Left ventricular myocardial work index and short-term prognosis in patients with light-chain cardiac amyloidosis: a retrospective cohort study
Introduction
Systemic light-chain amyloidosis is a progressive disease characterized by the extracellular deposition of insoluble amyloid fibrils in various organs, causing dysfunction (1). The infiltration in the myocardium could lead to irreversible congestive heart failure in a restrictive pattern (2), with a median survival of 3–6 months (3). For these advanced patients, relatively delayed chemotherapy responses may not improve the outcome (4,5), and the prognosis mainly depends on their cardiac function. Therefore, light-chain cardiac amyloidosis (AL-CA) is a critical subgroup of systemic light-chain amyloidosis. Further examination of AL-CA is required to understand its role in prognostic evaluation, especially the short-term risk of death, to optimize the risk stratification of patients.
Echocardiographically-derived left ventricular longitudinal strain (LVLS) is a useful marker that reflects the amyloid burden (6). However, the load dependency of longitudinal strain (LS) affects the accuracy of evaluating myocardial contractile function (7). The left ventricular myocardial work index (LVMWI) is a novel, noninvasive index that integrates brachial artery cuff blood pressure and LVLS; it overcomes the interference of afterload when assessing myocardial deformation (8).
Initial studies reported that, global LVMWI is associated with the long-term prognosis of patients with cardiac amyloidosis (CA) (9,10). However, these studies did not report on individual types of amyloidosis. Considering the poor prognosis of AL-CA, specific short-term risk stratification may be meaningful to recognize advanced patients.
An increased D-dimer level is a concern in many hematologic malignancies and has been independently associated with a poor prognosis (11,12). However, only one study has described the D-dimer level in AL-CA patients and assessed its prognostic value (13). The clinical applicability of this variate is attractive, but it needs further verification.
Therefore, we aimed to assess whether the LVMWI has satisfactory short-term prognostic potential for AL-CA patients and to construct a simple bivariate prognostic model based on LVMWI and D-dimer levels. We present the following article in accordance with the STROBE reporting checklist (available at https://qims.amegroups.com/article/view/10.21037/qims-22-386/rc).
Methods
Study population
Patients with biopsy-confirmed immunoglobulin AL-CA were retrospectively enrolled and analyzed from July 2015 to March 2021. The study was conducted in accordance with the Declaration of Helsinki (as revised in 2013). The study was approved by the Ethics Committee of the First Affiliated Hospital, Medical School of Zhejiang University, and individual consent for this retrospective analysis was waived.
The diagnostic criteria for AL-CA were as follows (14,15): (I) an endomyocardial or extracardiac biopsy with Congo red staining showing apple-green birefringence under polarized light after potassium permanganate pretreatment; (II) evidence of monoclonal kappa or lambda protein in serum/urine immunofixation electrophoresis or biopsy immunohistochemistry; and (III) echocardiographic features of cardiac involvement shown by the presence of a granular “sparkling” appearance of the myocardium as well as increased interventricular septum thickness and/or an left ventricular (LV) posterior wall that was greater than or equal to 12 mm thick, which could not be attributed to hypertension alone. Patients who met the following criteria were excluded: (I) previous diagnosis of AL-CA and completion of chemotherapy (n=8); (II) unavailable noninvasive brachial artery cuff blood pressure at the time of echocardiographic examination (n=1); (III) results with poor image quality (n=0); (IV) incomplete follow-up information (n=2); and (V) refusal to undergo chemotherapy (n=4).
Patient baseline clinical and demographic characteristics including age, gender, underlying diseases, blood pressure, New York Heart Association (NYHA) classification, and type of light-chain, as well as laboratory characteristics including the levels of natriuretic peptide, D-dimer, alkaline phosphatase, and creatinine (Cr), the estimated glomerular filtration rate (eGFR), and total bilirubin (TBil) were collected from the patients’ medical records within 2 weeks of the echocardiographic study. Among the data collected, the levels of N-terminal pro-B-type natriuretic peptide (NT-proBNP) and or B-type natriuretic peptide (BNP) were available and analyzed in 72 (91%) patients. Previous studies have shown that abnormal natriuretic peptide involves an NT-proBNP level greater than 8,500 pg/mL and or a BNP level greater than 700 pg/mL (16,17). The D-dimer level was available in all cases and was represented as fibrinogen equivalent units (FEU). The 6-month all-cause mortality was defined as the endpoint of the short-term outcome, which was registered by the electronic medical record system and telephone follow-up. The whole follow-up period was defined as the data from the initial echocardiograph to death or the last follow-up, which occurred in September 2021.
Echocardiography
Comprehensive 2-dimensional (2D) echocardiography (Vivid E9; GE Vingmed Ultrasound, Horten, Norway) and offline analysis were performed by a single experienced investigator. Measurements in this study were in accordance with the guidelines of the American Echocardiography Association (18,19).
The LV end-diastolic posterior wall and interventricular septum thickness were measured in the parasternal long axis view. The left ventricular ejection fraction (LVEF) was measured by the biplane Simpson method. The normal value of LVEF was defined as ≥52% for males and ≥54% for females, according to the recommendations from current guidelines (18). An apical 4-chamber view was acquired to measure the peak mitral flow velocities during early diastole (E). Tissue Doppler echocardiography was performed to measure the early diastolic mitral annular velocity (e’) at the lateral and septal sides. Lateral and septal velocities were averaged, and the value of E/e’ was defined as the ratio of E and averaged e’.
