Liver-spleen contrast standardization of gadolinium-ethoxybenzyl-diethylenetriamine penta-acetic acid-enhanced magnetic resonance imaging based on cross-calibration
Introduction
In the periodic screening examination for the detection of hepatocellular carcinoma, gadolinium-ethoxybenzyl-diethylenetriamine penta-acetic acid (Gd-EOB-DTPA)-enhanced magnetic resonance imaging (MRI), which includes the assessment of liver function, can provide the benefits of cost savings and convenience to patients with chronic liver diseases (1,2). To assess liver function using Gd-EOB-DTPA-enhanced MRI, the following four approaches have been suggested (2): T1 or T2* relaxometry (3), dynamic contrast-enhanced MRI (4), biliary enhancement (5), and liver-spleen contrast (LSC) (6-9). However, it can be difficult to distinguish between normal and Child-Push A using the T1 or T2* relaxometry evaluation method (3). In the calibration of signal intensity (SI) data, the hepatobiliary phase images in dynamic contrast-enhanced MRI differ from another phase images, such as the precontrast images, because other sequences are performed during the long wait time between the equilibrium and hepatobiliary phases (10). Additionally, these two methods require dedicated software for calculation or analysis and are costly (2-4). In biliary enhancement, random errors may be a problem when the region of interest (ROI) is too small to measure SI (11,12). On the other hand, the acquisition of LSC is possible by one breath hold in the hepatobiliary phase, and does not require expensive software (2,6). ROIs may be set at an arbitrary placement and adequate size (2,7). Moreover, LSC can be used to separate normal cases from the Grade 1 group of the albumin-bilirubin (ALBI) Grades (13). Therefore, LSC is the simplest and most common technique among these four methods (2).
In pharmacokinetic systems, Gd-EOB-DTPA has specifically taken up normal hepatocytes for bilirubin transport and metabolism through receptors on hepatocyte membranes (6). Gd-EOB-DTPA is also distributed non-specifically to the extracellular fluid (ECF) (14). Therefore, the contrast enhancement effect of Gd-EOB-DTPA is caused not only by hepatocyte uptake but also by an ECF pool (15). Due to the fact that liver enhancement of the ECF can approximate spleen enhancement (14,15), LSC is adopted as the index of hepatic function in Gd-EOB-DTPA-enhanced MRI. While liver function tests through laboratory examination evaluate the entire liver, the LSC can also evaluate zonal and lobar differences of the liver with high-resolution images (16). Moreover, Gd-EOB-DTPA-enhanced MRI can evaluate the future remnant liver functional reserve after hepatectomy by adding the liver volume to the LSC because the volume data can be obtained (15). Moderate correlations have also observed between the LSC and other liver function indexes, such as ALBI score, Child-Push class, and indocyanine green retention rate at 15 min (6,13,15). The LSC levels are also associated with severity of chronic liver diseases (13).
However, LSC is slightly inferior in quantitative value, because the measured SI to calculate LSC is highly dependent on the MRI scanner, acquisition parameters, and scanned body regions or patients (Figure 1) (2,7). For example, even if the type of coil is different, the LSC value differs by approximately 30% (7). For LSC, the conversion of SIs into relaxation rates through T1 relaxation time has been reported (8,9). However, these methods were verified only by a single device, which is MRI scanner-coil combination, from the same manufacturer, and addressing the limitation of LSC dependence on MRI scanners and acquisition parameters was questionable. Although the differences in the contrast among the devices can be minimized by aligning the acquisition parameters with the sensitivity corrections of phased array uniformity enhancement (PURE) (17), this method is not too suitable for the current situation of diverse sequences.
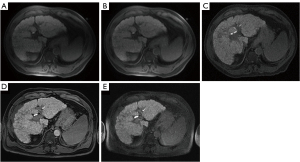
Therefore, to address this problem and as a new way of the standardization of LSC, we invented the conversion of the LSC into reference LSC (rLSC) acquired with another device through regression lines calculated from phantom contrasts (PC). The aim of this work was to assess the accuracy of this cross-calibration conversion technique, regarding the relationship between two different MRI scanner-coil combinations as a pilot study of standardization. We present the following article in accordance with the STROBE reporting checklist (available at https://qims.amegroups.com/article/view/10.21037/qims-22-174/rc).
Methods
Patients
All patient information were retrospectively collected and anonymized prior to analysis, by adding symbols, such as numbers. This study was conducted in accordance with the Declaration of Helsinki (as revised in 2013). The study design was approved by the ethics review board of the Japan Community Healthcare Organization Hokkaido Hospital (No. 2018-21). Given the retrospective nature of this study, the requirement for written informed consent was waived. We appealed for consent for the secondary use of medical data, by disclosing the research contents on a physical notice board and home page (https://hokkaido.jcho.go.jp/wp-content/uploads/2018/09/NEW2018-21.pdf), as per the provisions of the "Ethical guidelines for medical and health researches involving human subjects” (Ministry of Education, Culture, Sports, Science and Technology and Ministry of Health, Labour, and Welfare bulletin No.3 of 2014).
