Determination of pulmonary vessel alteration in Chinese male smokers by quantitative computed tomography measurements: a retrospective study
Introduction
Cigarette smoking is one of the biggest threats to public health and is an entirely preventable cause of cardiopulmonary morbidity and mortality (1). Cigarette smoke is a significant risk factor for many lung diseases, including chronic obstructive pulmonary disease (COPD) (2), interstitial lung diseases (3,4), and lung cancer (5). The impact of cigarette smoke on the pulmonary vasculature is not well understood. However, pulmonary vascular remodeling in smokers may be integral to the pathway from healthy lungs to advanced lung disease. Both animal models and human studies have elucidated the effects of tobacco on pulmonary vessels and its pathological manifestations, including endothelial inflammation (6), smooth muscle proliferation (7,8), and intimal thickening (9). These pathologies result in vasoconstriction and vessel occlusion.
Current approaches for assessing pulmonary vasculature, such as histologic examination and right heart catheterization (RHC), are invasive, limiting large-scale assessments. The advent of computer-aided image analysis of computed tomography (CT) scans has offered a non-invasive lens into the pulmonary blood vessels. Measurements of the total blood volume in all intraparenchymal vessels have been reported and proven to be associated with tobacco exposure. In the Multi-Ethnic Study of Atherosclerosis (MESA) study (10), ever-smokers (people who used to smoke but quit smoking for more than one year) had higher pulmonary vascular volumes than never-smokers. Similarly, tobacco exposure was associated with high absolute pulmonary vessel volume in the Framingham Heart Study (11). However, the MESA study did not screen for smoking or lung diseases. Although the Framingham Heart Study was conducted in a large cohort of community-dwelling and generally healthy adults and many confounders (age, height, weight, pack-years, occupation, etc.) were adjusted for in their model, they did not consider the critical effect of the lung itself on pulmonary blood vessels.
We hypothesized that cigarette smoking is associated with the pulmonary vascular volume. However, we aimed to assess this association in a generally healthy male population after adjusting for related demographic data and lung parameters, such as lung volume (LV), density, and total emphysema. Additionally, we planned to evaluate the dynamic changes in pulmonary vessel volume in a longitudinal cohort over a 2- to 3-year follow-up period. We present this article in accordance with the STROBE reporting checklist (available at https://qims.amegroups.com/article/view/10.21037/qims-23-1758/rc).
Methods
Study cohort and design
The study was conducted in accordance with the Declaration of Helsinki (as revised in 2013). All participants were fully informed of the nature of the study and provided written informed consent for participation. The Ethics Committee of Xi’an Jiaotong University approved this study (No. 2013-114-1), which was registered with the Chinese Clinical Trials Registry Center (http://www.chictr.org/en/; No. ChiCTR-OCH-14004935). All methods were performed according to relevant guidelines.
Male subjects admitted to the Department of Physical Examination of the First Affiliated Hospital of Xi’an Jiaotong University for annual health assessment between February 2017 and February 2018 were enrolled in this retrospective study. The inclusion criteria were as follows: (I) male subjects who underwent a non-contrast chest CT scan; (II) a complete history of smoking, including smoking, years of smoking, smoking index (SI), smoking cessation, and years of smoking cessation; and (III) age between 18 and 80 years. The exclusion criteria were: (I) subjects with a congenital deformity of the spine or thorax (n=7); (II) subjects with a history of lobectomy or pneumonectomy (n=14); (III) subjects who could not provide a complete history of smoking (n=24); (IV) subjects with noticeable respiratory or motion artifact (n=25); (V) chest CT examinations that did not include the apex or the base of lung (n=7); (VI) subjects with apparent lesions in the CT scan, such as the diffused emphysema, lobar consolidation, nodules or mass of the lung, active tuberculosis or tuberculosis involving multiple pulmonary lobes, lung atelectasis, interstitial lung diseases or pleura effusion (n=86); and (VII) subjects with severe heart, liver, and kidney dysfunction (n=8).
The baseline characteristics included age (years), height (cm), and weight (kg). “Non-smokers” were defined as those who had never smoked. “Current smokers” were defined as subjects who smoked more than one cigarette a day for more than six months, and “former smokers” were defined as subjects who had quit smoking more than one year at baseline and did not start smoking again during the whole observation time. Smoking was quantified using the SI, which was defined by multiplying the number of packs per day by the years of smoking.