Echocardiographic recordings were processed offline using dedicated software (EchoPAC 203; GE Vingmed Ultrasound). Poor image quality was defined as a frame rate of fewer than 40 frames per second or an inability to visualize or adequately trace more than 2 myocardial segments. According to the description from Russell et al. (8), LVMWI was calculated by the pressure-strain loop (PSL), which integrates LVLS and brachial artery cuff blood pressure, and was synchronized with echocardiography-derived valvular timing events. LVLS represents the average peak systolic LS in 3 views (apical long axis, 2-chamber, and 4-chamber views), using 2D speckle tracking imaging. Regions of interest were adjusted to optimize the tracer results. Noninvasive systolic brachial artery cuff pressure, as a surrogate for LV systolic pressure, was combined with strain to construct the PSL. The area of LV PSL represents the myocardial work from the mitral valve’s closure to its opening. The constructive work consists of the positive work for contracting myocardium in systole and the negative work for lengthening in isovolumetric relaxation. In contrast, the wasted work consists of the negative work in systole and the positive work in isovolumetric relaxation. Constructive work divided by the sum of constructive and wasted work provided the myocardial work efficiency. Based on the LV short axis views, LV was evaluated at 3 regions: apical, mid, and basal. According to the formula previously reported (20), the relative regional work ratio was defined as the average apical LVMWI divided by the sum of the average mid and basal LVMWI values.
Statistical analysis
Continuous variables were presented as the mean ± standard deviation or median (interquartile range) according to the normality, which was verified by the Kolmogorov-Smirnov test. To compare clinical characteristics between different CA subgroups, the Student’s t-test or the Mann-Whitney U test was used, as appropriate. Categorical variables were presented as a number (frequency) and compared using the chi-squared test or Fisher’s exact test.
Spearman correlation analysis was used to explore the association between LVMWI and other clinical characteristics. A correlation coefficient greater than 0.6 was considered as collinearity. The time-dependent receiver operating characteristic (ROC) and the area under the curve (AUC) were used to assess the accuracy of echocardiographic indicators in predicting outcomes at different time points. The Youden index was calculated to find the optimal cut-off value. Logistic regression was used to screen potential prognostic factors and structure a bivariate prognosis model. Multivariate nested logistic regression was used to verify the cumulative prognostic value of variates with log-likelihood chi-squared tests. Kaplan-Meier analysis was used to evaluate the cumulative mortality rate of the bivariate model based on the log-rank test. Intra-observer variabilities of LVMWI were assessed using the intraclass correlation coefficient (ICC) in 10 randomly selected patients with AL-CA. The software packages SPSS 23.0 (IBM Corp., Armonk, NY, USA) and R (version 4.0.5; The R Foundation for Statistical Computing, Vienna, Austria) were used for statistical analyses. A P value less than 0.05 was considered statistically significant.
Results
Study population
A total of 79 patients with AL-CA were enrolled in this study (Figure S1) (mean age, 62.7±7.9 years; 72% male). There were 57 patients (72%) with a positive lambda light chain, and 22 patients (28%) with a positive kappa light chain. The median follow-up time was 21 months (3–36 months). In total, chemotherapy was planned in all patients but was ultimately not performed in 6 (8%) patients, all of whom died prior to undergoing chemotherapy. The median time between echocardiographic measure and death was 10 (range, 6–40) days. The median time between chemotherapy and the echocardiographic operation was 9 (range, 4–22) days. Between patients with or without natriuretic peptide, no statistical difference was found in mortality, age, gender, chronic disease, blood pressure, or LVMWI.
The clinical characteristics of patients are listed in Table 1. A total of 23 (29%) patients died in the first 6 months; these patients had a lower level of systolic pressure and eGFR, a higher proportion of NYHA Class >II and abnormal natriuretic peptide, and a higher level of D-dimer, Cr, and TBil. Almost all patients (22/23, 96%) died of cardiac events. Only 1 patient died of severe liver failure due to primary involvement of the liver.
