Intraoperative identification of pulmonary nodules during minimally invasive thoracic surgery: a narrative review
Introduction
Computed tomography (CT) is widely used in the screening and follow-up of lung disease; as a result, the detection rate of pulmonary nodules has increased. The annual incidence of pulmonary nodules in the United States is expected to rise with the current screening guidelines and advances in imaging technology, and has been reported to be 1.5 million (1). The nodules detected by CT can be single or multiple, solid or subsolid, and subsolid nodules can further be divided into ground-glass nodules (GGNs) and partially solid nodules. More importantly, when pulmonary GGNs continue to increase in size for more than 3 months, and are bigger than 10 mm in diameter, the probability of malignancy can range from 10% to 50% (2). The American College of Chest Physicians (ACCP) recommends surgical lung biopsy for indeterminate nodules that are >8 mm in diameter, are hypermetabolic or functional imaging tests positive, have a high malignant probability, when a non-surgical biopsy is suspicious for malignancy, or when a fully informed patient selects a definitive diagnostic procedure (3). Currently, minimally invasive thoracic surgery (MITS), including video-assisted thoracoscopic surgery (VATS) and robotic-assisted thoracic surgery (RATS), is the preferred method of surgery for patients with pulmonary nodules due to its advantages of minimal invasiveness and rapid recovery. However, when subsolid or GGNs are >15 mm in diameter and >10 mm from the pleura, the failure rate of intraoperative nodule identification is extremely high and conversion to thoracotomy is required (4). The current methods for assisting the localization of pulmonary nodules include CT-guided percutaneous placement of markers, bronchoscopy placement of markers, intraoperative ultrasound (US), three-dimensional (3D) printing, artificial intelligence (AI)-assisted identification, and intraoperative molecular imaging (IMI). Some studies have shown no differences in localization success among solid, subsolid, and pure ground glass lesions using hook wire, microcoil, and blue dye (5-8). This paper reviews the most commonly used intraoperative techniques for the localization of pulmonary nodules undergoing MITS. We present the following article in accordance with the Narrative Review reporting checklist (available at https://qims.amegroups.com/article/view/10.21037/qims-22-309/rc).
Methods
Literature published in the Cochrane Library, PubMed, ClinicalTrials, and China National Knowledge Infrastructure from 1990 to 2022 were searched to obtain a comprehensive review of the different marker placement methods used for pulmonary nodules. Literature related to animal experiments were excluded. The following search terms were used: lung neoplasms, pulmonary/lung nodule, peripheral lung lesion, ground-glass opacities, preoperative marking, intraoperative marking, thoracoscopic surgery, video-assisted thoracoscopic surgery, hookwire, microcoils, hydrogel plug, methylene blue, lipiodol, barium, fluorescence tracer, intraoperative ultrasonography, transthoracic, bronchoscopy, virtual bronchoscopy, electromagnetic bronchoscopy, 3D printing, artificial intelligence, and intraoperative molecular imaging (Table 1).
Table 1
Items | Specification |
---|---|
Date of search (specified to date, month, and year) | 10/03/2022 |
Databases and other sources searched | Cochrane Library, PubMed, ClinicalTrials, and China National Knowledge Infrastructure |
Search terms used (including MeSH and free text search terms and filters) | MeSH: lung neoplasms Free text search terms: pulmonary/lung nodule, peripheral lung lesion, ground-glass opacities, preoperative marking, intraoperative marking, thoracoscopic surgery, video-assisted thoracoscopic surgery, Hookwire, microcoils, hydrogel plug, methylene blue, lipiodol, barium, radionuclide, fluorescence tracer, intraoperative ultrasonography, transthoracic, bronchoscopy, virtual bronchoscopy, electromagnetic bronchoscopy, 3D printing, artificial intelligence, and intraoperative molecular imaging. Filters: Human, Full text, Clinical Trial, Randomized Controlled Trial, and Meta-Analysis |
Timeframe | 1 January 1990 to 10 March 2022 |
Inclusion and exclusion criteria (study type, language restriction, etc.) | Inclusion: clinical trials, retrospective study, case reports. Exclusion: animal testing. Literature published in any language were included |
Selection process (who conducted the selection, whether it was conducted independently, how consensus was obtained, etc.) | A comprehensive literature search was independently conducted by two authors (Lu Tang and Yiheng Zhang) to identify the relevant published studies |
Any additional considerations, if applicable | None |
MeSH, Medical Subject Headings.
CT-guided percutaneous placement of markers
CT-guided percutaneous solid markers
Hookwire
After long-term practice, the percutaneous situation of localizers is viewed as a generally safe and effective method (9). At present, the most widely used method in clinical practice is CT-guided hookwire placement (10), which was first reported by Mack in 1992 (11). Hookwire is especially effective when the node is superficial to the lung. The process is based on CT-guided lung biopsy, and thus, it is associated with similar complications to CT-guided lung biopsies. A common complication is pulmonary hemorrhage, with a reported incidence of 31.38% (342/1,090), and includes lower-grade (24.4%, 266/1,090) and higher-grade hemorrhage (6.97%, 76/1,090) (12). The most fatal complication is air embolism (13). However, CT-guided hookwire placement also has its drawbacks, such as pneumothorax, hemothorax, subcutaneous emphysema, and wire shedding (14,15), in addition to life-threatening complications caused by air embolism, such as cerebral infarction, epilepsy, temporary and permanent paralysis, shock, and cardiac arrest (14,16). In 2002, Japanese researchers conducted a Hookwire marking and auxiliary suture system in 168 lesions of 150 patients and observed complications of asymptomatic pneumothorax in 54 cases (32.1%), intrapulmonary hemorrhage in 25 cases (14.9%), and hemothorax in 1 case (0.6%). The failure marking of 4 patients was due to superficial puncture, and none of the participants experienced major complications (17). Iguchi et al. also reported that 2 in 500 patients experienced retained Hookwires. One of the cases was due to the Hookwire being placed at a considerable distance from the target and the other was due to a wedge resection performed from the interlobar fissure side (18). Other studies and reviews have shown similar rates of relatively minor complications (10,19-23). However, this method has been banned in Japan due to the possibility of death due to air embolism (24).
Microcoils
Another widely used CT-guided intraoperative implanted is the microcoil. Mayo et al. reported the safety and efficacy of VATS guided by microcoils for the resection of 75 pulmonary nodules (4–24 mm). In their study, the microcoil displacement complication rate was 3%, and the diagnostic rate of fluoroscopy-guided resection was 97% (25). In a randomized trial, Finley et al. reported that microcoil localization of pulmonary nodules less than 15 mm in diameter had a success rate of 93% (27/29), without associated complications (26). The conversion rates for thoracotomy due to failure of nodule identification during VATS ranged from 0 to 6.6% (25,27). Small needle-pleura angle (≤30°), pleura-microcoil distance (≤20 mm), and the presence of pleural indentation during the procedure were identified as significant risk factors contributing to microcoil pleura marking failure (28). Compared to Hookwire, microcoil localization has a corresponding successful operative field targeting rate of 97% to 94%, a successful VATS rate of 97% to 96%, and a lower complication rate (9,29). Xu et al. reported microcoil localization in 47 patients (48 pulmonary nodules) covered by the scapulae, and the rates of successful targeting and localization were 95.8% (46/48) and 89.6% (43/48), respectively (30). The most common complications were asymptomatic pneumothorax (4–13%) and microcoil displacement (0–5%) (26,31) .
Hydrogel
To overcome complications such as pneumothorax caused by localizers, cylindrical hydrogel plugs have been proposed for intraoperative marking of pulmonary nodules (32-34). In this method, after CT-guidance of the lung tissue puncture, the dry hydrogel is used to enter the lung nodule. When the delivery system with the guide needle is withdrawn from the chest wall, the dry hydrogel is rapidly hydrated due to the contact with the parenchyma nearby. The needle tract is sealed, and the expanded hydrogel protrudes from the parenchymal pleura and marks the location of the inferior nodule. Imperatori et al. recently reported the use of this method to identify 28 pulmonary nodules, with 89% (25/27) of the nodules detected by hydrogel plug localization, and the other 11% discovered by palpation or pleural puncture displaced. Only 1 in 28 cases (4%) required drainage due to pneumothorax during hydrogel plug labeling, and no other complications were reported (35). Qian et al. also reported similar results using medical glue to locate 15 pulmonary nodules: 1 case of pneumothorax, 5 cases of chest pain, and 6 cases of cough were reported (36). Wang et al. used blue-stained glue in 20 patients with a 100% success rate for intraoperative localization, and 9 cases of pneumothorax and 4 cases of minor pulmonary hematoma were detected (37).
In addition to research innovations in implants, recently, cone beam CT (CBCT)-guided percutaneous approaches or DynaCT combined with intraoperative CT in the hybrid operating room (HOR) in the lung have been reported. In cases of simultaneous localization of difficult lesions, advantages such as less discomfort, fewer complications, and lower VATS conversion rates have been reported (38-40).