Therefore, we classified the participants into three baseline subgroups: non-smokers (n=311), current smokers (n=267), and former smokers (n=78). Then, the cohort was subgrouped according to the age. The median and the maximal age of our cohort was 60 and 80 years old, and people below 40 years old did not perform an annual health assessment with a chest CT scan. Thus, four age groups were separated using a 10-year span, including groups ≤50 years old, >50 and ≤60 years old, >60 and ≤70 years old, and >70 years old. Additionally, we planned to evaluate the dynamic changes in pulmonary vessel volume in a longitudinal cohort over a 2- to 3-year follow-up period. One hundred and fifty-two persons had follow-up data, including 70 non-smokers, 55 current smokers, and 27 former smokers. The study selection is presented in a flow diagram (Figure 1).
CT scan
Non-contrast inspiratory chest CT was performed in the supine position using a 64-detector-row scanner (Gemini TF PET/CT; Philips, Netherlands). The scan scope was set from the apex to the base of the lungs. All subjects underwent breathing training before the CT examination. The scan parameters were 120 KVp, automatic current with a range of 100–300 mA (based on body weight), exposure time of 0.5 s, 0.625 mm slice thickness, and matrix size of 512×512 pixels. Images were reconstructed with a 1-mm slice thickness (0.625 mm overlap) using full iterative reconstruction technique.
Quantitative CT (QCT) parameters assessment of the lung, pulmonary vessels, and the emphysema
Image analyses were performed at the First Affiliated Hospital of Xi’an Jiaotong University using software based on the FACT Lung Workstation (Dexin, Xi’an, China, http://www.dexhin.com/). A three-dimensional approach was employed to segment the region of bilateral lungs, and the intrapulmonary vessels (both arteries and veins) with tubal shape and higher density were segmented with computational geometric methods, as previously described (12,13). Measurements of the total volume of all intrapulmonary vessels (IPVV) were computed from all the segmented intrapulmonary vessels. In the meantime, the voxels with a density lower than −950 HU were identified, and the total volume of low-attenuation area <−950 Hounsfield unit (LAA-950) and its ratio to the entire lung (LAA-950%) were calculated. The density of each lung voxel was collected and averaged as the value of mean lung density (MLD) (HU, Hounsfield unit) (Figure 2). Two experienced radiologists (L.J. and C.S.) with >10 years of experience in thoracic image interpretation reviewed the CT images independently. The results of the segmentation were allowed for manual correction when the results were not satisfactory. Any disagreement between the two radiologists was resolved by another experienced radiologist (Y.G.).
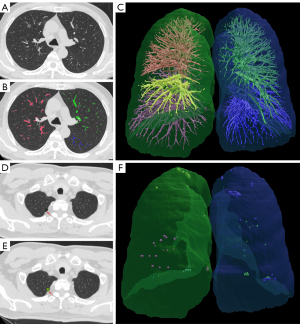
Statistical analysis
All statistical analyses were performed using SPSS (SPSS, version 25.0; Chicago, USA). Descriptive statistics were expressed as mean ± standard deviation (SD) for continuous variables with normal distribution or median with interquartile range (IQR) for continuous variables without normal distribution. Analysis of variance (ANOVA) was used to assess the differences in essential clinical characteristics and QCT parameters among non-smokers, current smokers, and former smokers. Afterward, the multiple comparisons between the two groups were conducted using the least significant difference (LSD) for the equal variance or by Tamhane T2 for the unequal variance. Subgroups analysis of ages was performed for the clinical and QCT parameters.
The correlation heat map using R language (v 4.3.1) showed correlations among age, height, weight, BMI, LV, LAA-950, LAA-950%, MLD, IPVV, and SI. Linear regression was used to estimate the association between IPVV (independent variable) and SI (dependent variable) using adjusted models. The model was adjusted for age, height, weight, body mass index (BMI), LV, LAA-950, LAA-950%, and MLD. A paired t-test was used to evaluate the differences in QCT parameters between the initial and follow-up CT scans. A two-sided P value <0.05 was considered significant for all statistical analyses.
Results
Clinical characteristics and the QCT measurements of the subjects
The study selection is presented in a flow diagram (Figure 1). The participants had a median age of 60 years, a median height of 172 cm, and a median weight of 74 kg. The median SI of the current and former smokers was 20 packs/year (Table 1).