Table 1
Variables | Total (n=79) | All-cause mortality during 6 months | |
---|---|---|---|
Survivors (n=56) | Non-survivors (n=23) | ||
Clinical characteristics | |||
Age (year) | 62.7±7.9 | 62.1±7.6 | 64.0±8.5 |
Gender (male, %) | 57 (72.2) | 41 (73.2) | 16 (69.6) |
Hypertension (%) | 19 (24.1) | 14 (25.0) | 5 (21.7) |
Diabetes (%) | 8 (10.1) | 6 (10.7) | 2 (8.7) |
Coronary heart disease (%) | 3 (3.8) | 1 (1.8) | 2 (8.7) |
SP (mmHg) | 103.0 (93.0, 115.5) | 105.0 (94.0, 117.0) | 95.0 (89.0, 107.0)* |
DP (mmHg) | 64.0 (59.0, 76.0) | 67.5 (59.5, 77.0) | 62.0 (56.5, 67.5) |
NYHA Class >II (%) | 39 (49.4) | 19 (33.9) | 20 (87.0)*** |
Type of light-chain (lambda, %) | 57 (72.2) | 40 (71.4) | 17 (73.9) |
Laboratory characteristics | |||
Abnormal natriuretic peptidea (%) | 31/72 (43.1) | 15/50 (30.0) | 16/22 (72.7)** |
D-dimer (FEU) (μg/mL) | 1.0 (0.7, 2.0) | 0.9 (0.5, 1.5) | 1.9 (1.1, 4.6)** |
ALP (U/L) | 90.0 (73.5, 127.0) | 89.5 (75.0, 118.0) | 91.0 (70.0, 156.5) |
Cr (μmol/L) | 91.0 (79.0, 122.5) | 86.0 (74.5, 107.0) | 105.0 (87.5, 173.5)* |
eGFR (mL/min/1.73 m2) | 74.4 (53.1, 87.4) | 81.6 (59.3, 89.3) | 66.2 (33.0, 76.3)** |
TBil (μmol/L) | 7.4 (4.8, 13.1) | 6.6 (4.2, 10.4) | 12.0 (7.2, 20.9)** |
a, n=72. Data are expressed as mean ± standard deviation, median (interquartile range), or number (frequency). Abnormal natriuretic peptide means BNP >700 pg/mL and or NT-proBNP >8,500 pg/mL. The level of D-dimer was represented as FEU. *, P<0.05; **, P<0.01; ***, P<0.001; comparison between survivor and non-survivor during 6 months. AL-CA, light-chain cardiac amyloidosis; SP, systolic pressure; DP, diastolic pressure; NYHA, New York Heart Association; FEU, fibrinogen equivalent units; ALP, alkaline phosphatase; Cr, creatinine; eGFR, estimated glomerular filtration rate; TBil, total bilirubin; BNP, B-type natriuretic peptide; NT-proBNP, N-terminal pro-B-type natriuretic peptide.
Echocardiographic characteristics
As shown in Table 2, echocardiographic characteristics were compared between survivors and non-survivors. In the first 6 months, non-survivors had thicker interventricular septal and posterior wall thickness, a higher level of LV mass index, left atrial volume index, E/e’ ratios, and tricuspid regurgitation gradient, and a lower level of LVEF.
Table 2
Variables | Total (n=79) | All-cause mortality in 6 months | |
---|---|---|---|
Survivors (n=56) | Non-survivors (n=23) | ||
General echocardiographic characteristics | |||
IVST (mm) | 17.3±2.8 | 16.6±2.5 | 18.9±3.1** |
PWT (mm) | 15.6±3.0 | 15.1±2.8 | 16.7±3.2* |
LVMI (g/m2) | 138.5±50.4 | 125.8±35.3 | 169.4±67.0** |
LAVI (mL/m2) | 37.6±13.9 | 34.7±12.7 | 44.5±14.7** |
E/e’ (ratio) | 17.6±8.4 | 15.9±7.6 | 21.6±9.1** |
LVEF (%) | 59.0 (50.5, 61.5) | 60.0 (55.0, 62.5) | 48.0 (43.5, 56.5)*** |
TR gradient (m/s) | 2.6 (2.4, 2.9) | 2.5 (2.4, 2.8) | 2.8 (2.6, 3.0)* |
Two-dimensional speckle tracking imaging | |||
LVLS (%) | −12.3±4.2 | −13.6±3.7 | −9.3±4.0*** |
LVMWI (mmHg%) | 860.0 (672.0, 1,231.0) | 1,029.5 (815.5, 1,382.5) | 593.0 (487.5, 753.0)*** |
LVMCW (mmHg%) | 1,027.0 (809.5, 1,492.0) | 1,243.5 (927.0, 1,610.5) | 741.0 (597.0, 891.5)*** |
LVMWW (mmHg%) | 78.0 (44.0, 105.0) | 80.5 (44.5, 116.5) | 69.0 (41.0, 99.0) |
LVMWE (%) | 90.0 (86.0, 93.0) | 92.0 (89.0, 94.0) | 86.0 (83.5, 89.5)*** |
Relative regional work ratio | 0.8 (0.7, 1.2) | 0.8 (0.6, 1.0) | 1.1 (0.7, 1.3)* |
Data are expressed as mean ± standard deviation, median (interquartile range). *, P<0.05; **, P<0.01; ***, P<0.001; comparison between survivor and non-survivor during 6 months. AL-CA, light-chain cardiac amyloidosis; IVST, interventricular septal thickness; PWT, posterior wall thickness; LVMI, left ventricular mass index; LAVI, Left atrial volume index; E/e’, ratio of peak early diastolic mitral inflow velocity to peak early diastolic mitral annular velocity; LVEF, left ventricular ejection fraction; TR, tricuspid regurgitation; LVLS, left ventricular longitudinal strain; LVMWI, left ventricular myocardial work index; LVMCW, left ventricular myocardial constructive work; LVMWW, left ventricular myocardial wasted work; LVMWE, left ventricular myocardial work efficiency.
The 2D speckle tracking imaging reported a lower magnitude of LVLS (the absolute value), LVMWI, LV myocardial constructive work, and work efficiency in the non-survivor group. No statistical difference was found in LV myocardial wasted work between these 2 groups. Further, the level of relative regional work ratio was significantly higher in the non-survivor group than in the survivor group.
Time-dependent ROC curves analysis
Figure 1 shows representative PSLs and bull’s eye maps in patients with normal cardiac function and AL-CA. To explore the accuracy of LVMWI for predicting all-cause mortality at different time points, time-dependent ROC was performed in this study (Figure 2). The AUC of 6-month, 1-year, and 2-year all-cause mortality for LVMWI were 0.805, 0.788, and 0.711, respectively. The continuous AUC curve showed that the LVMWI had the best predictive potential at the 6-month time point.