CT-guided percutaneous injection of liquid marker
Methylene blue (MB)
MB is one of the most commonly used dyes in clinical practice, and MB localization is a relatively safe and inexpensive method. The procedure is well tolerated by the patient as there is no foreign body implantation. The thoracoscopic identification rate of pulmonary nodules after MB localization is 90–100% (41,42). However, an important disadvantage of MB labeling is that the dye diffuses into the surrounding lung parenchyma, preventing accurate identification of pulmonary nodules (10), with a failure rate of up to 8% (43). Furthermore, in rare cases, MB can cause allergic reactions (44). In cases such as when anthrax hyperpigmentation is present in the lung parenchyma, the dye may not be distinguishable from the surrounding lung during thoracoscopy (41,44). Some researchers have suggested that VATS should be started within 120–150 minutes after localization to reduce the spread of MB to the surrounding tissues (43). To prevent this shortcoming of rapid diffusion, many researchers have developed improved methods, such as MB-stained autologous blood (45), MB mixed with contrast agents and collagen (46), and MB-stained glue (37). The same dye localization method also uses patent blue V (PBV) dye (47,48) and agar (49).
Lipiodol and barium
Lipiodol injection is another widely accepted localization method. Mogi et al. used CT-guided lipiodol to mark 56 patients during VATS. The candidates for lipiodol marking had lesions ≤10 mm in diameter and/or with a distance to the nearest pleural surface of >10 mm that were also localized in the outer third of the lung parenchyma. A total of 55/56 patients (98.2%) successfully underwent the resection (50). With regards to complications, pneumothorax, hemorrhaging, chest pain, blood in sputum, and pneumothorax were noted (50-52). Barium is another imaging agent for the localization of pulmonary nodules. Lee et al. reviewed 10 patients with pulmonary nodules who underwent CT-guided barium sulfate preoperative localization. Postoperative CT scans showed that the localization success rate was 100%. Besides 2 cases of pneumothorax after the operation, no other complications were detected (53). However, the barium suspension forms round or oval barium balls in the lung tissue, and the density is very different from that of lung tissues, which can cause a severe acute inflammatory reaction and even pulmonary edema or pulmonary fibrosis, and thus, should be used with caution (53).
Radionuclide
Radionuclide injection under CT guidance is mainly based on the injection of 99mTc-labeled human serum albumin at the lung nodules, followed by intraoperative probe detection of gamma rays converted into digital counts and audio signals (54). The advantage of radiotracers is that they remain in the body for more than 24 hours, can be well tolerated by patients, and can detect deep nodules. However, its disadvantage is that it is facility-dependent, requires special equipment (radiotracer, gamma probe, and radiation protection equipment), and increases radiation exposure. Ambrogi et al. used this method on 211 patients with a success rate of 98.6% (208/211). Only 1 case of pneumothorax during the labeling procedure was detected, where some radio- tracer had expanded into the pleural space, which suggested that this method is safe and effective (55).
Indocyanine green (ICG)
Currently, CT-guided localization using the fluorescent tracer ICG is popular due to its clear visualization (56). Zhang et al. reported the use of CT-guided percutaneous injection of ICG to localize 35 patients, with a success rate of 94.3% (33/35). No severe adverse reactions were found during the procedure (57). Although ICG can realize the detection of pulmonary nodules, the method has certain limitations, including the requirement for fluoroscopy equipment to detect ICG fluorescence. In addition, the diffuse or insufficient injection of ICG may seriously affect the ability of the operator to locate the nodule.
CT-guided percutaneous dual localization
Some markers can be combined for clinical applications. For example, Zhang et al. performed CT-guided labeling of 153 pulmonary nodules in 140 patients which were smaller than 2 cm with a mixed method of tissue adhesive and iohexol (58); Jiang et al. used medical glue and MB to mark 383 nodules in 346 patients (59); Doo et al. used Hookwire and 99mTc to mark 36 nodules in 34 patients (60); Wang et al. used 99mTc and MB to label a patient successfully (61); and Brady et al. localized 75 nodules with Hookwire combined with MB injection in 74 patients (62). Although these combined applications have also been associated with complications, it is presumed that they can also effectively improve the overall surgical recognition rate.
Bronchoscopy-guided placement of markers
An alternative to the percutaneously-mediated approach is the bronchoscopy approach. The latter appears to be an improvement in terms of complications such as pneumothorax and hemothorax. Furthermore, percutaneous CT marking can be complicated by air embolisms, yet there have been no reports of air embolism caused by bronchoscopy. In addition, bronchoscopy can be used in areas that are not accessible by percutaneous methods, including the interlobar fissures and areas facing the mediastinum, diaphragm, and scapula. Bronchoscopy labeling methods including instillation of barium mixtures (63-65), blue or fluorescent dyes (66-71), and placement of microcoils (72,73), all of which have a similar success rate as CT-guided percutaneous labeling. The implementation of these techniques is performed under CT fluoroscopy or virtual bronchoscopy.
Electromagnetic navigation bronchoscopy (ENB)
ENB is a technique proposed in recent years for the evaluation of small peripheral lung lesions. It combines virtual and conventional bronchoscopy with the aim of guiding the diagnosis and/or localization of pulmonary nodules with dye-labeled instruments. The ENB diagnosis rate of pulmonary nodules can reach 90–100% (67,74-76). Compared with other percutaneous markers, ENB-guided dye labeling for intraoperative VATS localization is safe and has fewer complications (70,75-78). A meta-analysis of 15 studies, including 681 procedures, demonstrated that diagnostic ENB is safe: 40 cases of pneumothorax, 7 cases of minor or moderate bleeding, and 2 cases of post-procedural respiratory failure were reported, with none requiring specific treatment (79).
Virtual-assisted lung mapping
The 3D localization of lung nodules is represented by virtual assisted lung mapping (VAL-MAP), which traditionally provides multiple markers on the lung, as well as geometric information, or X, Y, and/or Z axes on the lung surface. Importantly, multi-label two-dimensional (2D) or 3D information replaces single-dimensional localization that may cause inaccurate incisal margins, and CT repositioning after VAL-MAP ensures accurate or reproducible information. The Japanese researcher Sato published a series of reports on VAL-MAP labeling, with the methodology changing from 2D to multi-dimensional, from MB to visualization in dye, and from traditional CT to CBCT (24,74,80-84). Furthermore, VAL-MAP was associated with a low risk of pneumothorax (6.6% per case; 2.1% per mark), with no hemothorax (80).
Similarly, some cases of bronchoscopy combined with intraoperative use of cone-beam CT or ENB have been successfully performed, with bronchoscopy dye labeling of small pulmonary nodules (38,66).
Intraoperative ultrasonography
US technology has been used to locate pulmonary nodules since the 1990s. It has the advantage of being safe and effective in locating unpalpable nodules in real time without causing damage to the lung parenchyma, thus eliminating the risk of pneumothorax and hemoptysis. However, it has certain limitations. For example, to avoid artifacts, lung US requires complete deflation of the lungs, which is more difficult in patients with emphysema. Ground-glass opacities (GGOs) are difficult to identify by US due to their density being close to that of normal lung parenchyma. However, the study by Kondo et al. showed that experienced clinicians can safely and effectively localize these nonpalpable nodules by intraoperative US (85). Other studies have confirmed that the success rate of intraoperative US identification of pulmonary nodules can reach 93–98% (86-89). An US provides access to most of the visceral pleural surface. During VATS, US can almost completely explore the visceral pleura, and a 1 cm length US probe can enter areas of the lung that cannot be reached by finger palpation. In addition, the US can be positioned to compensate for the failure of intraoperative CT-guided marking.
Lachkar et al. reported the use of radial endobronchial ultrasound (r-EBUS) for virtual bronchoscopy of endoscopic pleural dye-labeled biopsy of pulmonary nodules (90). Ost et al. conducted a multicenter clinical study and found a diagnostic rate of 63.7% with either r-EBUS nor ENB, 57.0% with r-EBUS alone, 38.5% with ENB alone, and 47.1% with both ENB and r-EBUS. These results differed significantly from expectations, and prospective studies are warranted to further assess the efficacy and cost/benefit ratio of these modalities (91).
3D printing technology
Currently, 3D printing is an evolving manufacturing technology. When percutaneous placement of markers is used in combination with preoperative 3D-assisted printing and localization technology, it can effectively reduce unnecessary radiation exposure that occurs during conventional localization process. A study by Fu et al. involving 37 patients used CT scans to build models, and 3D printed models were then used for intraoperative percutaneous localization to help identify small lung nodules. After a learning curve, the localization success rate was as high as 95.6% (92). Zhang et al. randomized 190 patients into the groups in a 1:1 ratio and compared percutaneous localization with 3D template and CT-guided percutaneous localization. There was no deviation between the two groups of localizers, with patients in the 3D template group showing better localization time and less radiation dose (93).