Table 1
Groups | Items | Non-smokers | Current smokers | Former smokers | F | P |
---|---|---|---|---|---|---|
All cases | Number of cases | 311 | 267 | 78 | ||
Age (years) | 59 (53–67) | 60 (52–65) | 61.25±6.63 | 1.34 | 0.26 | |
Height (cm) | 172 (170–176) | 172 (170–175.5) | 172 (170–176) | 0.849 | 0.42 | |
Weight (kg) | 73 (66–80) | 74 (67–81) | 73.83±8.62 | 1.635 | 0.19 | |
BMI (kg/m2) | 24.49±2.50 | 24.82±2.95 | 24.89±2.71 | 1.356 | 0.25 | |
SI (pack/year) | NA | 20 (15.0–36.75) | 20 (10–30) | −0.989 | 0.32 | |
Duration of cessation (years) | NA | NA | 5 (2–12.75) | – | – | |
IPVV (mL) | 120.76±25.52 | 134.62±23.96* | 130.79±25.13* | 23.074 | <0.001 | |
LV (mL) | 5,200.27±904.40 | 5,287.95±911.34 | 5,165.65±903.69 | 0.907 | 0.40 | |
LAA-950 (mL) | 29.70 (13.42–56.43) | 19.74 (8.76–43.44) | 29.40 (13.16–71.06) | 0.019 | 0.98 | |
LAA-950% (%) | 0.57 (0.28–1.07) | 0.36 (0.18–0.75) | 0.59 (0.25–1.25) | 0.011 | 0.98 | |
MLD (HU) | −834.80 (−846.92 to −813.50) | −825.30 (−841.94 to −806.56)* | −826.74±29.02 | 5.864 | 0.003 | |
Age, years | ||||||
≤50 | Number of cases | 39 | 45 | 6 | ||
Age (years) | 45.41±3.61 | 45 (42–50) | 44.50±3.88 | 0.172 | 0.84 | |
Height (cm) | 173 (171–178) | 172.98±5.32 | 172.17±3.54 | 0.875 | 0.42 | |
Weight (kg) | 75.30±9.35 | 75.86±11.62 | 78.50±3.50 | 0.261 | 0.77 | |
BMI (kg/m2) | 24.75±2.45 | 25.33±3.60 | 26.49±1.19 | 0.999 | 0.37 | |
SI (pack/year) | NA | 16.96±9.90 | 10.83±5.16 | 1.479 | 0.14 | |
Duration of cessation (years) | NA | NA | 5 (2–15) | – | – | |
IPVV (mL) | 128.10±19.53 | 133.29 (123.17–142.14) | 165.82±25.62* | 8.031 | 0.001 | |
LV (mL) | 5,446.07±752.07 | 5,075.31±789.81* | 4,452.96±877.79* | 5.279 | 0.007 | |
LAA-950 (mL) | 28.88 (19.78–37.20) | 15.49 (7.94–24.84) | 5.25±5.11*† | 2.296 | 0.107 | |
LAA-950% (%) | 0.53(0.37–0.70) | 0.32 (0.18–0.64) | 0.47 (0.16–1.13)*† | 2.753 | 0.06 | |
MLD (HU) | −832.82±20.63 | −819.94±24.53* | −783.07±39.70*† | 11.827 | <0.001 | |
>50 and ≤60 | Number of cases | 141 | 92 | 32 | ||
Age (years) | 56 (53–59) | 56 (52–58) | 57 (55–59) | 1.338 | 0.26 | |
Height (cm) | 172 (170–176) | 173.5 (171–177) | 173.5 (172–176) | 1.112 | 0.33 | |
Weight (kg) | 74.29±8.05 | 75 (70–83) | 73.71±9.28 | 2.733 | 0.06 | |
BMI (kg/m2) | 24.80±2.30 | 25.32±2.51 | 24.61±3.05 | 1.629 | 0.19 | |
SI (pack/year) | NA | 20 (15–30) | 20 (10–30) | −0.049 | 0.96 | |
Duration of cessation (years) | NA | NA | 5 (2–15) | – | – | |
IPVV (mL) | 127.33 (111.67–139.22) | 138.64±22.43* | 133.53±22.06 | 6.169 | 0.002 | |
LV (mL) | 5,125.42±1004.87 | 5,435.04±935.06* | 5,309.98±936.75 | 2.84 | 0.06 | |
LAA-950 (mL) | 24.02 (9.89–39.96) | 16.83 (9.28–41.67) | 22.60 (8.34–67.17) | 0.059 | 0.94 | |
LAA-950% (%) | 0.45 (0.20–0.81) | 0.32 (0.18–0.64) | 0.47 (0.16–1.13) | 0.026 | 0.94 | |
MLD (HU) | −831.99 (−845.67 to −810.28) | −821.26±27.54 | −829.77±24.43 | 1.678 | 0.18 | |