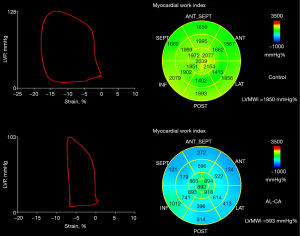
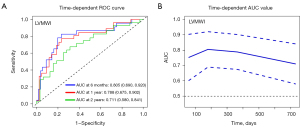
Univariable and multivariate analysis for 6-month all-cause mortality
Univariable logistic regression analysis was used to screen potential candidates to structure a bivariate model (Table 3). Among these variates, LVMWI showed the best potential in predicting a 6-month outcome [AUC =0.805; 95% confidence interval (CI): 0.690–0.920]. To observe the collinearity of variates, a correlation analysis between LVMWI and other clinical characteristics was performed (Table S1). Finally, 9 variates with less collinearity were combined with LVMWI to construct a bivariate model (Table 4 and Table S2). The results showed that the model, including LVMWI and D-dimer, had the optimum predictive value (AUC =0.877; 95% CI: 0.791–0.964). The model that included LVMWI and NYHA Class >II had an acceptable predictive value (AUC =0.854; 95% CI: 0.755–0.953). However, neither the abnormal natriuretic peptide nor the relative regional work ratio provided additional predictive information for LVMWI. A multivariate nested logistic regression model enrolled LVMWI, NYHA Class, and D-dimer. The chi-square value of NYHA class was 20.0 (P<0.001). The addition of LVMWI improved the power of the model (Δχ2=8.2; P=0.004). By adding D-dimer as an extra variable, the model was further improved significantly (Δχ2=10.3; P=0.001) (Figure 3).
Table 3
Variables | Univariate OR (95% CI) | P | AUC (95% CI) | P |
---|---|---|---|---|
Cardiac function | ||||
Systolic pressure (mmHg) | 0.966 (0.932, 1.001) | 0.057 | 0.649 (0.509, 0.790) | 0.038 |
NYHA Class >II | 12.982 (3.421, 49.266) | <0.001 | 0.765 (0.653, 0.877) | <0.001 |
Abnormal natriuretic peptidea | 6.222 (2.038, 19.000) | 0.001 | 0.714 (0.583, 0.845) | 0.004 |
Type of light-chain (lambda, %) | 0.882 (0.295, 2.642) | 0.823 | 0.512 (0.372, 0.653) | 0.863 |
LVMI (g/m2) | 1.019 (1.007, 1.032) | 0.002 | 0.703 (0.559, 0.848) | 0.005 |
LAVI (mL/m2) | 1.055 (1.015, 1.097) | 0.007 | 0.689 (0.558, 0.821) | 0.008 |
E/e’ | 1.087 (1.021, 1.158) | 0.010 | 0.692 (0.560, 0.823) | 0.008 |
LVEF (%) | 0.886 (0.829, 0.947) | <0.001 | 0.760 (0.630, 0.891) | <0.001 |
TR gradient (m/s) | 4.485 (1.008, 19.966) | 0.049 | 0.658 (0.522, 0.794) | 0.028 |
LVMWI (mmHg%) | 0.997 (0.995, 0.999) | <0.001 | 0.805 (0.690, 0.920) | <0.001 |
Relative regional work ratio (per 0.1 increase) |
1.216 (1.054, 1.404) | 0.008 | 0.670 (0.528, 0.812) | 0.018 |
Other laboratory characteristics | ||||
D-dimer (FEU) (μg/mL) | 1.421 (1.028, 1.965) | 0.034 | 0.743 (0.622, 0.863) | 0.001 |
ALP (U/L) | 1.002 (0.999, 1.004) | 0.197 | 0.503 (0.346, 0.661) | 0.961 |
Cr (μmol/L) | 1.012 (1.003, 1.022) | 0.011 | 0.681 (0.546, 0.817) | 0.012 |
TBil (μmol/L) | 1.048 (1.001, 1.097) | 0.046 | 0.729 (0.606, 0.853) | 0.001 |
a, n=72. Abnormal natriuretic peptide means BNP >700 pg/mL and or NT-proBNP >8,500 pg/mL. OR, odds ratio; CI, confidence interval; AUC, the area under the curve; NYHA, New York Heart Association; LVMI, left ventricular mass index; LAVI, left atrial volume index; E/e’, ratio of peak early diastolic mitral inflow velocity to peak early diastolic mitral annular velocity; LVEF, left ventricular ejection fraction; TR, tricuspid regurgitation; LVMWI, left ventricular myocardial work index; FEU, fibrinogen equivalent units; ALP, alkaline phosphatase; Cr, creatinine; TBil, total bilirubin; BNP, B-type natriuretic peptide; NT-proBNP, N-terminal pro-B-type natriuretic peptide.