AI
In this golden age of rapid advances in AI, researchers and surgeons are realizing that AI can contribute to every aspect of healthcare, especially surgery. In terms of pulmonary nodule localization, the use of CT-guided 3D reconstruction, virtual reality (VR), augmented reality (AR), and mixed reality (MR) have all be explored.
The VR technology can assist in the generation of immersive, completely artificial computer-simulated images and environments that allow for real-time interaction. Jensen et al. developed a VATS lobectomy assessment tool (VATSAT) which provides supervisors and assessors with a procedure-specific assessment tool for evaluating VATS lobectomy performance and helps to determine when a trainee is ready to work unsupervised (94). Ujiie et al. developed a VR surgical navigation system using head mounted displays (HMD) to improve preoperative planning and contribute to the safety and accuracy of anatomic resection (95). Qin et al. developed a training system (VatsSim-XR) which includes customized haptic-enabled thoracoscopic instruments, a VR helmet set, endoscope kit with navigation, and a patient-specific corresponding training environment (96).
The AR technology superimposes computer-generated imagery onto a real-life view. Peng et al. constructed a model using pigs and selected 30 native lung structures to simulate solitary lung nodules. Using the Microsoft HoloLens AR system (Microsoft Corp., Redmond, WA, USA), the points picked in the model could be accurately marked. The results showed an average success rate of 76.67% within a diameter of 1 cm around nodules, and 100% within a diameter of 2 cm around nodules. Therefore, HoloLens AR-assisted pulmonary nodule localization may be a promising technique to improve the surgical treatment of early-stage lung cancer (97).
Combining AR and VR gives rise to MR, where digital and physical objects interact in real-time. Perkins developed a software application and medical image processing pipeline for the Microsoft HoloLens to incorporate patient-specific data and provide a mixed-reality tool to explore and manipulate chest anatomy with a custom-designed user interface featuring gesture and voice recognition. This may facilitate the accurate and rapid identification of small lung lesions during minimally invasive surgeries and reduce the need for additional invasive preoperative localization procedures (98).
Although AI technology is still in the initial stages of research, it has broad prospects. For example, the simplification of operations is conducive to the training of young doctors. However, there are also many challenges, such as the diversification of surgical operations, the inability to achieve the unity of computer simulation, and some anatomical variations that may lead to errors in AI recognition. The effective integration of AI with thoracic surgery remains a challenging research topic.
IMI
Currently, IMI emerging as a new technique that uses targeted optical contrast agents in combination with imaging devices during surgery to identify resected lung cancer tissue. The commonly used imaging systems include Artemis (the Netherlands), PINPOINT (Canada), Firefly (USA), Karl Atorz Photodynamic Diagnostics (PPD; Germany), and DPM (China). Most of the research in this area has been performed by Professor Singhal of the University of Pennsylvania. Okusanya et al. first tried to locate pulmonary nodules by intravenous administration of ICG in 2014. They administered 5 mg/kg of ICG 24 hours before surgery and successfully identified the 16 pulmonary nodules in 18 patients, and an additional 5 sub-centimeter nodules were found, making this the first in-human trial using near-infrared (NIR) imaging to identify pulmonary nodules during thoracic surgery (99). Kim et al. demonstrated that ICG can locate pulmonary nodules in resected lung specimens at a dose of 1 mg/kg; just 2 false positives were seen in patients with no residual tumor after neoadjuvant treatment (100). Mao et al. also labelled 36 patients with intravenous administration of ICG. In 36 patients, 76 nodules were resected, and 68 nodules were found during in vivo exploration by ICG fluorescence imaging (101). Researchers have also applied ICG intravenous injection to detect lesions in patients with sarcoma lung metastases, and to identify colon cancer lung metastases during surgery, with some occult lesions detected in their studies (102,103). In addition to systemic administration, Quan et al. tested 6 patients through respiratory tract administration of ICG, and the results showed that the fluorescence mainly localized in non-cancerous tissues, and the edge of the tumor could be clearly displayed, which represents a method of reverse exploration (104).
Due to the limitations of ICG with poor specificity, including accumulation in areas of inflammation, researchers have sought a targeted molecular imaging agent that can specifically bind to and identify lung adenocarcinomas (LUADs). The folate receptor (FR) is a highly expressed target in LUAD. There are 4 members in the FR family, but only FRα and FRβ bind folic acid with high affinity. Approximately 80–90% of LUADs express apical luminal FRα (1–3 million receptors per cancer cell), and thus, bind greater amounts of serum folate than normal lung epithelial cells (105-107). Therefore, FRα may be a potential molecular target for the diagnosis of LUAD. Keating et al. designed a compound named EC17 (C42H34N10Na2O10S), which is produced by binding folic acid hapten (vitamin B9) and fluorescein isothiocyanate (FITC) through an ethylenediamine spacer. Patients were administered 0.1 mg/kg EC17 dissolved in 10 mL of normal saline 2 hours before surgery via peripheral vein injection lasting 10 minutes. Fluorescence imaging during surgery revealed that the lung nodules of all 3 patients showed green fluorescence, with no adverse reactions (108). Predina et al. conducted further studies on the imaging conditions. Due to the non-specific accumulation of contrast agents in non-cancerous tissues, lung parenchyma, granulomas, edema tissues, and others, the fluorescence signals may be similar to those of some tissues which have biological chromophores, such as hemoglobin, collagen, and porphyrin. The tumor-to-background ratio (TBR) can be improved by modifying the exposure time on the imaging capture device (109). Okusanya et al. reported using EC17 4 hours before surgery in 50 patients, with 92% sensitivity for pulmonary adenocarcinomas. Only 14% of nodules were identified with intraoperative fluorescence imaging (IFI) in vivo (105). Kennedy et al. also applied EC17 4 hours before surgery and achieved 100% specificity for pulmonary adenocarcinomas. These results indicated that optical biopsy may be more accurate than frozen section for the identification of pulmonary adenocarcinomas (110).
Since the success rate of nodules targeted by FITC is related to the distance from the pleura, the team developed another material, OTL38. OTL38 is a folate analog conjugated to the NIR dye S0456 with excitation and emission maxima at 776 and 796 nm, respectively. Due to light scattering reduction and reduced blood absorption, the depth of penetration into solid organs increases and autofluorescence decreases (111). Keating et al. first studied OTL38 in cells, followed by animal and human experiments. After intravenous injection of 0.025 mg/kg OTL38 2 hours before surgery, fluorescence was detected in all 3 lung nodules. The results of this study support the use of NIR thorascopic surgery with an appropriately targeted NIR contrast agent for the investigation of early stage non-small cell lung cancer to delineate tumor margins and identify metastatic lymph nodes (111). Predina et al. conducted a series of clinical trials and demonstrated that OTL38 has a sensitivity of 69.2–100% for the in vivo localization of pulmonary nodules in FR-positive patients, and the depth of pulmonary nodules from pleura can reach 2 cm (112-114). The mechanism is shown in Figure 1.
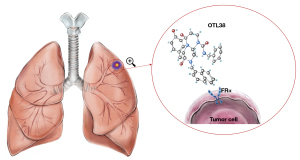
In summary, NIR imaging has several unique advantages compared with other techniques, including no requirement for radiation, better imaging through organs, no requirement for advance knowledge regarding the location of the nodule, ease of interpretation, real-time, and intuitive use for the observer. Therefore, this technology will likely become the treatment trend in the future. A summary of the clinical trials examining IFI for pulmonary nodules can be found in Table 2.