Images from patients who underwent Gd-EOB-DTPA-enhanced MRI using different MRI scanner-coil combinations from January 4, 2008 to March 31, 2021 were eligible for this study. Images with artifacts and those obtained from patients who had undergone splenectomy were excluded. The collected patient information was classified according to the model of the used MRI scanner. As an index for liver function evaluation, the ALBI score (18) was calculated using the values of the biochemical tests done within two weeks before and after the MRI, as follows:
Contrast agents
For Gd-EOB-DTPA-enhanced MRI, 0.025 mM/kg body weight of Gd-EOB-DTPA (Primovist 0.25 mM/mL; Bayer Yakuhin Ltd., Osaka, Japan) was intravenously injected, and the images in the hepatobiliary phase were acquired after 60 minutes at which the Gd-EOB-DTPA of the liver ECF space sufficiently washes out and the LSC reaches a state of equilibrium (14).
Twin phantom design
To measure the contrast distribution in the anterior-posterior direction before verifying the cross-calibration, the 2.0 and 5.0 mM/L phantoms were made in 2.0 L PET bottles (10.5 cm × 9.0 cm × 23.0 cm) using reagent grade anhydrous copper (II) sulfate (CuSO4) (Alfa Aesar, Thermo Fisher Scientific, Heysham, United Kingdom). The placement of the 5.0 and 2.0 mM/L CuSO4 phantoms is shown in Figure 2A,2B.
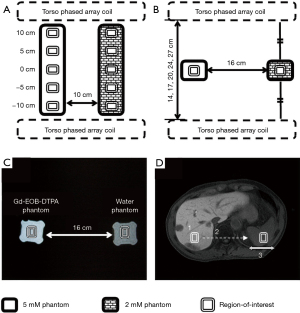
For cross-calibration, the 0.06, 0.14, 0.27, 0.63, 1.37, and 2.82 mM/L phantoms of the Gd-EOB-DTPA contrast, referred to the previous research regarding gadolinium-based contrast medium (19), were made in 500-mL PET bottles (5.6 cm × 5.6 cm × 15.0 cm). Additionally, a phantom was made using purified water in a 500-mL PET bottle. The placement of the Gd-EOB-DTPA aqueous solution and purified water phantoms was shown in Figure 2C. PC was measured by changing only the molarity of the Gd-EOB-DTPA phantoms.
The T1 and T2 values of these phantoms were measured using inversion recovery and spin echo methods, respectively.
Imaging apparatuses and procedures
The device used to acquire rLSC in all examinations was a scanner with a four-channel torso phased array coil (T-PAC) [SIGNA Horizon LX (Echo Speed) 1.5T Ver. 9.1, General Electric Healthcare Japan, Tokyo, Japan]. For the acquisition of converted LSC, the aforementioned device with another body coil (BC) that was built into MRI scanner was used for sample A, and another scanner with a 32-channel sensitivity encoding parallel imaging torso cardiac coil (SENSE-PAC) and a quadrature BC (Q-BC) (Achieva 1.5T A-series R.2.6, Philips Medical Systems Japan, Tokyo, Japan) was used for sample B on another day. The image processing workstation (Advantage Workstation Ver. 4.0, General Electric Healthcare Japan, Tokyo, Japan) and software (Basic Viewing, Philips Medical Systems Japan, Tokyo, Japan) were used to measure the SIs of T-PAC/BC and SENSE-PAC/Q-BC, respectively.
In all phantoms and patients, T1-weighted transverse images with T-PAC, BC SENSE-PAC, and Q-BC, which are shown in Figure 1A,1C-1E, respectively, were acquired using scan parameters presented in Table 1. The slice gap in two-dimensional images was set at ≥20% of the slice thickness to minimize the effect of crosstalk among slices (7). The reduction factor of image-based parallel imaging was 2.0. Surface coil intensity correction (SCIC) was not adopted (Figure 1A) because the contrast among organs reduces due to additional smoothing technique (Figure 1B) (20). PURE could not be used because it was not built into the scanner used. Contrast level appearance (CLEAR) was applied in sample B (Figure 1D).