>60 and ≤70 | Number of cases | 74 | 94 | 28 | ||
Age (years) | 64 (62–67.25) | 63.5 (62–66) | 64.89±3.01 | 0.562 | 0.57 | |
Height (cm) | 172 (169–175.2) | 172 (170–175) | 172.35±5.39 | 0.114 | 0.89 | |
Weight (kg) | 72.08±9.11 | 73.72±9.63 | 74.46±7.93 | 0.96 | 0.38 | |
BMI (kg/m2) | 24.32±2.59 | 24.79±2.86 | 25.06±2.34 | 1.015 | 0.36 | |
SI (pack/year) | NA | 30 (16–40) | 20 (10–30) | −2.673 | 0.008 | |
Duration of cessation (years) | NA | NA | 5 (1–10) | – | – | |
IPVV (mL) | 109.91 (94.06–130.98) | 135.68±23.84* | 125.65±21.91* | 19.217 | <0.001 | |
LV (mL) | 5,251.90±857.84 | 5,291.76±896.24 | 5,185.42±857.82 | 0.166 | 0.84 | |
LAA-950 (mL) | 36.36 (18.21–67.58) | 20.03 (7.15–51.06) | 35.13 (19.72–84.87) | 0.546 | 0.58 | |
LAA-950% (%) | 0.67 (0.37–1.40) | 0.37 (0.14–0.84) | 0.66 (0.39–1.29) | 0.671 | 0.51 | |
MLD (HU) | −837.58 (−848.56 to −814.00) | −821.93 ±28.26* | −833.27±23.62† | 4.796 | 0.009 | |
>70 | Number of cases | 57 | 36 | 12 | F | P |
Age (years) | 74 (71–76) | 75.19±2.63 | 74.93±2.84 | 1.925 | 0.15 | |
Height (cm) | 170 (168–174) | 171.11±5.16 | 170.43±5.02 | 0.583 | 0.56 | |
Weight (kg) | 68.70±8.94 | 67.38±9.29 | 68.64±9.78 | 0.251 | 0.77 | |
BMI (kg/m2) | 23.78±2.79 | 22.95±2.70 | 23.67±3.46 | 0.886 | 0.41 | |
SI (pack/year) | NA | 25.00 (15.00–40.00) | 42.00±24.39 | −1.794 | 0.07 | |
Duration of cessation (years) | NA | NA | 11.50±10.31 | – | – | |
IPVV (mL) | 110.23±23.05 | 120.13±23.30* | 117.58±23.42 | 2.126 | 0.12 | |
LV (mL) | 5,150.19±773.35 | 5,167.90±949.77 | 5,091.01±861.72 | 0.037 | 0.96 | |
LAA-950 (mL) | 41.44 (26.13–81.72) | 25.96 (17.26–115.68) | 62.21(39.90–196.32) | 1.411 | 0.24 | |
LAA-950% (%) | 0.83 (0.49–1.52) | 0.50 (0.35–2.26) | 1.19 (0.88–3.95) | 1.379 | 0.25 | |
MLD (HU) | −831.61±21.97 | −830.18±26.94 | −837.21 (−844.82 to −807.95) | 0.324 | 0.72 |
Data are presented as mean ± standard deviation or median (interquartile range). *, significantly compared with non-smokers; †, significantly compared with smokers. BMI, body mass index; SI, smoking index; IPVV, intrapulmonary vessel volume; LV, lung volume; LAA-950, low-attenuation area <−950 Hounsfield unit; MLD, mean lung density; HU, Hounsfield unit; NA, not applicable.
There were no significant differences in age, height, weight, and BMI among the three groups. The IPVV of current smokers was significantly more extensive than that of non-smokers (134.62±23.96 vs. 120.76±25.52 mL, mean difference =13.86±2.07 mL, P<0.001). Similarly, the IPVV of former smokers was significantly more extensive than that of non-smokers (130.79±25.13 vs. 120.76±25.52 mL, mean difference =10.03±3.15 mL, P=0.003). However, there was no significant difference in IPVV between the group of current smokers and former smokers (mean difference =3.83±3.19 mL, P=0.23).