Table 2
Principle investigator | Year | Disease | Surgical approach | Drug | Dose | Method | Time from injection to imaging | Imaging system | No. of patients | No. of nodules | Sensitivity for malignancy | Size of the smallest nodules detected | Maximum depth of detected nodules | TBR (range) | SUVmax |
---|---|---|---|---|---|---|---|---|---|---|---|---|---|---|---|
Okusanya (99) | 2014 | Lung cancer | Thoracotomy | ICG | 5 mg/kg | Systemic injection | 24 h | BioVision | 18 | 23 | 95.5% (21/22) | 0.2 cm | 1.3 cm | 1.5–4.4 | 0–11 |
Kim (100) | 2016 | Lung cancer | VATS | ICG | 1 mg/kg | Systemic injection | 24 h | PINPOINT | 11 | 11 | 88.9% (8/9) | 0.3 cm | 1.4 cm | NR | 0.8–9.6 |
Mao (101) | 2017 | Lung cancer | VATS | ICG | 5 mg/kg | Systemic injection | 24 h | D-Light P, SUPEREYE | 36 | 76 | 88.7% (63/71) | 0.1 cm | 1.3 cm | 1.43–11.9 | 3.29±1.81 (mean) |
Newton (102) | 2018 | Colon cancer lung metastases | VATS | ICG | 3 mg/kg | Systemic injection | 24 h | Iridium | 1 | NR | 100% (1/1) | 0.2 cm | NR | NR | NR |
Predina (103) | 2019 | Sarcomas lung metastasis | Thoracotomy | ICG | 5 mg/kg | Systemic injection | 24 h | Iridium | 10 | 16 | 87.5% (14/16) | 0.3 cm | 1.3 cm | 2.7–4.2 | NR |
VATS | ICG | 5 mg/kg | Systemic injection | 24 h | Iridium | 20 | 37 | 89.1% (33/37) | 0.2 cm | NR | 2.9±0.3 (mean) | NR | |||
Quan (104) | 2020 | Lung cancer | VATS | ICG | 0.75 mg/mL | Respiratory inhalation | 1 h | Pinpoint | 6 | 6 | 100% (6/6) | 0.2 cm | 1.0 cm | NR | NR |
Keating (108) | 2016 | Lung cancer | NR | EC17 | 0.1 mg/kg | Systemic injection | 2 h | NR | 3 | 3 | 100% (3/3) | 2 cm | NR | 3.3–3.6 | 5.2 |
Okusanya (105) | 2015 | Lung cancer | Thoracotomy | EC17 | 0.1 mg/kg | Systemic injection | 4 h | Artemis or FluoCam | 50 | 50 | 92.3% (48/52) | 0.3 cm | 0 | 2.0–14.7 | 0–19 |
Kennedy (110) | 2015 | Lung cancer | VATS | EC17 | 0.1 mg/kg | Systemic injection | 4 h | FluoCam | 30 | 30 | 100% (19/19) | 0.7 cm | 0 | 4.8–6.3 | 1.8–6.1 |
Keating (111) | 2017 | Lung cancer | Thoracotomy | OTL38 | 0.025 mg/kg | Systemic injection | 3 h | Karl Storz | 3 | 3 | 100% (3/3) | 3.4 cm | NR | 2.2–3.4 | NR |
Predina (112) | 2018 | Lung cancer | NR | OTL38 | 0.025 mg/kg | Systemic injection | 2.8–4.5 h | Iridium | 20 | 21 | 100% (24/24) | 0.1 cm | 0.39 cm (mean) | 2.1–4.2 | 2.8–10.0 |
Predina (113) | 2018 | Lung cancer | VATS | OTL38 | 0.025 mg/kg | Systemic injection | 3.4–5.1 h | Iridium | 12 | 13 | 69.2% (9/13) | 1.1 cm | 1.2 cm | 2.2–3.7 | 3.5–8.9 |
Predina (114) | 2017 | Lung cancer | MITS | OTL38 | 0.025 mg/kg | Systemic injection | 3–6 h | Iridium | 50 | 66 | 95.6% (65/68) | 0.2 cm | NR | 3.2±0.8 (mean) | 6.5±3.7 (mean) |
No., number; TBR, tumor-to-background fluorescence ratio; ICG, indocyanine green; VATS, video assisted thoracoscopic surgery; NR, not reported; MITS, minimally invasive thoracic surgery.
Limitations
There were several limitations to this review. First, good quality data was not available for some of the topics covered. Second, some relevant articles may have been missed. Third, a formal quality assessment of the included articles was not performed.
Summary
Currently, a variety of techniques are available for the identification of intraoperative pulmonary nodules. This article summarized the advantages and disadvantages of existing research methods and their clinical progress, so as to assist doctors in providing optimized clinical strategies. Clinicians can communicate with the multidisciplinary team and decide upon the appropriate positioning method according to each patient’s circumstances and the support of the existing equipment and technology of the institution. The various methods, instruments, and modalities described in this paper each have their own shortcomings and limitations (Tables 3,4), and further research to identify improved solutions is warranted.
Table 3
Modality | Method | Time to surgery | Advantages | Disadvantages | Complications |
---|---|---|---|---|---|
Hookwires | Percutaneous | ≤3 h | High success rate; easy to operate | Hookwire dislodgement; patient discomfort; immediate operation; difficult to locate pulmonary nodules in some areas | Pneumothorax; parenchymal hemorrhage; subcutaneous emphysema; retained; air embolism (rare) |
Microcoils | Percutaneous; ENB | Days | Well tolerated; easy to operate; flexibility with time to surgery | No direct mark on the pleural surface results; radiation exposure increased | Embolization; migration; parenchymal hematoma |
Hydrogel plugs or medical glue | Percutaneous; ENB | Weeks | Sealing up the needle track, decreasing the rate of puncture-related complications; non-toxic; good biosafety; long duration in body | Pungent smell | Irritating cough |
Methylene blue/PBV | Percutaneous; ENB | ≤3 h | Cheap and easy to get; no foreign body left in the lung; well tolerated; no anatomic limitation; no effect on pathology | Dye diffusion; immediate surgery; less reliable in anthracotic pigmentation lung | Anaphylaxis (rare) |
Lipiodol | Percutaneous; ENB | Months | Easy to operate; well tolerated; flexibility with time to surgery; well demarcation; no effect on pathology | Radiation exposure; pneumothorax; hemorrhaging in the lung parenchyma | Systemic embolization; allergy |
Barium | Percutaneous; ENB | Months | Easy to operate; well tolerated; stable and no diffusion; flexibility with time to surgery; easy to recognize | Effect on pathology; radiation exposure | Lung inflammation and pneumonia |
99mTc | Percutaneous; ENB; intravenous | ≤24 h | Easy to operate; well tolerated; deep detection | Spillage into the pleural; half-life 6 h; special equipment required; radiation exposure | Unremarkable |
ICG and target molecular fluorescent dye | Percutaneous; ENB | 24 h | Dyeing durable; safe; visible fluorescence; cheap and easy to get; no foreign body left in the lung; well tolerated; no anatomic limitation; no effect on pathology; easy to operate | Controlling the injection dose; complex imaging system needed; limited depth of penetration | Allergy (rare) |
Intravenous | 2–24 h | Detecting unidentified nodules | Only useful for pulmonary adenocarcinoma; high false positive rate | ||
Inhalation | 1 h | Only accumulation in the lung; convenient; application during the operation; low allergic reaction | Reverse imaging; complex imaging system needed | Unremarkable |
PBV, patent blue V; ICG, indocyanine green; ENB, electromagnetic navigation bronchoscopy.
Table 4
Instrument | Advantages | Disadvantages |
---|---|---|
CT | Easy to operate; short operation time; high success rate; low cost | Pneumothorax; parenchymal hemorrhage; subcutaneous emphysema; difficult to locate pulmonary nodules in some areas |
Bronchoscopy | Reduce pneumothorax and bleeding and can locate some positions that are difficult to puncture anatomically | Limited localization accuracy; complicated localization procedure; higher localization costs; pneumothorax; minor or moderate bleeding |
ENB | High success rate; safety | Expensive; complex operation process; high technical requirements |
VAL-MAP | Better determination of the surgical margin; safety; effectiveness | Expensive; complex operation process; high technical requirements; cumbersome process; immediate surgery is required |
AI | Reducing surgical fatigue; removing the lesions under direct vision | Expensive; high technical requirements; recognition error |
Hybrid operation room | Shorten the time period from localization to VATS; one anesthesia for the operations | Expensive; high technical requirements |
US | Safety; real time locating; without any material in lungs | Complete deflation of the lungs; normal lung parenchyma density nodules not identifiable; poor mobility and limited depth |
CT, computed tomography; ENB, electromagnetic navigation bronchoscopy; VAL-MAP, virtual assisted lung map; AI, artificial intelligence; US, ultrasound; VATS, video assisted thoracoscopic surgery.
This article provided a detailed summary of IMI, which is non-invasive, simple to operate, and does not increase the difficulty nor risk of surgery. However, all the associated target markers are nonspecific or target LUADs, and thus, the development of fluorescent nanomaterials with new targets may be the direction of future developments.
Acknowledgments
Funding: This study was supported by the Science and Technology Research Project of Jilin Provincial Department of Education (No. JJKH20211203KJ) and the Beijing Medical Award Foundation (No. YXJL-2020-0785-1108).
Footnote
Reporting Checklist: The authors have completed the Narrative Review reporting checklist. Available at https://qims.amegroups.com/article/view/10.21037/qims-22-309/rc
Conflicts of Interest: All authors have completed the ICMJE uniform disclosure form (available at https://qims.amegroups.com/article/view/10.21037/qims-22-309/coif). The authors have no conflicts of interest to declare.
Ethical Statement: The authors are accountable for all aspects of the work in ensuring that questions related to the accuracy or integrity of any part of the work are appropriately investigated and resolved.
Open Access Statement: This is an Open Access article distributed in accordance with the Creative Commons Attribution-NonCommercial-NoDerivs 4.0 International License (CC BY-NC-ND 4.0), which permits the non-commercial replication and distribution of the article with the strict proviso that no changes or edits are made and the original work is properly cited (including links to both the formal publication through the relevant DOI and the license). See: https://creativecommons.org/licenses/by-nc-nd/4.0/.