Table 1
Device scan parameter | SIGNA Horizon LX | Achieva A-series | |
---|---|---|---|
Phased array coil | Body coil | ||
Dimension | 2 | 3 | 2 |
Sequence | Fast SPGR | FFE, e-THRIVE | FFE of multislice |
Fat suppression | CHESS | SPAIR | ProSet |
Echo time (ms) | 1.3 | 2.3 | 5.1 |
Repetition time (ms) | 148 | 4.6 | 126 |
Flip angle (°) | 70 | 10 | 80 |
Number of excitations | 1 | 1 | 1 |
k-space trajectory | Sequential | Linear | Linear |
Band width (Hz/pixel) | 325.5 | 360.8 | 313.8 |
Field of view (mm) | 430 | 370 | 420 |
Matrix (frequency × phase) | 256×160 | 240×153 | 256×160 |
Scan percentage (%) | 100 | 79.8 | 62.5 |
Phase field of view (%) | 75 | 80 | 100 |
Slice thickness (mm) | 6.5 | 4.0 | 6.5 |
Slice gap (mm) | 3.5 | 0 | 3.5 |
Slice scan order | Slice number | – | Interleaved |
Slice number | 20 | 40 | 7 |
Respiration | Breath hold | Breath hold | Breath hold |
Scan time (s) | 10.0 | 14.3 | 20.7 |
SPGR, spoiled gradient recalled acquisition of steady state; FFE, fast field echo; e-THRIVE, enhanced-T1 high resolution isotropic volume excitation; CHESS, chemical shift saturation; SPAIR, spectral attenuated with inversion recovery; ProSet, principle of selective excitation technique.
Image analysis
The ROI size of a rectangle was set to approximately 50 pixels, which is reported to be the minimum number of pixels required to suppress random error (11). The ROI was placed at the location with the smallest standard deviation in order to minimize signal fluctuation (7,11). For measuring the PC, the ROI was set at the center of the phantom (Figure 2C). For measuring the LSC, the vasculature and tumor were excluded from the ROI locations (Figure 2D). The ROIs were acquired multiple times from five continuous cross sections at the right lobe (12).
On PAC, the SI was the concave distribution in the anterior–posterior direction, which was perpendicular to the anterior and posterior PACs, because the PAC was made by linking the small plural radiofrequency coils on a plane (Figure 1A) (21). Furthermore, even if the SCIC was applied, the SI drop remained in the central portion of the images (Figure 1B) (21). Therefore, in measuring the contrasts using T-PAC, two ROIs of the liver and spleen were placed at the same distance from the PACs in order to keep the same condition of the SI drop between these two ROIs (Figure 2C,2D). For measuring the LSC, the ROI in the liver was set using the smallest standard deviation, followed by placement of the ROI in the spleen at the same height as that of the liver (Figure 2D) (7). However, when using the built-in BC, aligning the heights of these two ROIs is not needed, because the BC had uniform sensitivity in effective imaging regions (Figure 1C,1E). In the SENSE-PAC with CLEAR, these ROIs are not aligned (Figure 1D).
Contrasts were calculated using the following Michelson contrast formulas (13,22):
and
In the PCs, the SI1 and SI2 represented the phantom SIs on the liver and spleen sides, respectively. For the LSC, the SI1 and SI2 represented the SIs of the liver and spleen, respectively. The measured value was the average of the contrast values of five images.
The Michelson contrast is an index that normalizes the difference between light and dark signals with the average value of both and is used in optical systems, such as the theory of signal processing (22,23). When an object on MRI has a wide dynamic range, the Michelson contrast, compared with the contrast ratio (6,16), is said to closely approximate the actual evaluation, because the object on contrast ratio, which is the ratio of two SIs, is not assumed to be a logarithmic response (22,23). In this study, the Michelson contrast was selected with the intent to exclude the liver enhancement effect of ECF. For the comparison with previous studies using other contrast formulas, the conversion formula from contrast ratio (Cr) and Weber contrast (CW) (15) to Michelson contrast (CM) is shown below:
Verification of the setting method of two ROIs
To verify the method of aligning the height of the two ROIs adopted in this study, the phantoms of CuSO4 aqueous solution were perpendicularly placed, and the PC distribution in the anterior-posterior direction was measured at 0, 5, and 10 cm away from the center between the anterior and posterior T-PACs (Figure 2A). The measurement values were represented by the ratio to the center (0 cm).
In addition, the influence of the interval between confronting PACs on contrasts (21) was examined with the aim of grasping the influence of body thickness on contrasts. The phantoms of CuSO4 aqueous solution were set on the center between the anterior and posterior T-PACs, and the PC was measured at intervals of 14, 17, 20, 24, and 27 cm (Figure 2B). The measurement values were represented by the ratio to the value measured at 20 cm.
Acquisition of regression lines with PC
Because the relationship between the molarity and T1-weighted Gd contrast becomes a sigmoid curve at low and medium concentrations (19), it is assumed that this relationship can be approximated to a straight line by logarithmically converting PC. Therefore, the relationship between the PC of the converted device and the PC of the reference device (i.e., rPC) was formulated using the following regression line:
where a0 and b0 were the slope and intercept, respectively.
However, because the Michelson contrast had a negative value in Eq. [3], the antilogarithm in Eq. [5] was aligned to a positive value by adding 1, as shown in the following linear equation:
and
where ap and bp were the slope and intercept, respectively.
To obtain the regression line (i.e., Eq. [6]), PCs were measured at multiple molarities of the Gd-EOB-DTPA phantoms. In sample A, in which only the coil differed from the reference device, the regression line was acquired from T-PAC and BC. In sample B, in which the acquisition parameter and MRI scanner differed from the reference device, the regression line was acquired from T-PAC and SENSE-PAC/Q-BC.