Table 4
Models | Multivariate OR (95% CI) | P | The AUC of bivariate model |
---|---|---|---|
Model 1 | |||
LVMWI | 0.998 (0.996, 0.999) | 0.013 | |
NYHA Class >II | 7.606 (1.856, 31.159) | 0.005 | 0.854 (0.755, 0.953) |
Model 2 | |||
LVMWI | 0.997 (0.995, 0.999) | 0.001 | |
Abnormal natriuretic peptidea | 2.477 (0.676, 9.076) | 0.171 | 0.805 (0.690, 0.920) |
Model 3 | |||
LVMWI | 0.997 (0.995, 0.999) | <0.001 | |
Relative regional work ratio (per 0.1 increase) |
1.065 (0.911, 1.246) | 0.429 | 0.805 (0.690, 0.920) |
Model 4 | |||
LVMWI | 0.996 (0.994, 0.998) | 0.001 | |
D-dimer | 1.640 (1.006, 2.673) | 0.047 | 0.877 (0.791, 0.964) |
a, n=72. OR, odds ratio; CI, confidence interval; AUC, the area under the curve; LVMWI, left ventricular myocardial work index; NYHA, New York Heart Association.
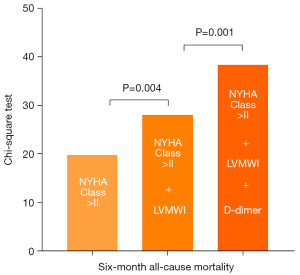
To construct a risk stratification for advanced patients with AL-CA, all participants were divided into 3 groups according to the cut-off value: Group 1, LVMWI >802 mmHg% and D-dimer <1.4 µg/mL FEU; Group 2, LVMWI <802 mmHg% or D-dimer >1.4 µg/mL FEU; and Group 3, LVMWI <802 mmHg% and D-dimer >1.4 µg/mL FEU. Kaplan-Meier analysis demonstrated that these 2 variates had a synergistic effect, as the accumulated 6-month mortality of patients in Group 3 was significantly higher than that of patients in Group 2 (86% vs. 30%; log-rank, P<0.001) and Group 1 (86% vs. 3%; log-rank, P<0.001). Additionally, the accumulated 6-month mortality of patients in Group 2 was higher than in Group 1 (30% vs. 3%; log-rank, P=0.003; Figure 4A). Similar results were also obtained from the analysis of short-term accumulated mortality for AL-CA patients with normal LVEF (Figure 4B). During the long-term follow-up, this simple model still showed clinical potential in risk stratification (Figure S2).
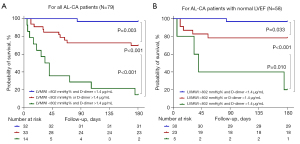
Intra-observer variability of LVMWI
To verify the intra-observer variability of LVMWI, the measurement of LVMWI from 10 randomly selected patients with AL-CA was independently performed by a single experienced investigator. The investigator measured the intra-observer variability of LVMWI twice on separate days with a washout period of more than 1 month. The intra-observer ICCs revealed good repeatability for LVMWI (Table S3).
Discussion
In our study, we found that LVMWI showed the best predictive potential of all-cause mortality at the 6-month time point for AL-CA patients, and D-dimer had a synergistic value with LVMWI for predicting short-term outcomes.
By relying on 2D speckle tracking imaging and brachial artery cuff blood pressure, LVMWI was measured as an extension to the existing LVLS. Doing so overcame the interference of afterload when assessing myocardial deformation and may provide a novel perspective for evaluating cardiac function. Unlike LVLS, LVMWI reflected the cardiac work in the whole cardiac cycle rather than during systole. Previous studies have reported the clinical potential of myocardial work parameters in several cardiovascular diseases (21-27). For patients with AL-CA, cardiac involvement is always more severe than transthyretin amyloidosis (28). A previous study reported that 6-month cardiac and renal responses were 26.1% and 22%, respectively, in AL amyloidosis treated with frontline bortezomib (5). Therefore, poor cardiac function and relatively delayed response to chemotherapy cause a higher proportion of early death for patients with AL-CA and necessitate a specific short-term risk stratification for this subgroup.
One study measured the level of LVMWI in 34 light chain and 66 transthyretin amyloidosis patients and identified its potential in predicting long-term outcomes (9). This team also found inefficient myocardial energy exploitation in patients with CA (29). Another study, which included 26 light chain and 92 transthyretin amyloidosis patients, reported similar results (10). However, no studies have focused on the short-term or long-term outcomes of patients with AL-CA alone. In our study, 79 patients diagnosed with AL-CA were enrolled, and a high mortality rate (29%) was reported in the first 6 months. Almost all patients died of cardiogenic events. Time-dependent ROC curve analysis showed that LVMWI had the best predictive potential at the 6-month time point. This is understandable because 6 months may reflect the “blank period” of chemotherapy, and the 6-month prognosis mainly depends on the patient’s own cardiac function. This may partly explain why chemotherapy does not improve the outcome of AL-CA patients with irreversible heart failure. Identifying this high-risk group through risk stratification and helping them safely pass the blank period could enable them to benefit from chemotherapy. Additionally, daratumumab, as a new treatment, has been shown to benefit patients with advanced AL-CA (30,31). How to accomplish careful patient selection under precise risk stratification is a quandary worth exploring.
In this study, the abnormal natriuretic peptide and relative regional work ratio failed to provide prognostic information for LVMWI, suggesting that LVMWI has an advantage in evaluating cardiac function. Further, a bivariate model including LVMWI and D-dimer was shown to have a synergistic value in predicting the 6-month prognosis. Patients with 2, 1, and none of the variates beyond the cut-off value showed a significantly different risk of 6-month all-cause mortality. This potential for risk stratification was also applied to the subgroup who had normal LVEF, reflecting its superiority in recognizing subclinical systolic dysfunction.