The MLD of current smokers was higher than that of non-smokers (Z= 3.305, P=0.001), and the MLD of former smokers was higher than that of non-smokers, although the difference was not statistically significant (Z=0.390, P=0.69). The LAA-950 and LAA-950% of current smokers were lower than those of non-smokers without significance (P=0.99, P=0.92). The LAA-950 and LAA-950% were comparable between the non-smokers and former smokers (P=0.29, P=0.22).
In individuals aged ≤50, current smokers with a mean SI of 16.96±9.90 pack/year had higher IPVVs than non-smokers, but the difference was not statistically significant. However, the LV was reduced and the MLD increased significantly in current smokers compared to non-smokers (P=0.03, P=0.01). Only six former smokers were identified in this age group. The IPVV and MLD of former smokers were significantly higher than those of their control counterparts (P<0.001, P=0.03); on the other hand, the LV, LAA-950, and LAA-950% were significantly lower than those of their control counterparts (P=0.005, P<0.001, P<0.001).
In the group of participants aged >50 and ≤60, current smokers with a median SI of 20 pack/year had significantly higher IPVV and LV than non-smokers (P<0.001, P=0.02). For the group of participants aged >60 and ≤70 years, the SI of current smokers was significantly higher than that of former smokers (P=0.008), and both current and former smokers had a larger IPVV than that of non-smokers (P<0.001, P=0.01). Additionally, the MLD of current smokers was higher than that of non-smokers, and the significance was in the group aged ≤50 and the group aged >60 and ≤70 years (P=0.01, P=0.006).
Correlation of the QCT parameters and the SIs
The correlation heat map shows that IPVV was negatively correlated with age, LAA-950, and LAA-950% (r=−0.26, P<0.001; r=−0.19, P<0.001; r=−0.22, P<0.001) and positively correlated with height, weight, BMI, LV, MLD, years of smoking, and SI (r=0.29, P<0.001; r=0.29, P<0.001; r=0.19, P<0.001; r=0.11, P=0.006; r=0.42, P<0.001; r=0.20, P<0.001; and r=0.19, P<0.001). LAA-950 was positively correlated with age (r=0.19, P<0.001) and negatively associated with weight, BMI, and MLD (r=−0.19, P<0.001; r=−0.21, P<0.001; and r=−0.50, P<0.001) (Figure 3).
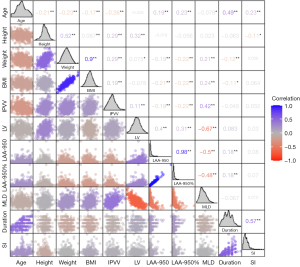
After adjusting for age, height, weight, BMI, LV, LAA-950, LAA-950%, and MLD, a higher cigarette smoking level was associated with greater IPVV [non-standardized coefficient: 0.167, 95% confidence interval (CI): 0.086–0.248] (Table 2).
Table 2
Items | Non-standardized coefficient | Standardized coefficient | T (95% CI) | P |
---|---|---|---|---|
Intercept | 733.616 | – | 26.837 (679.938, 787.294) | <0.001 |
Age (years) | −0.532 | −0.192 | −6.721 (−0.687, −0.376) | <0.001 |
LV (mL) | 0.019 | 0.662 | 17.173 (0.017, 0.021) | <0.001 |
MLD (HU) | 0.814 | 0.838 | 21.481 (0.739, 0.888) | <0.001 |
SI | 0.167 | 0.116 | 4.048 (0.086, 0.248) | <0.001 |
IPVV, intrapulmonary vessel volume; SI, smoking index; CI, confidence interval; LV, lung volume; MLD, mean lung density; HU, Hounsfield unit.
Longitudinal changes of QCT parameters
In the longitudinal comparisons, the IPVV of non-smokers and former smokers in the follow-up scan showed no significant increase or decrease compared with the baseline scan (P=0.79, P=0.81). However, the IPVV of the follow-up scan significantly increased for current smokers compared with that of the baseline scan (P=0.02), as shown in Table 3.