Cross-calibration of the LSC
The PC of Eq. [6] was replaced by LSC, as follows:
where E was the system error between PC and LSC. The r’LSC was the LSC converted into the rLSC by cross-calibration. Eq. [8] was transformed, as follows:
First, to obtain the E of Eq. [8], the regression line of the LSC between T-PAC and BC was acquired from sample A and was compared with the regression line of the PC between T-PAC and BC. Second, the conversion formula (i.e., Eq. [9]) was calculated from the regression line and E. Thirdly, the LSCs of BC, SENSE-PAC, and Q-BC were substituted for the conversion formula, and cross-calibration from LSC to r’LSC was performed in samples A and B. Lastly, each LSC and r’LSC was compared with the rLSC to examine the effect and accuracy of cross-calibration, respectively.
In creating the cross-calibration mentioned above, we referred to the method of standardizing the heart-to-mediastinum ratio in myocardial sympathetic scintigraphy using 123I-metaiodobenzylguanidine (24). The process of cross-calibration is shown in Figure 3.
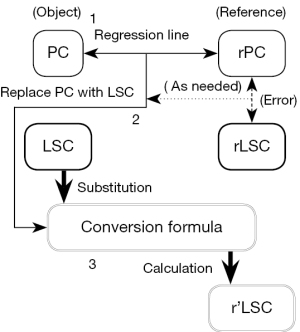
Statistical analysis
For the comparison between subject groups, a student’s t-test was performed assuming equal various between two samples. For the comparison of SI or contrast distribution between T-PAC and BC, analysis of variance (ANOVA) in a two-way layout was performed. The regression line was calculated by linear regression analysis. After carrying out the test of no correlation, Pearson’s correlation coefficient (R), the coefficient of determination (R2), and standard error (SE) were also calculated on all regression analyses. In sample A, the LSC and r’LSC were compared with rLSC using paired t-test and regression analysis. In sample B, LSC and r’LSC were compared with rLSC using analysis of covariance (ANCOVA) with the ALBI scores. ANCOVA was performed to remove any confounding effect of the patient’s liver function; this was because the LSC or r’LSC by SENSE-PAC and Q-BC was not acquired on the same day as the rLSC by T-PAC was acquired. After testing the parallelism of the regression lines between groups and the significance of regression (slope ≠ 0), the difference of the intercepts between groups was tested. ANCOVA was also performed for the comparison of the regression lines of PC and LSC.
In all statistical tests, a two-sided probability (P) value of less than 0.05 was considered to indicate a statistical significance. The 95% confidence interval (CI) was calculated as the interval estimation. All statistical analyses were carried out using the Analysis ToolPak in Excel Add-ins (Microsoft Excel 2010 SP2, Microsoft Corporation, Washington, USA).
Results
Subject groups
After excluding unsuitable cases based on the criteria mentioned above from 1,421 patients who underwent Gd-EOB-DTPA-enhanced MRI, 126 participants (77 men and 49 women) remained. The final numbers of sample A and B were 85 and 41 patients, respectively. The selection of this study population is presented in Figure 4. The patient details and clinical features are shown in Table 2. There was no significant difference in the factors that influenced the images between sample A and B (P>0.05).
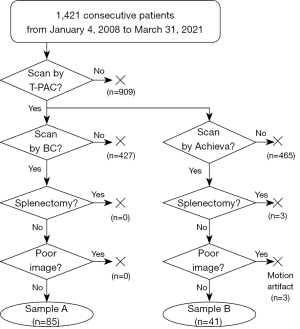
Table 2
Item | Sample A | Sample B | P value |
---|---|---|---|
Sample | |||
Size | 85 | 41 | |
Sex (male/female) (n) | 56/29 | 21/20 | |
Age (years) | 68.7±16.2 (29 to 89) | 68.4±8.7 (52 to 86) | 0.511 |
Physique | |||
Body weight (kg) | 63.4±9.1 (46.0 to 104.0) | 64.4±8.5 (50.0 to 85.0) | 0.576 |
Body thickness (cm) | 22.2±2.2 (16.0 to 29.3) | 22.4±2.1 (16.7 to 26.4) | 0.650 |
Body width (cm) | 30.6±2.2 (24.6 to 36.8) | 30.6±2.3 (25.8 to 35.0) | 0.878 |
Liver disease (n) | |||
Chronic viral hepatitis (type B and/or C) | 16 | 11 | |
Liver cirrhosis (hepatitis B and/or C) | 35 | 19 | |
Alcoholic liver disease | 13 | 7 | |
Nonalcoholic fatty liver disease | 4 | 2 | |
Primary biliary cholangitis | 1 | 0 | |
Normality | 16 | 2 | |
Mass (HCC/metastasis) (n) | 50/6 | 32/0 | |
Liver function test | |||
ALBI score | −2.46±0.57 (−3.30 to −0.92) | −2.55±0.44 (−3.41 to −1.43) | 0.353 |
ALBI grade (Grade 1/Grade 2/Grade 3) (n) | 43/35/7 | 20/21/0 | |
Classic liver function data | |||
Albumin (g/dL) | 3.78±0.60 (2.40 to 4.80) | 3.85±0.51 (2.60 to 4.80) | 0.542 |
Total bilirubin (mg/dL) | 0.95±0.64 (0.20 to 3.90) | 0.78±0.34 (0.30 to 2.00) | 0.114 |
Direct bilirubin (mg/dL) | 0.40±0.48 (0.06 to 3.22) | 0.35±0.45 (0.07 to 3.00) | 0.575 |
All data except those indicated as n represent mean ± standard deviation (minimum to maximum). P, probability; n, number of patients; HCC, hepatocellular carcinoma; ALBI, albumin-bilirubin.
