBE reporting checklist. Available at https://qims.amegroups.com/article/view/10.21037/qims-22-82/rc
Conflicts of Interest: All authors have completed the ICMJE uniform disclosure form (available at https://qims.amegroups.com/article/view/10.21037/qims-22-82/coif). The authors have no conflicts of interest to declare.
Ethical Statement: The authors are accountable for all aspects of the work in ensuring that questions related to the accuracy or integrity of any part of the work are appropriately investigated and resolved. The study was conducted in accordance with the Declaration of Helsinki (as revised in 2013). This study protocol was approved by our Hospital Ethics Committee (approval No. 2017-K-127), and informed consent was obtained from all individual participants included in the study.
Open Access Statement: This is an Open Access article distributed in accordance with the Creative Commons Attribution-NonCommercial-NoDerivs 4.0 International License (CC BY-NC-ND 4.0), which permits the non-commercial replication and distribution of the article with the strict proviso that no changes or edits are made and the original work is properly cited (including links to both the formal publication through the relevant DOI and the license). See: https://creativecommons.org/licenses/by-nc-nd/4.0/.
References
- Li J, Sun F, Chen Z, Yang Y, Zhao J, Li M, Tian X, Zeng X. The clinical characteristics of Chinese Takayasu's arteritis patients: a retrospective study of 411 patients over 24 years. Arthritis Res Ther 2017;19:107. [Crossref] [PubMed]
- Espígol-Frigolé G, Prieto-González S, Alba MA, Tavera-Bahillo I, García-Martínez A, Gilabert R, Hernández-Rodríguez J, Cid MC. Advances in the diagnosis of large vessel vasculitis. Rheum Dis Clin North Am 2015;41:125-40. ix. [Crossref] [PubMed]
- Lee GY, Jang SY, Ko SM, Kim EK, Lee SH, Han H, Choi SH, Kim YW, Choe YH, Kim DK. Cardiovascular manifestations of Takayasu arteritis and their relationship to the disease activity: analysis of 204 Korean patients at a single center. Int J Cardiol 2012;159:14-20. [Crossref] [PubMed]
- Gong J, Yang Y, Ma Z, Guo X, Wang J, Kuang T, Yang S, Li J, Miao R, Huang K. Clinical and imaging manifestations of Takayasu's arteritis with pulmonary hypertension: A retrospective cohort study in China. Int J Cardiol 2019;276:224-9. [Crossref] [PubMed]
- Keenan NG, Mason JC, Maceira A, Assomull R, O'Hanlon R, Chan C, Roughton M, Andrews J, Gatehouse PD, Firmin DN, Pennell DJ. Integrated cardiac and vascular assessment in Takayasu arteritis by cardiovascular magnetic resonance. Arthritis Rheum 2009;60:3501-9. [Crossref] [PubMed]
- Takeda N, Takahashi T, Seko Y, Maemura K, Nakasone H, Sakamoto K, Hirata Y, Nagai R. Takayasu myocarditis mediated by cytotoxic T lymphocytes. Intern Med 2005;44:256-60. [Crossref] [PubMed]
- Seko Y, Minota S, Kawasaki A, Shinkai Y, Maeda K, Yagita H, Okumura K, Sato O, Takagi A, Tada Y. Perforin-secreting killer cell infiltration and expression of a 65-kD heat-shock protein in aortic tissue of patients with Takayasu's arteritis. J Clin Invest 1994;93:750-8. [Crossref] [PubMed]
- Bechman K, Gopalan D, Nihoyannopoulos P, Mason JC. A cohort study reveals myocarditis to be a rare and life-threatening presentation of large vessel vasculitis. Semin Arthritis Rheum 2017;47:241-6. [Crossref] [PubMed]
- Comarmond C, Cluzel P, Toledano D, Costedoat-Chalumeau N, Isnard R, Gaudric J, Chiche L, Koskas F, Cacoub P, Saadoun D. Findings of cardiac magnetic resonance imaging in asymptomatic myocardial ischemic disease in Takayasu arteritis. Am J Cardiol 2014;113:881-7. [Crossref] [PubMed]
- Zhang H, Zhao L, Zhang C, Tian J, Ding Y, Zhao X, Ma X. Quantification of Myocardial Deformation in Patients with Takayasu Arteritis by Cardiovascular Magnetic Resonance Feature Tracking Imaging. J Magn Reson Imaging 2022;55:1828-40. [Crossref] [PubMed]
- Smiseth OA, Torp H, Opdahl A, Haugaa KH, Urheim S. Myocardial strain imaging: how useful is it in clinical decision making? Eur Heart J 2016;37:1196-207. [Crossref] [PubMed]
- Kowallick JT, Morton G, Lamata P, Jogiya R, Kutty S, Lotz J, Hasenfuß G, Nagel E, Chiribiri A, Schuster A. Inter-study reproducibility of left ventricular torsion and torsion rate quantification using MR myocardial feature tracking. J Magn Reson Imaging 2016;43:128-37. [Crossref] [PubMed]
- Morton G, Schuster A, Jogiya R, Kutty S, Beerbaum P, Nagel E. Inter-study reproducibility of cardiovascular magnetic resonance myocardial feature tracking. J Cardiovasc Magn Reson 2012;14:43. [Crossref] [PubMed]