References
- Gould MK, Tang T, Liu IL, Lee J, Zheng C, Danforth KN, Kosco AE, Di Fiore JL, Suh DE. Recent Trends in the Identification of Incidental Pulmonary Nodules. Am J Respir Crit Care Med 2015;192:1208-14. [Crossref] [PubMed]
- Mazzone PJ, Lam L. Evaluating the Patient With a Pulmonary Nodule: A Review. JAMA 2022;327:264-73. [Crossref] [PubMed]
- Detterbeck FC, Lewis SZ, Diekemper R, Addrizzo-Harris D, Alberts WM. Executive Summary: Diagnosis and management of lung cancer, 3rd ed: American College of Chest Physicians evidence-based clinical practice guidelines. Chest 2013;143:7S-37S.
- Tamura M, Oda M, Fujimori H, Shimizu Y, Matsumoto I, Watanabe G. New indication for preoperative marking of small peripheral pulmonary nodules in thoracoscopic surgery. Interact Cardiovasc Thorac Surg 2010;11:590-3. [Crossref] [PubMed]
- Congedo MT, Iezzi R, Nachira D, Larici AR, Chiappetta M, Calandriello L, Vita ML, Meacci E, Porziella V, Ismail M, Manfredi R, Margaritora S. Uniportal VATS Coil-Assisted Resections for GGOs. J Oncol 2019;2019:5383086. [Crossref] [PubMed]
- Sui X, Zhao H, Yang F, Li JL, Wang J. Computed tomography guided microcoil localization for pulmonary small nodules and ground-glass opacity prior to thoracoscopic resection. J Thorac Dis 2015;7:1580-7. [PubMed]
- Xu X, Yao Y, Shen Y, Zhang P, Zhou J. Clinical Analysis of Percutaneous Computed Tomography-Guided Hook Wire Localization of 168 Small Pulmonary Nodules. Ann Thorac Surg 2015;100:1861-7. [Crossref] [PubMed]
- Lin MW, Tseng YH, Lee YF, Hsieh MS, Ko WC, Chen JY, Hsu HH, Chang YC, Chen JS. Computed tomography-guided patent blue vital dye localization of pulmonary nodules in uniportal thoracoscopy. J Thorac Cardiovasc Surg 2016;152:535-544.e2. [Crossref] [PubMed]
- Park CH, Han K, Hur J, Lee SM, Lee JW, Hwang SH, Seo JS, Lee KH, Kwon W, Kim TH, Choi BW. Comparative Effectiveness and Safety of Preoperative Lung Localization for Pulmonary Nodules: A Systematic Review and Meta-analysis. Chest 2017;151:316-28. [Crossref] [PubMed]
- Li C, Liu B, Jia H, Dong Z, Meng H. Computed tomography-guided hook wire localization facilitates video-assisted thoracoscopic surgery of pulmonary ground-glass nodules. Thorac Cancer 2018;9:1145-50. [Crossref] [PubMed]
- Mack MJ, Gordon MJ, Postma TW, Berger MS, Aronoff RJ, Acuff TE, Ryan WH. Percutaneous localization of pulmonary nodules for thoracoscopic lung resection. Ann Thorac Surg 1992;53:1123-4. [Crossref] [PubMed]
- Zhu J, Qu Y, Wang X, Jiang C, Mo J, Xi J, Wen Z. Risk factors associated with pulmonary hemorrhage and hemoptysis following percutaneous CT-guided transthoracic lung core needle biopsy: a retrospective study of 1,090 cases. Quant Imaging Med Surg 2020;10:1008-20. [Crossref] [PubMed]
- Fang X, Li J, Sun B, Liu M, Tang Z. Underestimated pulmonary hemorrhage-a fatal complication combined with systemic air embolism after CT-guided lung biopsy: a case description. Quant Imaging Med Surg 2021;11:4661-6. [Crossref] [PubMed]
- Kleedehn M, Kim DH, Lee FT, Lubner MG, Robbins JB, Ziemlewicz TJ, Hinshaw JL. Preoperative Pulmonary Nodule Localization: A Comparison of Methylene Blue and Hookwire Techniques. AJR Am J Roentgenol 2016;207:1334-9. [Crossref] [PubMed]
- Zhao G, Yu X, Chen W, Geng G, Li N, Liu H, Yin P, Sun L, Jiang J. Computed tomography-guided preoperative semi-rigid hook-wire localization of small pulmonary nodules: 74 cases report. J Cardiothorac Surg 2019;14:149. [Crossref] [PubMed]
- Sakiyama S, Kondo K, Matsuoka H, Yoshida M, Miyoshi T, Yoshida S, Monden Y. Fatal air embolism during computed tomography-guided pulmonary marking with a hook-type marker. J Thorac Cardiovasc Surg 2003;126:1207-9. [Crossref] [PubMed]
- Dendo S, Kanazawa S, Ando A, Hyodo T, Kouno Y, Yasui K, Mimura H, Akaki S, Kuroda M, Shimizu N, Hiraki Y. Preoperative localization of small pulmonary lesions with a short hook wire and suture system: experience with 168 procedures. Radiology 2002;225:511-8. [Crossref] [PubMed]
- Iguchi T, Hiraki T, Gobara H, Fujiwara H, Sugimoto S, Miyoshi S, Kanazawa S. Retained Short Hook Wires Used for Preoperative Localization of Small Pulmonary Lesions During Video-Assisted Thoracoscopic Surgery: A Report of 2 Cases. Cardiovasc Intervent Radiol 2015;38:1376-9. [Crossref] [PubMed]
- Yoshida Y, Inoh S, Murakawa T, Ota S, Fukayama M, Nakajima J. Preoperative localization of small peripheral pulmonary nodules by percutaneous marking under computed tomography guidance. Interact Cardiovasc Thorac Surg 2011;13:25-8. [Crossref] [PubMed]
- Zhang Z, Su T, Yu J, Cao S, Wang T, Yang S, Han Y, Wang H, Lu H, Tan Y, Jin L. Modified Microcoil for Preoperative Localization of Solitary Pulmonary Nodules: A Prospective, Single-Arm, Multicenter Clinical Study. J Vasc Interv Radiol 2021;32:1470-1477.e1. [Crossref] [PubMed]
- Sortini D, Feo C, Maravegias K, Carcoforo P, Pozza E, Liboni A, Sortini A. Intrathoracoscopic localization techniques. Review of literature. Surg Endosc 2006;20:1341-7. [Crossref] [PubMed]
- Ichinose J, Kohno T, Fujimori S, Harano T, Suzuki S. Efficacy and complications of computed tomography-guided hook wire localization. Ann Thorac Surg 2013;96:1203-8. [Crossref] [PubMed]
- Hanauer M, Perentes JY, Krueger T, Ris HB, Bize P, Schmidt S, Gonzalez M. Pre-operative localization of solitary pulmonary nodules with computed tomography-guided hook wire: report of 181 patients. J Cardiothorac Surg 2016;11:5. [Crossref] [PubMed]
- Sato M. Precise sublobar lung resection for small pulmonary nodules: localization and beyond. Gen Thorac Cardiovasc Surg 2020;68:684-91. [Crossref] [PubMed]
- Mayo JR, Clifton JC, Powell TI, English JC, Evans KG, Yee J, McWilliams AM, Lam SC, Finley RJ. Lung nodules: CT-guided placement of microcoils to direct video-assisted thoracoscopic surgical resection. Radiology 2009;250:576-85. [Crossref] [PubMed]
- Finley RJ, Mayo JR, Grant K, Clifton JC, English J, Leo J, Lam S. Preoperative computed tomography-guided microcoil localization of small peripheral pulmonary nodules: a prospective randomized controlled trial. J Thorac Cardiovasc Surg 2015;149:26-31. [Crossref] [PubMed]
- Liu L, Zhang LJ, Chen B, Cao JM, Lu GM, Yuan L, Li K, Xu J. Novel CT-guided coil localization of peripheral pulmonary nodules prior to video-assisted thoracoscopic surgery: a pilot study. Acta Radiol 2014;55:699-706. [Crossref] [PubMed]
- Xu Y, Ma L, Sun H, Huang Z, Zhang Z, Xiao F, Ma Q, Li C, Zhang X, Xie S. CT-guided microcoil localization for pulmonary nodules before VATS: a retrospective evaluation of risk factors for pleural marking failure. Eur Radiol 2020;30:5674-83. [Crossref] [PubMed]
- Rostambeigi N, Scanlon P, Flanagan S, Frank N, Talaie R, Andrade R, Golzarian J, Shrestha P CT. Fluoroscopic-Guided Coil Localization of Lung Nodules prior to Video-Assisted Thoracoscopic Surgical Resection Reduces Complications Compared to Hook Wire Localization. J Vasc Interv Radiol 2019;30:453-9. [Crossref] [PubMed]
- Xu Y, Ma L, Lin J, Sun H, Huang Z, Zhang Z, Xiao F, Ma Q. CT-guided microcoil localization for pulmonary nodules in the scapula-shadowed area before Video-Assisted Thoracic Surgery. Clin Respir J 2021;15:897-903. [Crossref] [PubMed]
- Hajjar W, Al-Nassar S, Almousa O, Rahal S, Al-Aqeed A, Ahmed I, Aboreida F. Thoracoscopic resection of suspected metastatic pulmonary nodules after microcoil localization technique: a prospective study. J Cardiovasc Surg (Torino) 2017;58:606-12. [Crossref] [PubMed]
- Ahrar JU, Gupta S, Ensor JE, Mahvash A, Sabir SH, Steele JR, McRae SE, Avritscher R, Huang SY, Odisio BC, Murthy R, Ahrar K, Wallace MJ, Tam AL. Efficacy of a Self-expanding Tract Sealant Device in the Reduction of Pneumothorax and Chest Tube Placement Rates After Percutaneous Lung Biopsy: A Matched Controlled Study Using Propensity Score Analysis. Cardiovasc Intervent Radiol 2017;40:270-6. [Crossref] [PubMed]
- Grage RA, Naveed MA, Keogh S, Wang D. Efficacy of a Dehydrated Hydrogel Plug to Reduce Complications Associated With Computed Tomography-guided Percutaneous Transthoracic Needle Biopsy. J Thorac Imaging 2017;32:57-62. [Crossref] [PubMed]
- Zaetta JM, Licht MO, Fisher JS, Avelar RL; Bio-Seal Study Group. A lung biopsy tract plug for reduction of postbiopsy pneumothorax and other complications: results of a prospective, multicenter, randomized, controlled clinical study. J Vasc Interv Radiol 2010;21:1235-43.e1-3.