Table 3
Groups | Items | Baseline CT scan | Follow-up CT scan | T | P |
---|---|---|---|---|---|
Non-smokers | Number of cases | 70 | 70 | – | – |
Age (years) | 58.5 (54–68) | 60.5 (56–68) | −7.881 | <0.001 | |
Height (cm) | 172.5±5.27 | 172.65±5.02 | −0.990 | 0.32 | |
Weight (kg) | 74.5±8.85 | 74.06±8.82 | 1.018 | 0.31 | |
BMI (kg/m2) | 25.00±2.51 | 24.80±2.42 | 1.321 | 0.19 | |
SI (pack/year) | NA | NA | – | – | |
IPVV (mL) | 121.66±30.33 | 121.09±29.76 | 0.273 | 0.78 | |
LV (mL) | 5,057.18±898.47 | 5,136.53±1,046.06 | −1.565 | 0.12 | |
LAA-950 (mL) | 27.41 (13.52–46.13) | 29.48 (14.97–51.03) | −1.695 | 0.09 | |
LAA-950% (%) | 0.53 (0.29–0.91) | 0.53 (0.32–1.00) | −1.798 | 0.07 | |
MLD (HU) | −825.33±25.77 | −829.38±26.54 | 2.298 | 0.02 | |
Current smokers | Number of cases | 55 | 55 | ||
Age (years) | 61.58±6.84 | 63.40±6.89 | −26.31 | <0.001 | |
Height (cm) | 173 (171–175) | 173 (171–175) | 1.000 | 0.32 | |
Weight (kg) | 74.96±9.10 | 74.94±9.11 | 1.000 | 0.32 | |
BMI (kg/m2) | 24.82±2.60 | 24.82±2.60 | 1.000 | 0.32 | |
SI (pack/year) | 20 (15–40) | 23 (15.75–42) | −9.487 | <0.001 | |
IPVV (mL) | 129.73±29.75 | 135.49±28.60 | −2.326 | 0.02 | |
LV (mL) | 5,451.63±996.11 | 5,558.20±1,010.97 | −1.434 | 0.15 | |
LAA-950 (mL) | 18.81 (10.05–45.80) | 18.32 (9.40–49.61) | −1.995 | 0.05 | |
LAA-950% (%) | 0.33 (0.21–0.82) | 0.32 (0.17–0.91) | −2.104 | 0.04 | |
MLD (HU) | −823.07±25.86 | −822.76±26.47 | −0.136 | 0.89 | |
Former smokers | Number of cases | 27 | 27 | – | – |
Age (years) | 60 (58–70) | 62 (60.5–72.5) | −5.355 | <0.001 | |
Height (cm) | 171.44±4.65 | 171.48±4.54 | 0.002 | >0.99 | |
Weight (kg) | 72.52±8.10 | 73.15±7.19 | −1.785 | 0.07 | |
BMI (kg/m2) | 24.70±2.84 | 24.92±2.58 | −2.066 | 0.03 | |
SI (pack/year) | 20 (10–30) | 20 (10–30) | – | – | |
IPVV (mL) | 124.63±24.07 | 123.86±23.53 | 0.238 | 0.81 | |
LV (mL) | 5,182.49±995.74 | 5,144.21±1,022.76 | 0.353 | 0.72 | |
LAA-950 (mL) | 22.99 (9.98–53.66) | 37.24 (11.06–77.73) | −0.756 | 0.45 | |
LAA-950% (%) | 0.46 (0.22–0.99) | 0.71 (0.26–1.48) | −1.130 | 0.26 | |
MLD (HU) | −828.83±30.31 | −831.74±27.23 | 0.731 | 0.47 |
Data are presented as mean ± standard deviation or median (interquartile range). QCT, quantitative CT; CT, computed tomography; BMI, body mass index; SI, smoking index; IPVV, intrapulmonary vessel volume; LV, lung volume; LAA-950, low-attenuation area <−950 Hounsfield unit; MLD, mean lung density; HU, Hounsfield unit; NA, not applicable.
The MLD of non-smokers decreased significantly in the follow-up scan compared to that in the baseline scan (P=0.03). Meanwhile, the MLD of the follow-up scans for current and former smokers showed no significant changes compared to the baseline scan (P=0.89, P=0.47), as shown in Table 3. Table 3 also shows that there was an increase in LAA-950% in the follow-up scan for current smokers (P=0.04), whereas there was no increase in the follow-up scan for both non-smokers and former smokers (P=0.08, P=0.27).
Discussion
Chronic exposure to cigarette smoke can result in the remodeling of the pulmonary vasculature, emphysematous destruction of the lung parenchyma (14), and lung inflammation (5). This study aimed to investigate the effects of chronic exposure to cigarette smoke on pulmonary vasculature, lung parenchyma, and lung inflammation in a Chinese male population. IPVV, MLD, LAA-950, and LAA-950% were quantified using a three-dimensional (3D) algorithm based on non-contrast CT images. All CT scans in our study were performed using the same scanner at a single center and using a uniform protocol, thus reducing the systematic bias of the QCT parameters.