- Sharma BK, Jain S, Suri S, Numano F. Diagnostic criteria for Takayasu arteritis. Int J Cardiol 1996;54:S141-7. [Crossref] [PubMed]
- Hata A, Noda M, Moriwaki R, Numano F. Angiographic findings of Takayasu arteritis: new classification. Int J Cardiol 1996;54:S155-63. [Crossref] [PubMed]
- Kerr GS, Hallahan CW, Giordano J, Leavitt RY, Fauci AS, Rottem M, Hoffman GS. Takayasu arteritis. Ann Intern Med 1994;120:919-29. [Crossref] [PubMed]
- Happe MR, Battafarano DF, Dooley DP, Rennie TA, Murphy FT, Casey TJ, Ward JA. Validation of the Diesse Mini-Ves erythrocyte sedimentation rate (ESR) analyzer using the Westergren ESR method in patients with systemic inflammatory conditions. Am J Clin Pathol 2002;118:14-7. [Crossref] [PubMed]
- Ishikawa K, Maetani S. Long-term outcome for 120 Japanese patients with Takayasu's disease. Clinical and statistical analyses of related prognostic factors. Circulation 1994;90:1855-60. [Crossref] [PubMed]
- Grotenhuis HB, Aeschlimann FA, Hui W, Slorach C, Yeung RSM, Benseler SM, Bradley TJ, Grosse-Wortmann L. Increased Arterial Stiffness Adversely Affects Left Ventricular Mechanics in Patients With Pediatric Takayasu Arteritis From a Toronto Cohort. J Clin Rheumatol 2019;25:171-5. [Crossref] [PubMed]
- Yurdakul S, Alibaz-Öner F, Direskeneli H, Aytekin S. Impaired cardiac and vascular motion in patients with Takayasu's arteritis: A velocity vector imaging-based study. Eur J Rheumatol 2018;5:16-21. [Crossref] [PubMed]
- Kalam K, Otahal P, Marwick TH. Prognostic implications of global LV dysfunction: a systematic review and meta-analysis of global longitudinal strain and ejection fraction. Heart 2014;100:1673-80. [Crossref] [PubMed]
- DeVore AD, McNulty S, Alenezi F, Ersboll M, Vader JM, Oh JK, Lin G, Redfield MM, Lewis G, Semigran MJ, Anstrom KJ, Hernandez AF, Velazquez EJ. Impaired left ventricular global longitudinal strain in patients with heart failure with preserved ejection fraction: insights from the RELAX trial. Eur J Heart Fail 2017;19:893-900. [Crossref] [PubMed]
- Yao Q, Hu XH, He LL. Evaluation of comprehensive myocardial contractility in children with Kawasaki disease by cardiac magnetic resonance in a large single center. Quant Imaging Med Surg 2022;12:481-92. [Crossref] [PubMed]
- Cho YH, Kang JW, Choi SH, Yang DH, Anh TTX, Shin ES, Kim YH. Reference parameters for left ventricular wall thickness, thickening, and motion in stress myocardial perfusion CT: Global and regional assessment. Clin Imaging 2019;56:81-7. [Crossref] [PubMed]
- Kallianos K, Brooks GC, Mukai K, Seguro de Carvalho F, Liu J, Naeger DM, De Marco T, Ordovas KG. Cardiac Magnetic Resonance Evaluation of Left Ventricular Myocardial Strain in Pulmonary Hypertension. Acad Radiol 2018;25:129-35. [Crossref] [PubMed]
- Wang Y, Yang H, Nolan M, Pathan F, Negishi K, Marwick TH. Variations in subclinical left ventricular dysfunction, functional capacity, and clinical outcomes in different heart failure aetiologies. ESC Heart Fail 2018;5:343-54. [Crossref] [PubMed]
- Eleftheriou D, Varnier G, Dolezalova P, McMahon AM, Al-Obaidi M, Brogan PA. Takayasu arteritis in childhood: retrospective experience from a tertiary referral centre in the United Kingdom. Arthritis Res Ther 2015;17:36. [Crossref] [PubMed]
- Taylor RJ, Moody WE, Umar F, Edwards NC, Taylor TJ, Stegemann B, Townend JN, Hor KN, Steeds RP, Mazur W, Leyva F. Myocardial strain measurement with feature-tracking cardiovascular magnetic resonance: normal values. Eur Heart J Cardiovasc Imaging 2015;16:871-81. [Crossref] [PubMed]
- Qu YY, Paul J, Li H, Ma GS, Buckert D, Rasche V. Left ventricular myocardial strain quantification with two- and three-dimensional cardiovascular magnetic resonance based tissue tracking. Quant Imaging Med Surg 2021;11:1421-36. [Crossref] [PubMed]
- Seidman A, Hudis C, Pierri MK, Shak S, Paton V, Ashby M, Murphy M, Stewart SJ, Keefe D. Cardiac dysfunction in the trastuzumab clinical trials experience. J Clin Oncol 2002;20:1215-21. [Crossref] [PubMed]
- Samuel TJ, Kitzman DW, Haykowsky MJ, Upadhya B, Brubaker P, Nelson MB, Hundley WG, Nelson MD. Left ventricular diastolic dysfunction and exercise intolerance in obese heart failure with preserved ejection fraction. Am J Physiol Heart Circ Physiol 2021;320:H1535-42. [Crossref] [PubMed]
- Fredholm M, Jörgensen K, Houltz E, Ricksten SE. Load-dependence of myocardial deformation variables - a clinical strain-echocardiographic study. Acta Anaesthesiol Scand 2017;61:1155-65. [Crossref] [PubMed]