- Imperatori A, Fontana F, Dominioni L, Piacentino F, Macchi E, Castiglioni M, Desio M, Cattoni M, Nardecchia E, Rotolo N. Video-assisted thoracoscopic resection of lung nodules localized with a hydrogel plug. Interact Cardiovasc Thorac Surg 2019;29:137-43. [Crossref] [PubMed]
- Qian K, Zhi X, Zhang Y, Li Y, Hu M, Li J, Shen C. Application of CT-guided localization with medical glue injection in treatment of ground-glass opacity and pulmonary nodules with video-assisted thoracoscope surgery. Journal of Capital Medical University 2015;36:529-32.
- Wang J, Gao LB, Zhang H, Wang J, Liu L, Shi WY. Preresection Stained Glue Injection to Localize Pulmonary Small Nodules and Ground-glass Opacities. J Thorac Imaging 2020;35:260-4. [Crossref] [PubMed]
- Yang SM, Yu KL, Lin KH, Liu YL, Sun SE, Meng LH, Ko HJ. Real-time augmented fluoroscopy-guided lung marking for thoracoscopic resection of small pulmonary nodules. Surg Endosc 2020;34:477-84. [Crossref] [PubMed]
- Ng CSH, Man Chu C, Kwok MWT, Yim APC, Wong RHL. Hybrid DynaCT scan-guided localization single-port lobectomy. Chest 2015;147:e76-e78. [corrected]. [Crossref] [PubMed]
- Stanzi A, Mazza F, Lucio F, Ghirardo D, Grosso M, Locatelli A, Melloni G. Tailored intraoperative localization of non-palpable pulmonary lesions for thoracoscopic wedge resection using hybrid room technology. Clin Respir J 2018;12:1661-7. [Crossref] [PubMed]
- Lin CY, Chang CC, Huang LT, Chung TJ, Liu YS, Yen YT, Tseng YL. Computed Tomography-Guided Methylene Blue Localization: Single vs. Multiple Lung Nodules. Front Med (Lausanne) 2021;8:661956. [Crossref] [PubMed]
- Velasquez R, Martin A, Abu Hishmeh M, DeLorenzo L, Dhillon SS, Harris K. Placement of markers to assist minimally invasive resection of peripheral lung lesions. Ann Transl Med 2019;7:360. [Crossref] [PubMed]
- Vandoni RE, Cuttat JF, Wicky S, Suter M. CT-guided methylene-blue labelling before thoracoscopic resection of pulmonary nodules. Eur J Cardiothorac Surg 1998;14:265-70. [Crossref] [PubMed]
- Wu TT, Chang YC, Lee JM, Hung MH. Anaphylactic reaction to patent blue V used in preoperative computed tomography-guided dye localization of small lung nodules. J Formos Med Assoc 2016;115:288-9. [Crossref] [PubMed]
- McConnell PI, Feola GP, Meyers RL. Methylene blue-stained autologous blood for needle localization and thoracoscopic resection of deep pulmonary nodules. J Pediatr Surg 2002;37:1729-31. [Crossref] [PubMed]
- Yoshida R, Yoshizako T, Tanaka S, Ando S, Nakamura M, Kishimoto K, Kitagaki H. CT-guided color marking of impalpable pulmonary nodules prior to video-assisted thoracoscopic surgery. Clin Imaging 2021;74:84-8. [Crossref] [PubMed]
- Tseng YH, Lee YF, Hsieh MS, Chien N, Ko WC, Chen JY, Lee JM, Huang PM, Lin MW, Chen JS, Chang YC. Preoperative computed tomography-guided dye injection to localize multiple lung nodules for video-assisted thoracoscopic surgery. J Thorac Dis 2016;8:S666-71. [Crossref] [PubMed]
- Tsai TM, Chiang XH, Liao HC, Tsou KC, Lin MW, Chen KC, Hsu HH, Chen JS. Computed tomography-guided dye localization for deeply situated pulmonary nodules in thoracoscopic surgery. Ann Transl Med 2019;7:31. [Crossref] [PubMed]
- Tsuchida M, Yamato Y, Aoki T, Watanabe T, Koizumi N, Emura I, Hayashi J. CT-guided agar marking for localization of nonpalpable peripheral pulmonary lesions. Chest 1999;116:139-43. [Crossref] [PubMed]
- Mogi A, Yajima T, Tomizawa K, Onozato R, Tanaka S, Kuwano H. Video-Assisted Thoracoscopic Surgery after Preoperative CT-Guided Lipiodol Marking of Small or Impalpable Pulmonary Nodules. Ann Thorac Cardiovasc Surg 2015;21:435-9. [Crossref] [PubMed]
- Watanabe K, Nomori H, Ohtsuka T, Kaji M, Naruke T, Suemasu K. Usefulness and complications of computed tomography-guided lipiodol marking for fluoroscopy-assisted thoracoscopic resection of small pulmonary nodules: experience with 174 nodules. J Thorac Cardiovasc Surg 2006;132:320-4. [Crossref] [PubMed]
- Miura H, Yamagami T, Tanaka O, Yoshimatsu R, Ichijo Y, Kato D, Shimada J. CT findings after lipiodol marking performed before video-assisted thoracoscopic surgery for small pulmonary nodules. Acta Radiol 2016;57:303-10. [Crossref] [PubMed]
- Lee NK, Park CM, Kang CH, Jeon YK, Choo JY, Lee HJ, Goo JM. CT-guided percutaneous transthoracic localization of pulmonary nodules prior to video-assisted thoracoscopic surgery using barium suspension. Korean J Radiol 2012;13:694-701. [Crossref] [PubMed]
- Ricciardi S, Davini F, Manca G, De Liperi A, Romano G, Zirafa CC, Melfi F. Radioguided Surgery, a Cost-Effective Strategy for Treating Solitary Pulmonary Nodules: 20-Year Experience of a Single Center. Clin Lung Cancer 2020;21:e417-22. [Crossref] [PubMed]
- Ambrogi MC, Melfi F, Zirafa C, Lucchi M, De Liperi A, Mariani G, Fanucchi O, Mussi A. Radio-guided thoracoscopic surgery (RGTS) of small pulmonary nodules. Surg Endosc 2012;26:914-9. [Crossref] [PubMed]
- Nagai K, Kuriyama K, Inoue A, Yoshida Y, Takami K. Computed tomography-guided preoperative localization of small lung nodules with indocyanine green. Acta Radiol 2018;59:830-5. [Crossref] [PubMed]
- Zhang C, Lin H, Fu R, Zhang T, Nie Q, Dong S, Yang XN, Wu YL, Zhong WZ. Application of indocyanine green fluorescence for precision sublobar resection. Thorac Cancer 2019;10:624-30. [Crossref] [PubMed]
- Zhang B, Peng M, Yu F, Mei X, Tang J, Wang X, Liu W, Chen C, Chen X. A novel technique for preoperative localization of pulmonary nodules using a mixture of tissue adhesive and iohexol under computed tomography guidance: A 140 patient single-center study. Thorac Cancer 2021;12:854-63. [Crossref] [PubMed]
- Jiang T, Lin M, Zhao M, Zhan C, Li M, Feng M, Wang Q. Preoperative Computed Tomography-Guided Localization for Pulmonary Nodules with Glue and Dye. Thorac Cardiovasc Surg 2020;68:525-32. [Crossref] [PubMed]
- Doo KW, Yong HS, Kim HK, Kim S, Kang EY, Choi YH. Needlescopic resection of small and superficial pulmonary nodule after computed tomographic fluoroscopy-guided dual localization with radiotracer and hookwire. Ann Surg Oncol 2015;22:331-7. [Crossref] [PubMed]
- Wang YZ, Boudreaux JP, Dowling A, Woltering EA. Percutaneous localisation of pulmonary nodules prior to video-assisted thoracoscopic surgery using methylene blue and TC-99. Eur J Cardiothorac Surg 2010;37:237-8. [Crossref] [PubMed]
- Brady JJ, Hirsch Reilly C, Guay R, Dasika U. Combined Hookwire and Methylene Blue Localization of Pulmonary Nodules: Analysis of 74 Patients. Innovations (Phila) 2018;13:184-9. [Crossref] [PubMed]