In patients with AL-CA, the level of D-dimer is less of a concern. However, its elevation has attracted great attention in many malignancies and is associated with a poor prognosis (12,32,33). Only one study retrospectively enrolled 897 patients with AL amyloidosis and found a high prevalence (approximately 50%) of elevated D-dimer. The prognostic analysis confirmed that D-dimer was associated with survival in all cardiac stages (13). These meaningful results suggested that D-dimer could provide additional prognostic information beyond cardiac function, which may be attributed to thrombophilia and fibrinolytic activation, vascular involvement and endothelial dysfunction, or the extent of organ involvement (13) Unlike the indicators reflecting the mechanical systolic and diastolic function, such as LVMWI, D-dimer is more likely to reflect a systemic, multi-organ involved state in the whole body. Although the prognostic value of D-dimer alone was not satisfactory, it provides a new perspective and supplement on the short-term prognosis of AL-CA. The application of this sample model may be meaningful to recognize moderate- and high-risk patients and help them through the early stages of the disease.
Limitations
The present study has some limitations. (I) This study was retrospective and conducted in a single center with a small sample size. To avoid overfitting of the prognostic model, only limited variates were selected. Further prospective, multicenter investigations with a larger sample size are required to confirm our findings. (II) The acknowledged Mayo score was unable to be reported because troponin (n=31), natriuretic peptide (n=7), and free light chain (n=39) were not available in all patients. Therefore, our findings can only be explained to a limited extent. To reduce the impact of missing data on the results, troponin and free light chain were not included in this study, and the natriuretic peptide was added as a variate to construct a bivariate model. (III) The LV stress-strain loop area, rather than the PSL area, is more persuasive in illustrating LVMWI (9). According to Laplace’s law, patients with CA are characterized by decreased LV wall stress levels, which are mainly attributed to the increase in ventricular wall thickness and decrease in the LV radius. (IV) Moreover, the use of brachial artery systolic pressure as the surrogate of LV pressure is imprecise. Therefore, the actual myocardial work of patients with AL-CA may be lower than that reported in our study.
Conclusions
The LVMWI, calculated by the PSL, is associated with the short-term all-cause mortality of patients with AL-CA. Further, D-dimer provides additional prognostic information for the LVMWI.
Acknowledgments
Funding: This work was supported by the National Natural Science Foundation of China (NSFC) (No. 81400192 to J Yang; No. 82070050 to X Wang; No. 82102240 to X Tao), the Natural Science Foundation of Zhejiang Province (No. LY19H020002 to J Yang), and the Zhejiang Provincial Medical and Health Science and Technology Project (No. 2021KY158 to B Wu).
Footnote
Reporting Checklist: The authors have completed the STROBE reporting checklist (available at https://qims.amegroups.com/article/view/10.21037/qims-22-386/rc).
Conflicts of Interest: All authors have completed the ICMJE uniform disclosure form (available at https://qims.amegroups.com/article/view/10.21037/qims-22-386/coif). The authors have no conflicts of interest to declare.
Ethical Statement: The authors are accountable for all aspects of the work in ensuring that questions related to the accuracy or integrity of any part of the work are appropriately investigated and resolved. The study was conducted in accordance with the Declaration of Helsinki (as revised in 2013). The study was approved by the Ethics Committee of the First Affiliated Hospital, Medical School of Zhejiang University, and individual consent for this retrospective analysis was waived.
Open Access Statement: This is an Open Access article distributed in accordance with the Creative Commons Attribution-NonCommercial-NoDerivs 4.0 International License (CC BY-NC-ND 4.0), which permits the non-commercial replication and distribution of the article with the strict proviso that no changes or edits are made and the original work is properly cited (including links to both the formal publication through the relevant DOI and the license). See: https://creativecommons.org/licenses/by-nc-nd/4.0/.