Relaxation times of phantoms
The 2.0 and 5.0 mM/L phantoms of CuSO4 aqueous solution had T1 values of 570 and 331 ms, respectively, and T2 values of 561 and 197 ms, respectively.
The 0.06, 0.14, 0.27, 0.63, 1.37, and 2.82 mM/L phantoms of the Gd-EOB-DTPA aqueous solution had T1 values of 3,400, 1,900, 1,500, 960, 460, and 260 ms, respectively, and T2 values of 1,800, 1,200, 610, 420, 170, and 91 ms, respectively.
Verification of the setting method of two ROIs
The distributions of SI and contrast in the anterior–posterior direction are shown in Figure 5A,5B, respectively. In BC, the distributions of SI and contrast were almost constant, regardless of ROI locations. In T-PAC, the SI increased as it approached the PACs, and the SI that was near the PAC was about twice of that in the center. However, by aligning the height of two ROIs in the anterior–posterior direction, the contrast variation was suppressed to about 10%.
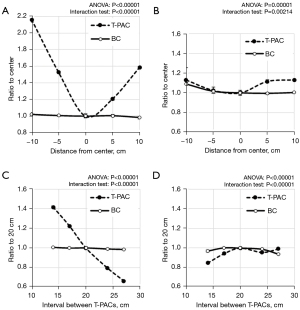
The influences of the interval between confronting PACs on SI and contrast are shown in Figure 5C,5D, respectively. In BC, the SI and contrast were constant regardless of the interval between confronting PACs. In T-PAC, the SI decreased as the interval between confronting PACs increased, and the SI in the interval of 27 cm was half of that in the interval of 14 cm. However, the contrast was constant at an interval of ≥17 cm, which largely covered the body thickness of the subjects measured in this study (Table 2). Even at an interval of 14 cm, the difference in the contrast with BC was about 10%.
Acquisition of regression lines with PC
The relationship between the PC of BC, SENSE-PAC, or Q-BC and the rPC of T-PAC is shown in Figure 6A. In the PC of BC, the regression line with rPC was as follows:
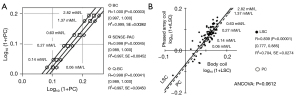
In the PC of SENSE-PAC, the regression line with rPC was as follows:
In the PC of Q-BC, the regression line with rPC was as follows:
The R and R2 values of these three regression lines were both 0.99. Moreover, their SEs were both less than 0.005.
Cross-calibration of the LSC
The relationship between the LSC of BC and the rLSC of T-PAC is shown in Figure 6B. The PCs of up to 1.37 mM/L were included in the range of the LSC. On ANCOVA, the PC tended to be 0.0105 lower than the LSC in the logarithmic contrasts, but no statistically significant difference in the LSC was detected (P=0.0612). Therefore, the E of Eq. [8] was defined as 0.0105.
In the case sample A, the conversion formula was as follows:
On paired t-test, the rLSC significantly differed from the LSC (P<0.00001, 95% CI: 0.0824–0.151) but not from the r’LSC (P=0.492). The effect of cross-calibration in sample A is shown in Figure 7. The regression equation between r’LSC and rLSC after cross-calibration was as follows:
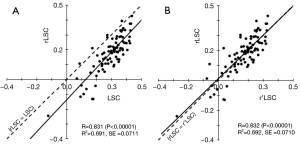
The regression coefficient between r’LSC and rLSC was about 1.0 (P<0.00001, 95% CI: 0.827–1.110). The intercept was not statistically significant (P=0.461). Therefore, this regression equation corresponded with the line of identity (Figure 7B). Before and after cross-calibration, the SEs were both 0.071 and did not change.
The effect of cross-calibration in sample B is shown in Figure 8. In SENSE-PAC, the conversion formula was as follows:
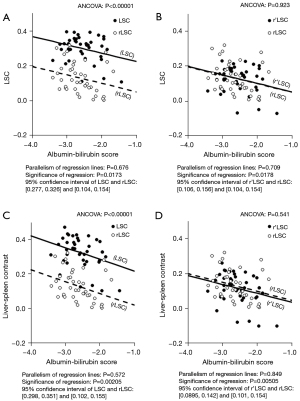
The rLSC significantly differed from the LSC (P<0.00001, 95% CI: 0.138–0.207) (Figure 8A) but not from the r’LSC (P=0.923) (Figure 8B). In Q-BC, the conversion formula was as follows:
The rLSC significantly differed from the LSC (P<0.00001, 95% CI: 0.159–0.234) (Figure 8C) but not from the r’LSC (P=0.541) (Figure 8D). The 95% CI values of the LSC, r’LSC, and rLSC were stable within an interval of 0.05, which did not change before and after cross-calibration.