- Asano F, Shindoh J, Shigemitsu K, Miya K, Abe T, Horiba M, Ishihara Y. Ultrathin bronchoscopic barium marking with virtual bronchoscopic navigation for fluoroscopy-assisted thoracoscopic surgery. Chest 2004;126:1687-93. [Crossref] [PubMed]
- Asano F, Matsuno Y, Ibuka T, Takeichi N, Oya H. A barium marking method using an ultrathin bronchoscope with virtual bronchoscopic navigation. Respirology 2004;9:409-13. [Crossref] [PubMed]
- Okumura T, Kondo H, Suzuki K, Asamura H, Kobayashi T, Kaneko M, Tsuchiya R. Fluoroscopy-assisted thoracoscopic surgery after computed tomography-guided bronchoscopic barium marking. Ann Thorac Surg 2001;71:439-42. [Crossref] [PubMed]
- Ng CSH, Zhao Z, Long H, Lau RWH. Electromagnetic Navigation Bronchoscopy Triple Contrast Dye Marking for Lung Nodule Localization. Thorac Cardiovasc Surg 2020;68:253-5. [Crossref] [PubMed]
- Bowling MR, Folch EE, Khandhar SJ, Arenberg DA, Awais O, Minnich DJ, Pritchett MA, Rickman OB, Sztejman E, Anciano CJ. Pleural dye marking of lung nodules by electromagnetic navigation bronchoscopy. Clin Respir J 2019;13:700-7. [Crossref] [PubMed]
- Anayama T, Qiu J, Chan H, Nakajima T, Weersink R, Daly M, McConnell J, Waddell T, Keshavjee S, Jaffray D, Irish JC, Hirohashi K, Wada H, Orihashi K, Yasufuku K. Localization of pulmonary nodules using navigation bronchoscope and a near-infrared fluorescence thoracoscope. Ann Thorac Surg 2015;99:224-30. [Crossref] [PubMed]
- Bolton WD, Howe H 3rd, Stephenson JE. The utility of electromagnetic navigational bronchoscopy as a localization tool for robotic resection of small pulmonary nodules. Ann Thorac Surg 2014;98:471-5; discussion 475-6. [Crossref] [PubMed]
- Krimsky WS, Minnich DJ, Cattaneo SM, Sarkar SA, Harley DP, Finley DJ, Browning RF, Parrish SC. Thoracoscopic detection of occult indeterminate pulmonary nodules using bronchoscopic pleural dye marking. J Community Hosp Intern Med Perspect 2014; [Crossref] [PubMed]
- Abbas A, Kadakia S, Ambur V, Muro K, Kaiser L. Intraoperative electromagnetic navigational bronchoscopic localization of small, deep, or subsolid pulmonary nodules. J Thorac Cardiovasc Surg 2017;153:1581-90. [Crossref] [PubMed]
- Miyoshi T, Kondo K, Takizawa H, Kenzaki K, Fujino H, Sakiyama S, Tangoku A. Fluoroscopy-assisted thoracoscopic resection of pulmonary nodules after computed tomography--guided bronchoscopic metallic coil marking. J Thorac Cardiovasc Surg 2006;131:704-10. [Crossref] [PubMed]
- Toba H, Kondo K, Miyoshi T, Kajiura K, Yoshida M, Kawakami Y, Takizawa H, Kenzaki K, Sakiyama S, Tangoku A. Fluoroscopy-assisted thoracoscopic resection after computed tomography-guided bronchoscopic metallic coil marking for small peripheral pulmonary lesions. Eur J Cardiothorac Surg 2013;44:e126-32. [Crossref] [PubMed]
- Sato M, Shinohara Y, Yanagiya M, Karasaki T, Kitano K, Nagayama K, Nakajima J. Use of electromagnetic navigation bronchoscopy in virtual-assisted lung mapping: the effect of on-site adjustment. Gen Thorac Cardiovasc Surg 2019;67:1062-9. [Crossref] [PubMed]
- Marino KA, Sullivan JL, Weksler B. Electromagnetic Navigation Bronchoscopy for Identifying Lung Nodules for Thoracoscopic Resection. Ann Thorac Surg 2016;102:454-7. [Crossref] [PubMed]
- Song G, Qiu T, Xuan Y, Zhao Y, Jiao W. Clinical Application of Vectorial Localization of Peripheral Pulmonary Nodules Guided by Electromagnetic Navigation Bronchoscopy in Thoracic Surgery. Zhongguo Fei Ai Za Zhi 2019;22:709-13. [PubMed]
- Awais O, Reidy MR, Mehta K, Bianco V, Gooding WE, Schuchert MJ, Luketich JD, Pennathur A. Electromagnetic Navigation Bronchoscopy-Guided Dye Marking for Thoracoscopic Resection of Pulmonary Nodules. Ann Thorac Surg 2016;102:223-9. [Crossref] [PubMed]
- Tay JH, Wallbridge PD, Larobina M, Russell PA, Irving LB, Steinfort DP. Electromagnetic Navigation Bronchoscopy-directed Pleural Tattoo to Aid Surgical Resection of Peripheral Pulmonary Lesions. J Bronchology Interv Pulmonol 2016;23:245-50. [Crossref] [PubMed]
- Zhang W, Chen S, Dong X, Lei P. Meta-analysis of the diagnostic yield and safety of electromagnetic navigation bronchoscopy for lung nodules. J Thorac Dis 2015;7:799-809. [PubMed]
- Sato M, Omasa M, Chen F, Sato T, Sonobe M, Bando T, Date H. Use of virtual assisted lung mapping (VAL-MAP), a bronchoscopic multispot dye-marking technique using virtual images, for precise navigation of thoracoscopic sublobar lung resection. J Thorac Cardiovasc Surg 2014;147:1813-9. [Crossref] [PubMed]
- Sato M, Kuwata T, Yamanashi K, Kitamura A, Misawa K, Imashimizu K, Kobayashi M, Ikeda M, Koike T, Kosaka S, Fukai R, Sekine Y, Isowa N, Hirayama S, Sakai H, Watanabe F, Nagayama K, Aoyama A, Date H, Nakajima J. Safety and reproducibility of virtual-assisted lung mapping: a multicentre study in Japan. Eur J Cardiothorac Surg 2017;51:861-8. [Crossref] [PubMed]
- Sato M, Nagayama K, Kuwano H, Nitadori JI, Anraku M, Nakajima J. Role of post-mapping computed tomography in virtual-assisted lung mapping. Asian Cardiovasc Thorac Ann 2017;25:123-30. [Crossref] [PubMed]
- Sato M, Kobayashi M, Kojima F, Tanaka F, Yanagiya M, Kosaka S, Fukai R, Nakajima J. Effect of virtual-assisted lung mapping in acquisition of surgical margins in sublobar lung resection. J Thorac Cardiovasc Surg 2018;156:1691-1701.e5. [Crossref] [PubMed]
- Sato M, Nagayama K, Kobayashi M, Nakajima J. Virtual-Assisted Lung Mapping 2.0: Preoperative Bronchoscopic Three-Dimensional Lung Mapping. Ann Thorac Surg 2019;108:269-73. [Crossref] [PubMed]
- Kondo R, Yoshida K, Hamanaka K, Hashizume M, Ushiyama T, Hyogotani A, Kurai M, Kawakami S, Fukushima M, Amano J. Intraoperative ultrasonographic localization of pulmonary ground-glass opacities. J Thorac Cardiovasc Surg 2009;138:837-42. [Crossref] [PubMed]
- Matsumoto S, Hirata T, Ogawa E, Fukuse T, Ueda H, Koyama T, Nakamura T, Wada H. Ultrasonographic evaluation of small nodules in the peripheral lung during video-assisted thoracic surgery (VATS). Eur J Cardiothorac Surg 2004;26:469-73. [Crossref] [PubMed]
- Mattioli S, D'Ovidio F, Daddi N, Ferruzzi L, Pilotti V, Ruffato A, Bolzani R, Gavelli G. Transthoracic endosonography for the intraoperative localization of lung nodules. Ann Thorac Surg 2005;79:443-9; discussion 443-9. [Crossref] [PubMed]
- Khereba M, Ferraro P, Duranceau A, Martin J, Goudie E, Thiffault V, Liberman M. Thoracoscopic localization of intraparenchymal pulmonary nodules using direct intracavitary thoracoscopic ultrasonography prevents conversion of VATS procedures to thoracotomy in selected patients. J Thorac Cardiovasc Surg 2012;144:1160-5. [Crossref] [PubMed]
- Piolanti M, Coppola F, Papa S, Pilotti V, Mattioli S, Gavelli G. Ultrasonographic localization of occult pulmonary nodules during video-assisted thoracic surgery. Eur Radiol 2003;13:2358-64. [Crossref] [PubMed]