References
- Merlini G, Dispenzieri A, Sanchorawala V, Schönland SO, Palladini G, Hawkins PN, Gertz MA. Systemic immunoglobulin light chain amyloidosis. Nat Rev Dis Primers 2018;4:38. [Crossref] [PubMed]
- Gertz MA, Dispenzieri A, Sher T. Pathophysiology and treatment of cardiac amyloidosis. Nat Rev Cardiol 2015;12:91-102. [Crossref] [PubMed]
- Wechalekar AD, Schonland SO, Kastritis E, Gillmore JD, Dimopoulos MA, Lane T, Foli A, Foard D, Milani P, Rannigan L, Hegenbart U, Hawkins PN, Merlini G, Palladini G. A European collaborative study of treatment outcomes in 346 patients with cardiac stage III AL amyloidosis. Blood 2013;121:3420-7. [Crossref] [PubMed]
- Kristen AV, Brokbals E, Aus dem Siepen F, Bauer R, Hein S, Aurich M, Riffel J, Behrens HM, Krüger S, Schirmacher P, Katus HA, Röcken C. Cardiac Amyloid Load: A Prognostic and Predictive Biomarker in Patients With Light-Chain Amyloidosis. J Am Coll Cardiol 2016;68:13-24. [Crossref] [PubMed]
- Ravichandran S, Cohen OC, Law S, Foard D, Fontana M, Martinez-Naharro A, Whelan C, Gillmore JD, Lachmann HJ, Sachchithanantham S, Mahmood S, Hawkins PN, Wechalekar AD. Impact of early response on outcomes in AL amyloidosis following treatment with frontline Bortezomib. Blood Cancer J 2021;11:118. [Crossref] [PubMed]
- Ternacle J, Bodez D, Guellich A, Audureau E, Rappeneau S, Lim P, Radu C, Guendouz S, Couetil JP, Benhaiem N, Hittinger L, Dubois-Randé JL, Plante-Bordeneuve V, Mohty D, Deux JF, Damy T. Causes and Consequences of Longitudinal LV Dysfunction Assessed by 2D Strain Echocardiography in Cardiac Amyloidosis. JACC Cardiovasc Imaging 2016;9:126-38. [Crossref] [PubMed]
- Hubert A, Le Rolle V, Leclercq C, Galli E, Samset E, Casset C, Mabo P, Hernandez A, Donal E. Estimation of myocardial work from pressure-strain loops analysis: an experimental evaluation. Eur Heart J Cardiovasc Imaging 2018;19:1372-9. [Crossref] [PubMed]
- Russell K, Eriksen M, Aaberge L, Wilhelmsen N, Skulstad H, Remme EW, Haugaa KH, Opdahl A, Fjeld JG, Gjesdal O, Edvardsen T, Smiseth OA. A novel clinical method for quantification of regional left ventricular pressure-strain loop area: a non-invasive index of myocardial work. Eur Heart J 2012;33:724-33. [Crossref] [PubMed]
- Clemmensen TS, Eiskjær H, Ladefoged B, Mikkelsen F, Sørensen J, Granstam SO, Rosengren S, Flachskampf FA, Poulsen SH. Prognostic implications of left ventricular myocardial work indices in cardiac amyloidosis. Eur Heart J Cardiovasc Imaging 2021;22:695-704. [Crossref] [PubMed]
- Roger-Rollé A, Cariou E, Rguez K, Fournier P, Lavie-Badie Y, Blanchard V, Roncalli J, Galinier M, Carrié D, Lairez OToulouse Amyloidosis Research Network collaborators. Can myocardial work indices contribute to the exploration of patients with cardiac amyloidosis? Open Heart 2020;7:e001346. [Crossref] [PubMed]
- Edwards RL, Rickles FR, Moritz TE, Henderson WG, Zacharski LR, Forman WB, Cornell CJ, Forcier RJ, O'Donnell JF, Headley E. Abnormalities of blood coagulation tests in patients with cancer. Am J Clin Pathol 1987;88:596-602. [Crossref] [PubMed]
- Ay C, Dunkler D, Pirker R, Thaler J, Quehenberger P, Wagner O, Zielinski C, Pabinger I. High D-dimer levels are associated with poor prognosis in cancer patients. Haematologica 2012;97:1158-64. [Crossref] [PubMed]
- Pudusseri A, Sanchorawala V, Sloan JM, Bever KM, Doros G, Kataria S, Sarosiek S. Prevalence and prognostic value of D-dimer elevation in patients with AL amyloidosis. Am J Hematol 2019;94:1098-103. [Crossref] [PubMed]
- Quarta CC, Solomon SD, Uraizee I, Kruger J, Longhi S, Ferlito M, Gagliardi C, Milandri A, Rapezzi C, Falk RH. Left ventricular structure and function in transthyretin-related versus light-chain cardiac amyloidosis. Circulation 2014;129:1840-9. [Crossref] [PubMed]
- Uzan C, Lairez O, Raud-Raynier P, Garcia R, Degand B, Christiaens LP, Rehman MB. Right ventricular longitudinal strain: a tool for diagnosis and prognosis in light-chain amyloidosis. Amyloid 2018;25:18-25. [Crossref] [PubMed]
- Dispenzieri A, Gertz MA, Kyle RA, Lacy MQ, Burritt MF, Therneau TM, Greipp PR, Witzig TE, Lust JA, Rajkumar SV, Fonseca R, Zeldenrust SR, McGregor CG, Jaffe AS. Serum cardiac troponins and N-terminal pro-brain natriuretic peptide: a staging system for primary systemic amyloidosis. J Clin Oncol 2004;22:3751-7. [Crossref] [PubMed]
- Lilleness B, Ruberg FL, Mussinelli R, Doros G, Sanchorawala V. Development and validation of a survival staging system incorporating BNP in patients with light chain amyloidosis. Blood 2019;133:215-23. [Crossref] [PubMed]