Discussion
Although a built-in BC that has a uniform SI distribution is an ideal coil to quantitatively measure contrasts, Gd-EOB-DTPA-enhanced MRI is generally performed with PACs to acquire images of high sensitivity and resolution (20). PACs have a concave distribution of SI (21). Therefore, it is necessary to smooth this distribution by an image-based correction technique (e.g., SCIC or homogeneity correction), which adjusts the SIs by predetermined coefficients, or a calibration-based correction technique (e.g., PURE or CLEAR), which uses the reference images of the built-in BC (20,21). However, it had been pointed out that the images rendered by these correction methods cannot be used for quantitative evaluation using contrasts, because these techniques lower the LSC and cannot recover the contrast among organs (20). Our study indicated that the measurement method of aligning the height of two ROIs on an anterior-posterior direction can suppress the variation of contrasts by 10% without correcting the SIs. In addition, within the expected range of body thickness, measurement of the contrasts in uniform distribution was possible, regardless of the positions of the PACs on the anterior-posterior direction (21). The method used in this study entailed acquisition of images before the postprocessing for correction. However, the method of aligning two ROIs can largely contribute to the quantitative measurement of the LSC when correction techniques with a phase image or a B1 map were not built into using MRI scanners. In future, MultiTransmit system should be used, because the MultiTransmit parallel radio frequency transmission technology can improve image nonuniformity and has high contrast, compared with body tuned CLEAR (25).
In this study, the linear model obtained by the logarithmic transformation of the contrast values was proposed to represent the relationship of contrasts between two different devices. In PC, both regression lines indicated strong linearity and high reliability. In the LSC, the relationship between T-PAC and BC showed the same characteristics as that in PC. Therefore, this linear model has validity at a Gd-EOB-DTPA concentration range of up to 2.82 mM/L. For the Gd-EOB-DTPA phantoms, the expected range in the LSC was covered by a concentration range of 0.14 to 1.37 mM/L, except the negative range of the LSC. Additionally, the reference concentrations that simulate normal liver, liver cirrhosis, and tumor were reported to be 0.63, 0.42, and 0.15 mM/L, respectively (26). Accordingly, the line-up of Gd-EOB-DTPA phantoms corresponded to the variations in the LSC and was valid. There was no significant difference between PC and LSC, and the SE values of PC were small. Therefore, as an alternative to the LSC, the phantoms in this study had sufficient accuracy and precision. In the relationship between T-PAC and BC, the regression line with these phantoms was almost identical with the equation of the LSC. Therefore, the regression lines obtained from PCs sufficiently reflected the relationship of the LSCs obtained by different devices.
The conversion formulas based on the regression lines of PC had no error in the two cases of different MRI scanner-coil combinations. In terms of system errors, there was no significant difference between r’LSC and rLSC. For random errors, there was no change in the SE or 95% CI values before and after conversion. These results supported the high accuracy and precision of this conversion formula. This conversion formula can correct the system errors of the phantoms. In addition, even if the acquisition parameters of the MRI scanners changed before and after conversion, there was no significant difference between the r’LSCs of SENSE-PAC/Q-BC and the rLSC of T-PAC. Therefore, cross-calibration had high accuracy and utility.
Our study had the limitation of using the unconsidered phantoms about human equivalent electrical conductivity. The phantoms for dilution with purified water had higher T2 values, compared with that of abdominal living tissues (27). However, the effects of high T2 values appear to be limited because Gd-EOB-DTPA-enhanced MRI is acquired by minimum echo and repetition times (26). In fact, the difference between PC and the LSC did not significantly differ. If high accuracy of the phantoms is required, the high T2 values could be improved by dilution with normal saline at 0.9 w/v% (28) and the size of phantoms had better be about a human body to heighten uniformity. Another limitation of our study was that the only three cases of different MRI scanner-coil combinations were compared. Nevertheless, as a pilot study, our findings sufficiently provided clear evidence that our method of cross-calibration of the LSC had high accuracy and universality. Future multicenter studies on cross-calibration are needed to standardize the LSC if the LSC becomes an almost complete substitute for traditional liver function indices with sufficient evidence.
Conclusions
We proposed a method of converting the LSC to rLSC acquired by another device using conversion formulas obtained from PC. Cross-calibration using the regression lines of PC had high accuracy and utility and is sure to contribute to the standardization of the LSC.
Acknowledgments
Funding: None.