- Lachkar S, Baste JM, Thiberville L, Peillon C, Rinieri P, Piton N, Guisier F, Salaun M. Pleural Dye Marking Using Radial Endobronchial Ultrasound and Virtual Bronchoscopy before Sublobar Pulmonary Resection for Small Peripheral Nodules. Respiration 2018;95:354-61. [Crossref] [PubMed]
- Ost DE, Ernst A, Lei X, Kovitz KL, Benzaquen S, Diaz-Mendoza J, et al. Diagnostic Yield and Complications of Bronchoscopy for Peripheral Lung Lesions. Results of the AQuIRE Registry. Am J Respir Crit Care Med 2016;193:68-77. [Crossref] [PubMed]
- Fu R, Chai YF, Zhang JT, Zhang T, Chen XK, Dong S, Yan HH, Yang XN, Huang MP, Wu YL, Zhuang J, Zhong WZ. Three-dimensional printed navigational template for localizing small pulmonary nodules: A case-controlled study. Thorac Cancer 2020;11:2690-7. [Crossref] [PubMed]
- Zhang L, Wang L, Kadeer X, Zeyao L, Sun X, Sun W, et al. Accuracy of a 3-Dimensionally Printed Navigational Template for Localizing Small Pulmonary Nodules: A Noninferiority Randomized Clinical Trial. JAMA Surg 2019;154:295-303. [Crossref] [PubMed]
- Jensen K, Hansen HJ, Petersen RH, Neckelmann K, Vad H, Møller LB, Pedersen JH, Konge L. Evaluating competency in video-assisted thoracoscopic surgery (VATS) lobectomy performance using a novel assessment tool and virtual reality simulation. Surg Endosc 2019;33:1465-73. [Crossref] [PubMed]
- Ujiie H, Yamaguchi A, Gregor A, Chan H, Kato T, Hida Y, Kaga K, Wakasa S, Eitel C, Clapp TR, Yasufuku K. Developing a virtual reality simulation system for preoperative planning of thoracoscopic thoracic surgery. J Thorac Dis 2021;13:778-83. [Crossref] [PubMed]
- Qin Z, Tai Y, Xia C, Peng J, Huang X, Chen Z, Li Q, Shi J. Towards Virtual VATS, Face, and Construct Evaluation for Peg Transfer Training of Box, VR, AR, and MR Trainer. J Healthc Eng 2019;2019:6813719. [Crossref] [PubMed]
- Peng M, Yu L, Zhou Y, Yang Y, Luo Q, Cheng X. Augmented reality-assisted localization of solitary pulmonary nodules for precise sublobar lung resection: a preliminary study using an animal model. Transl Lung Cancer Res 2021;10:4174-84. [Crossref] [PubMed]
- Perkins SL, Krajancich B, Yang CJ, Hargreaves BA, Daniel BL, Berry MF. A Patient-Specific Mixed-Reality Visualization Tool for Thoracic Surgical Planning. Ann Thorac Surg 2020;110:290-5. [Crossref] [PubMed]
- Okusanya OT, Holt D, Heitjan D, Deshpande C, Venegas O, Jiang J, Judy R, DeJesus E, Madajewski B, Oh K, Wang M, Albelda SM, Nie S, Singhal S. Intraoperative near-infrared imaging can identify pulmonary nodules. Ann Thorac Surg 2014;98:1223-30. [Crossref] [PubMed]
- Kim HK, Quan YH, Choi BH, Park JH, Han KN, Choi Y, Kim BM, Choi YH. Intraoperative pulmonary neoplasm identification using near-infrared fluorescence imaging. Eur J Cardiothorac Surg 2016;49:1497-502. [Crossref] [PubMed]
- Mao Y, Chi C, Yang F, Zhou J, He K, Li H, Chen X, Ye J, Wang J, Tian J. The identification of sub-centimetre nodules by near-infrared fluorescence thoracoscopic systems in pulmonary resection surgeries. Eur J Cardiothorac Surg 2017;52:1190-6. [Crossref] [PubMed]
- Newton AD, Predina JD, Frenzel-Sulyok LG, Shin MH, Wang Y, Singhal S. Intraoperative near-infrared imaging can identify sub-centimeter colorectal cancer lung metastases during pulmonary metastasectomy. J Thorac Dis 2018;10:E544-8. [Crossref] [PubMed]
- Predina JD, Newton AD, Corbett C, Shin M, Sulfyok LF, Okusanya OT, Delikatny EJ, Nie S, Gaughan C, Jarrar D, Pechet T, Kucharczuk JC, Singhal S. Near-infrared intraoperative imaging for minimally invasive pulmonary metastasectomy for sarcomas. J Thorac Cardiovasc Surg 2019;157:2061-9. [Crossref] [PubMed]
- Quan YH, Oh CH, Jung D, Lim JY, Choi BH, Rho J, Choi Y, Han KN, Kim BM, Kim C, Park JH, Kim HK. Evaluation of Intraoperative Near-Infrared Fluorescence Visualization of the Lung Tumor Margin With Indocyanine Green Inhalation. JAMA Surg 2020;155:732-40. [Crossref] [PubMed]
- Okusanya OT, DeJesus EM, Jiang JX, Judy RP, Venegas OG, Deshpande CG, Heitjan DF, Nie S, Low PS, Singhal S. Intraoperative molecular imaging can identify lung adenocarcinomas during pulmonary resection. J Thorac Cardiovasc Surg 2015;150:28-35.e1. [Crossref] [PubMed]
- Low PS, Kularatne SA. Folate-targeted therapeutic and imaging agents for cancer. Curr Opin Chem Biol 2009;13:256-62. [Crossref] [PubMed]
- O'Shannessy DJ, Yu G, Smale R, Fu YS, Singhal S, Thiel RP, Somers EB, Vachani A. Folate receptor alpha expression in lung cancer: diagnostic and prognostic significance. Oncotarget 2012;3:414-25. [Crossref] [PubMed]
- Keating JJ, Okusanya OT, De Jesus E, Judy R, Jiang J, Deshpande C, Nie S, Low P, Singhal S. Intraoperative Molecular Imaging of Lung Adenocarcinoma Can Identify Residual Tumor Cells at the Surgical Margins. Mol Imaging Biol 2016;18:209-18. [Crossref] [PubMed]
- Predina JD, Okusanya O. D Newton A, Low P, Singhal S. Standardization and Optimization of Intraoperative Molecular Imaging for Identifying Primary Pulmonary Adenocarcinomas. Mol Imaging Biol 2018;20:131-8. [Crossref] [PubMed]
- Kennedy GT, Okusanya OT, Keating JJ, Heitjan DF, Deshpande C, Litzky LA, Albelda SM, Drebin JA, Nie S, Low PS, Singhal S. The Optical Biopsy: A Novel Technique for Rapid Intraoperative Diagnosis of Primary Pulmonary Adenocarcinomas. Ann Surg 2015;262:602-9. [Crossref] [PubMed]
- Keating JJ, Runge JJ, Singhal S, Nims S, Venegas O, Durham AC, Swain G, Nie S, Low PS, Holt DE. Intraoperative near-infrared fluorescence imaging targeting folate receptors identifies lung cancer in a large-animal model. Cancer 2017;123:1051-60. [Crossref] [PubMed]
- Predina JD, Newton AD, Keating J, Dunbar A, Connolly C, Baldassari M, Mizelle J, Xia L, Deshpande C, Kucharczuk J, Low PS, Singhal S. A Phase I Clinical Trial of Targeted Intraoperative Molecular Imaging for Pulmonary Adenocarcinomas. Ann Thorac Surg 2018;105:901-8. [Crossref] [PubMed]
- Predina JD, Newton AD, Xia L, Corbett C, Connolly C, Shin M, Sulyok LF, Litzky L, Deshpande C, Nie S, Kularatne SA, Low PS, Singhal S. An open label trial of folate receptor-targeted intraoperative molecular imaging to localize pulmonary squamous cell carcinomas. Oncotarget 2018;9:13517-29. [Crossref] [PubMed]
- Predina JD, Newton AD, Keating J, Barbosa EM Jr, Okusanya O, Xia L, Dunbar A, Connolly C, Baldassari MP, Mizelle J, Delikatny EJ, Kucharczuk JC, Deshpande C, Kularatne SA, Low P, Drebin J, Singhal S. Intraoperative Molecular Imaging Combined With Positron Emission Tomography Improves Surgical Management of Peripheral Malignant Pulmonary Nodules. Ann Surg 2017;266:479-88. [Crossref] [PubMed]