Several exciting results were obtained. First, current smokers had the highest IPVV and MLD, followed by ex-smokers and non-smokers. The LAA-950 and LAA-950% of current smokers were lower than those of non-smokers and former smokers, although the difference was not statistically significant. Second, IPVV correlated with age, height, weight, BMI, LV, LAA-950, LAA-950%, MLD, and cigarette exposure (quantified by SI). After adjusting for demographic data and lung parameters, cigarette exposure also positively affected IPVV. Third, IPVV continued to increase in current smokers in the follow-up CT scan, but not in non-smokers and former smokers. This suggests that pulmonary vascular volumes detectable by CT are associated with cigarette exposure and quitting smoking may at least slow or prevent remodeling of the pulmonary vasculature. To the best of our knowledge, this is the first in vivo study describing the relationship between IPVV infection and smoking status.
Although the original recruitment strategy targeted residents of the town of Xi’an, the Han Chinese population, our findings are consistent with those of other studies. Cigarette exposure was also associated with higher pulmonary blood vessel volumes in the Framingham Heart (11,15), MESA (10), and COPD Gene studies (16). A previous study also confirmed the association between the smoking and the pulmonary blood volumes (17). However, none of these studies considered the effects of lung size and density on IPVV. As shown in the correlation map, a higher IPVV was correlated with a higher total LV, higher MLD, and lower age. The computational geometric method of pulmonary vessel segmentation is initially based on density, and this image analysis technique cannot differentiate the volume of the vessel wall from the volume of blood (i.e., the volume of the vessel lumen). Thus, the increase in the MLD may lead to higher blood vessel volume. The LV expansion was also positively correlated with IPVV, which is a compliance change of the IPVV in the LV expansion. However, in the linear regression analysis, after adjusting for age, total LV, and MLD, cigarette smoke had a positive effect on IPVV.
Histologically, tobacco-related vasculopathy is a vascular remodeling process that includes the marked proliferation of pulmonary artery smooth muscle cells that contribute to vascular wall hypertrophy and vascular lumen narrowing due to the production of vasoconstrictors and vasoproliferative agents (18). However, vascular lumen narrowing and vasoconstriction mainly affect microvessels (19) with a cross-sectional area of less than 1 mm2, and vascular congestion upstream of these microvessels may increase IPVV. Another explanation is that hypoxic pulmonary vasoconstriction is blocked in the presence of inflammation, thus allowing perfusion maintenance in inflamed regions (20-22). In an animal study, carbon monoxide burned from the cigarette was shown to induce vasodilation via nitric oxide-mediated mechanisms (23), which may be another explanation for the positive relationship.
In the sub-group analysis, the difference in IPVV between current smokers and non-smokers was not significant in the group younger than 50 years old, and this may be due to the less cumulative effect of cigarette smoking. The LV of current smokers decreased in the group younger than 50 years old and increased in the group of participants aged >60 and ≤70 years significantly compared with non-smokers (P<0.05). This may be due to the small sample of the study. Smoking-related lung diseases are broad spectrum, such as COPD, lung cancer, and interstitial lung diseases. COPD is often manifested as lung expansion and interstitial lung diseases lead to the reduction of LV. Thus, these results need to be proved in larger cohorts and in longer follow-up duration.
In the correlation analysis, the SI is positively associated with larger IPVV and higher MLD, but not with the LAA-950 and LAA-950%, presumably reflecting that the increased level of pulmonary inflammation is the main pathological change of smoking. Emphysema is thought to occur owing to lung inflammation. LAA-950 and LAA-950% decreased in current smokers compared to non-smokers, although the difference was not statistically significant. Previously, studies have proved that there is less emphysema in current smokers compared with former smokers visually (24) and quantitatively (25). However, the conclusion was drawn from the COPD cohort. In our study, the recruitment strategy targeted residents of the town, and the male subjects performed a chest CT examination mainly for an annual health assessment, not the lung disease evaluation. Thus, the insignificant can be reasonable. The MLD correlated negatively with LAA-950 and LAA-950%, which means that higher MLD prevents the detection of lower attenuation.