Footnote
Reporting Checklist: The authors have completed the STROBE reporting checklist. Available at https://qims.amegroups.com/article/view/10.21037/qims-22-174/rc
Conflicts of Interest: Both authors have completed the ICMJE uniform disclosure form (available at https://qims.amegroups.com/article/view/10.21037/qims-22-174/coif). The authors have no conflicts of interest to declare.
Ethical Statement: The authors are accountable for all aspects of the work in ensuring that questions related to the accuracy or integrity of any part of the work are appropriately investigated and resolved. The study was conducted in accordance with the Declaration of Helsinki (as revised in 2013). The study was approved by the ethics review board of the Japan Community Healthcare Organization Hokkaido Hospital (Research Reference No. 2018-21). Written individual consent for this retrospective analysis was waived.
Open Access Statement: This is an Open Access article distributed in accordance with the Creative Commons Attribution-NonCommercial-NoDerivs 4.0 International License (CC BY-NC-ND 4.0), which permits the non-commercial replication and distribution of the article with the strict proviso that no changes or edits are made and the original work is properly cited (including links to both the formal publication through the relevant DOI and the license). See: https://creativecommons.org/licenses/by-nc-nd/4.0/.
References
- Xie S, Liu C, Yu Z, Ren T, Hou J, Chen L, Huang L, Cheng Y, Ji Q, Yin J, Zhang L, Shen W. One-stop-shop preoperative evaluation for living liver donors with gadoxetic acid disodium-enhanced magnetic resonance imaging: efficiency and additional benefit. Clin Transplant 2015;29:1164-72. [Crossref] [PubMed]
- Bae KE, Kim SY, Lee SS, Kim KW, Won HJ, Shin YM, Kim PN, Lee MG. Assessment of hepatic function with Gd-EOB-DTPA-enhanced hepatic MRI. Dig Dis 2012;30:617-22. [Crossref] [PubMed]
- Katsube T, Okada M, Kumano S, Hori M, Imaoka I, Ishii K, Kudo M, Kitagaki H, Murakami T. Estimation of liver function using T1 mapping on Gd-EOB-DTPA-enhanced magnetic resonance imaging. Invest Radiol 2011;46:277-83. [Crossref] [PubMed]
- Nilsson H, Blomqvist L, Douglas L, Nordell A, Jonas E. Assessment of liver function in primary biliary cirrhosis using Gd-EOB-DTPA-enhanced liver MRI. HPB (Oxford) 2010;12:567-76. [Crossref] [PubMed]
- Takao H, Akai H, Tajima T, Kiryu S, Watanabe Y, Imamura H, Akahane M, Yoshioka N, Kokudo N, Ohtomo K. MR imaging of the biliary tract with Gd-EOB-DTPA: effect of liver function on signal intensity. Eur J Radiol 2011;77:325-9. [Crossref] [PubMed]
- Motosugi U, Ichikawa T, Sou H, Sano K, Tominaga L, Kitamura T, Araki T. Liver parenchymal enhancement of hepatocyte-phase images in Gd-EOB-DTPA-enhanced MR imaging: which biological markers of the liver function affect the enhancement? J Magn Reson Imaging 2009;30:1042-6. [Crossref] [PubMed]
- Mori H, Furuya K, Akimoto S, Ajioka R, Emoto T. Examination of the means of measuring liver function in the hepatobiliary phase. Nihon Hoshasen Gijutsu Gakkai Zasshi 2009;65:1502-11. [Crossref] [PubMed]
- Norén B, Forsgren MF, Dahlqvist Leinhard O, Dahlström N, Kihlberg J, Romu T, Kechagias S, Almer S, Smedby Ö, Lundberg P. Separation of advanced from mild hepatic fibrosis by quantification of the hepatobiliary uptake of Gd-EOB-DTPA. Eur Radiol 2013;23:174-81. [Crossref] [PubMed]
- Norén B, Dahlström N, Forsgren MF, Dahlqvist Leinhard O, Kechagias S, Almer S, Wirell S, Smedby Ö, Lundberg P. Visual assessment of biliary excretion of Gd-EOB-DTPA in patients with suspected diffuse liver disease - A biopsy-verified prospective study. Eur J Radiol Open 2015;2:19-25. [Crossref] [PubMed]
- Onoda M, Hyodo T, Murakami T, Okada M, Uto T, Hori M, Miyati T. Optimizing signal intensity correction during evaluation of hepatic parenchymal enhancement on gadoxetate disodium-enhanced MRI: comparison of three methods. Eur J Radiol 2015;84:339-45. [Crossref] [PubMed]
- Ogura A, Miyati T, Kobayashi M, Imai H, Shimizu K, Tsuchihashi T, Doi T, Machida Y. Method of SNR determination using clinical images. Nihon Hoshasen Gijutsu Gakkai Zasshi 2007;63:1099-104. [Crossref] [PubMed]