- Lang RM, Badano LP, Mor-Avi V, Afilalo J, Armstrong A, Ernande L, Flachskampf FA, Foster E, Goldstein SA, Kuznetsova T, Lancellotti P, Muraru D, Picard MH, Rietzschel ER, Rudski L, Spencer KT, Tsang W, Voigt JU. Recommendations for cardiac chamber quantification by echocardiography in adults: an update from the American Society of Echocardiography and the European Association of Cardiovascular Imaging. Eur Heart J Cardiovasc Imaging 2015;16:233-70. [Crossref] [PubMed]
- Nagueh SF, Smiseth OA, Appleton CP, Byrd BF 3rd, Dokainish H, Edvardsen T, Flachskampf FA, Gillebert TC, Klein AL, Lancellotti P, Marino P, Oh JK, Popescu BA, Waggoner AD. Recommendations for the Evaluation of Left Ventricular Diastolic Function by Echocardiography: An Update from the American Society of Echocardiography and the European Association of Cardiovascular Imaging. J Am Soc Echocardiogr 2016;29:277-314. [Crossref] [PubMed]
- Senapati A, Sperry BW, Grodin JL, Kusunose K, Thavendiranathan P, Jaber W, Collier P, Hanna M, Popovic ZB, Phelan D. Prognostic implication of relative regional strain ratio in cardiac amyloidosis. Heart 2016;102:748-54. [Crossref] [PubMed]
- Chan J, Edwards NFA, Khandheria BK, Shiino K, Sabapathy S, Anderson B, Chamberlain R, Scalia GM. A new approach to assess myocardial work by non-invasive left ventricular pressure-strain relations in hypertension and dilated cardiomyopathy. Eur Heart J Cardiovasc Imaging 2019;20:31-9. [Crossref] [PubMed]
- Hiemstra YL, van der Bijl P, El Mahdiui M, Bax JJ, Delgado V, Marsan NA. Myocardial Work in Nonobstructive Hypertrophic Cardiomyopathy: Implications for Outcome. J Am Soc Echocardiogr 2020;33:1201-8. [Crossref] [PubMed]
- Boe E, Russell K, Eek C, Eriksen M, Remme EW, Smiseth OA, Skulstad H. Non-invasive myocardial work index identifies acute coronary occlusion in patients with non-ST-segment elevation-acute coronary syndrome. Eur Heart J Cardiovasc Imaging 2015;16:1247-55. [Crossref] [PubMed]
- Hubert A, Gallard A, Rolle VL, Smiseth OA, Leclercq C, Voigt JU, Galli E, Galand V, Hernandez A, Donal E. Left ventricular strain for predicting the response to cardiac resynchronization therapy: two methods for one question. Eur Heart J Cardiovasc Imaging 2021;jeaa422. Epub ahead of print. [Crossref] [PubMed]
- Duchenne J, Aalen JM, Cvijic M, Larsen CK, Galli E, Bézy S, Beela AS, Ünlü S, Pagourelias ED, Winter S, Hopp E, Kongsgård E, Donal E, Fehske W, Smiseth OA, Voigt JU. Acute redistribution of regional left ventricular work by cardiac resynchronization therapy determines long-term remodelling. Eur Heart J Cardiovasc Imaging 2020;21:619-28. [Crossref] [PubMed]
- Lustosa RP, Butcher SC, van der Bijl P, El Mahdiui M, Montero-Cabezas JM, Kostyukevich MV, Rocha De Lorenzo A, Knuuti J, Ajmone Marsan N, Bax JJ, Delgado V. Global Left Ventricular Myocardial Work Efficiency and Long-Term Prognosis in Patients After ST-Segment-Elevation Myocardial Infarction. Circ Cardiovasc Imaging 2021;14:e012072. [Crossref] [PubMed]
- Zhu M, Wang Y, Cheng Y, Su Y, Chen H, Shu X. The value of non-invasive myocardial work indices derived from left ventricular pressure-strain loops in predicting the response to cardiac resynchronization therapy. Quant Imaging Med Surg 2021;11:1406-20. [Crossref] [PubMed]
- Kumar S, Li D, Joseph D, Trachtenberg B. State-of-the-art review on management of end-stage heart failure in amyloidosis: transplant and beyond. Heart Fail Rev 2022;27:1567-78. [Crossref] [PubMed]
- Clemmensen TS, Eiskjær H, Mikkelsen F, Granstam SO, Flachskampf FA, Sørensen J, Poulsen SH. Left Ventricular Pressure-Strain-Derived Myocardial Work at Rest and during Exercise in Patients with Cardiac Amyloidosis. J Am Soc Echocardiogr 2020;33:573-82. [Crossref] [PubMed]
- Gounot R, Le Bras F, Dupuis J, Oghina S, Bodez D, Roulin L, Maarek A, Ladaique A, Beldi-Ferchiou A, Poullot E, Molinier-Frenkel V, Haioun C, Damy T, Belhadj K, Lemonnier F. Daratumumab is safe and induces a rapid hematological response in light-chain amyloidosis with severe cardiac impairment. Leuk Lymphoma 2021;62:979-83. [Crossref] [PubMed]
- Jeryczynski G, Antlanger M, Duca F, Binder-Rodriguez C, Reiter T, Simonitsch-Klupp I, Bonderman D, Kain R, Krauth MT, Agis H. First-line daratumumab shows high efficacy and tolerability even in advanced AL amyloidosis: the real-world experience. ESMO Open 2021;6:100065. [Crossref] [PubMed]
- Zhang PP, Sun JW, Wang XY, Liu XM, Li K. Preoperative plasma D-dimer levels predict survival in patients with operable non-small cell lung cancer independently of venous thromboembolism. Eur J Surg Oncol 2013;39:951-6. [Crossref] [PubMed]
- Altiay G, Ciftci A, Demir M, Kocak Z, Sut N, Tabakoglu E, Hatipoglu ON, Caglar T. High plasma D-dimer level is associated with decreased survival in patients with lung cancer. Clin Oncol (R Coll Radiol) 2007;19:494-8. [Crossref] [PubMed]