- Tokorodani R, Kume T, Daikoku K, Oka M. Evaluation of the Validity of ROI Setting in CEI Used for the Assessment of Liver. Nihon Hoshasen Gijutsu Gakkai Zasshi 2022;78:44-52. [Crossref] [PubMed]
- Mori H, Machimura H, Iwaya A, Baba M, Furuya K. Comparison of liver scintigraphy and the liver-spleen contrast in Gd-EOB-DTPA-enhanced MRI on liver function tests. Sci Rep 2021;11:22472. [Crossref] [PubMed]
- Dahlqvist Leinhard O, Dahlström N, Kihlberg J, Sandström P, Brismar TB, Smedby O, Lundberg P. Quantifying differences in hepatic uptake of the liver specific contrast agents Gd-EOB-DTPA and Gd-BOPTA: a pilot study. Eur Radiol 2012;22:642-53. [Crossref] [PubMed]
- Yamada A, Hara T, Li F, Fujinaga Y, Ueda K, Kadoya M, Doi K. Quantitative evaluation of liver function with use of gadoxetate disodium-enhanced MR imaging. Radiology 2011;260:727-33. [Crossref] [PubMed]
- Okubo H, Mogami M, Ozaki Y, Igusa Y, Aoyama T, Amano M, Kokubu S, Miyazaki A, Watanabe S. Liver function test by gadolinium-ethoxybenzyl-diethylenetriamine pentaacetic acid-enhanced magnetic resonance imaging with consideration of intrahepatic regional differences. Hepatogastroenterology 2013;60:1547-51. [PubMed]
- Naka T, Takahashi M, Takeda K, Igarashi T, Hondera T. Optimization of T1-weighted Imaging Parameters in MR Carotid Plaque Imaging: Influence for the Quantitative Evaluation among Different MRI Scanner Types. Nihon Hoshasen Gijutsu Gakkai Zasshi 2017;73:104-11. [Crossref] [PubMed]
- Hiraoka A, Michitaka K, Kumada T, Izumi N, Kadoya M, Kokudo N, Kubo S, Matsuyama Y, Nakashima O, Sakamoto M, Takayama T, Kokudo T, Kashiwabara K, Kudo M. Validation and Potential of Albumin-Bilirubin Grade and Prognostication in a Nationwide Survey of 46,681 Hepatocellular Carcinoma Patients in Japan: The Need for a More Detailed Evaluation of Hepatic Function. Liver Cancer 2017;6:325-36. [Crossref] [PubMed]
- Kumashiro M, Dohke M, Nakada K. Difference of T1-weighted contrast among various pulse sequences: basic study by using a phantom containing Gd-DTPA solutions. Jpn J Magn Reson Med 1999;19:528-38. (JJMRM).
- Ogasawara G, Inoue Y, Matsunaga K, Fujii K, Hata H, Takato Y. Image Non-Uniformity Correction for 3-T Gd-EOB-DTPA-Enhanced MR Imaging of the Liver. Magn Reson Med Sci 2017;16:115-22. [Crossref] [PubMed]
- Kimura T, Tahara D, Iiduka A, Taniguchi Y, Ishikuro A, Hongo T, Inoue H, Ogura A. Examination of measurement and its method of compensation of the sensitivity distribution using phased array coil for body scan. Nihon Hoshasen Gijutsu Gakkai Zasshi 2003;59:1164-73. [Crossref] [PubMed]
- Michelson AA. Studies in optics. Chicago: Chicago Press; 1927.
- Peli E. Contrast in complex images. J Opt Soc Am A 1990;7:2032-40. [Crossref] [PubMed]
- Nakajima K, Okuda K, Matsuo S, Yoshita M, Taki J, Yamada M, Kinuya S. Standardization of metaiodobenzylguanidine heart to mediastinum ratio using a calibration phantom: effects of correction on normal databases and a multicentre study. Eur J Nucl Med Mol Imaging 2012;39:113-9. [Crossref] [PubMed]
- Shibukawa S, Horie T, Nishio H, Muro I. Basic examination of uniformity of image and contrast of multi-transmit system. Nihon Hoshasen Gijutsu Gakkai Zasshi 2011;67:1192-9. [Crossref] [PubMed]
- Oura D, Abe K, Nambu T, Kondo Y. Basic evaluation of the optimal flip angle for hepatocyte phase with gadolinium ethoxybenzyl diethylenetriamine pantaacetic acid: selection of the optimal flip angle by the imaging findings. Nihon Hoshasen Gijutsu Gakkai Zasshi 2013;69:1394-404. [Crossref] [PubMed]
- de Bazelaire CM, Duhamel GD, Rofsky NM, Alsop DC. MR imaging relaxation times of abdominal and pelvic tissues measured in vivo at 3.0 T: preliminary results. Radiology 2004;230:652-9. [Crossref] [PubMed]
- Murakami K, Yoshida K, Kakuba K, Yanagimoto S. Contrast-to-noise ratio for liver parenchyma of the large arterial blood maximum density of Gd-EOB-DTPA and Gd-DTPA in first pass dynamic study (3D-FSPGR technique). Nihon Hoshasen Gijutsu Gakkai Zasshi 2009;65:301-5. [Crossref] [PubMed]