This study has several limitations. First, as a single-center retrospective study, although our models were adjusted for related demographic data and lung parameters, residual confounders (such as second-hand tobacco exposure, socioeconomic status, occupation, the clinical history of hypertension and diabetes) could not be excluded. Second, it should be noted that male participants who presented for routine health checkups may differ from the broader population of China. Third, as the IPVVs evaluated by CT do not distinguish between the pulmonary artery and pulmonary vein, the effects of smoking on the pulmonary arteries, veins, and microvasculature cannot be independently evaluated using CT. Fourth, this was a longitudinal observational study, and too many cases were lost to follow-up. Few follow-up patients may prevent us from the more clinically significant conclusions, like malignant or non-malignant pulmonary diseases. Now, we are trying to include more chest CT examinations to give more definite and convincing findings. As so few patients can stop smoking permanently, we may need to follow up for a longer duration.
Conclusions
In conclusion, pulmonary vascular volumes detectable by non-contrast CT are associated with cigarette exposure and cessation of smoking. Terminate smoking may slow or prevent the remodeling of the pulmonary vasculature.
Acknowledgments
Funding: This work was supported by
Footnote
Reporting Checklist: The authors have completed the STROBE reporting checklist. Available at https://qims.amegroups.com/article/view/10.21037/qims-23-1758/rc
Conflicts of Interest: All authors have completed the ICMJE uniform disclosure form (available at https://qims.amegroups.com/article/view/10.21037/qims-23-1758/coif). The authors have no conflicts of interest to declare.
Ethical Statement: The authors are accountable for all aspects of the work in ensuring that questions related to the accuracy or integrity of any part of the work are appropriately investigated and resolved. The study was conducted in accordance with the Declaration of Helsinki (as revised in 2013). Approval was granted by the Ethics Committee of Xi’an Jiaotong University (No. 2013-114-1). All participants were fully informed of the nature of the study and provided written informed consent for participation.
Open Access Statement: This is an Open Access article distributed in accordance with the Creative Commons Attribution-NonCommercial-NoDerivs 4.0 International License (CC BY-NC-ND 4.0), which permits the non-commercial replication and distribution of the article with the strict proviso that no changes or edits are made and the original work is properly cited (including links to both the formal publication through the relevant DOI and the license). See: https://creativecommons.org/licenses/by-nc-nd/4.0/.
References
- Münzel T, Hahad O, Kuntic M, Keaney JF, Deanfield JE, Daiber A. Effects of tobacco cigarettes, e-cigarettes, and waterpipe smoking on endothelial function and clinical outcomes. Eur Heart J 2020;41:4057-70. [Crossref] [PubMed]
- Hattori N, Nakagawa T, Yoneda M, Hayashida H, Nakagawa K, Yamamoto K, Htun MW, Shibata Y, Koji T, Ito T. Compounds in cigarette smoke induce EGR1 expression via the AHR, resulting in apoptosis and COPD. J Biochem 2022;172:365-76. [Crossref] [PubMed]
- Lederer DJ, Enright PL, Kawut SM, Hoffman EA, Hunninghake G, van Beek EJ, Austin JH, Jiang R, Lovasi GS, Barr RG. Cigarette smoking is associated with subclinical parenchymal lung disease: the Multi-Ethnic Study of Atherosclerosis (MESA)-lung study. Am J Respir Crit Care Med 2009;180:407-14. [Crossref] [PubMed]
- Sangani RG, Deepak V, Ghio AJ, Forte MJ, Zulfikar R, Patel Z, King A, Alshaikhnassir E, Abbas G, Vos J. Interstitial lung abnormalities and interstitial lung diseases associated with cigarette smoking in a rural cohort undergoing surgical resection. BMC Pulm Med 2022;22:172. [Crossref] [PubMed]
- Bade BC, Dela Cruz CS. Lung Cancer 2020: Epidemiology, Etiology, and Prevention. Clin Chest Med 2020;41:1-24. [Crossref] [PubMed]
- Xue H, Xie B, Xu N, Li H, Chen Q, Xie W, Wang H. Etanercept Protected Against Cigarette Smoke Extract-Induced Inflammation and Apoptosis of Human Pulmonary Artery Endothelial Cells via Regulating TNFR1. Int J Chron Obstruct Pulmon Dis 2021;16:1329-45. [Crossref] [PubMed]
- Ohshima Y, Iwata K, Ibi M, Matsumoto M, Katsuyama M, Yabe-Nishimura C. Nicotine and methyl vinyl ketone, major components of cigarette smoke extracts, increase protective amyloid-β peptides in cells harboring amyloid-β precursor protein. J Toxicol Sci 2018;43:257-66. [Crossref] [PubMed]
- Sevilla-Montero J, Labrousse-Arias D, Fernández-Pérez C, Fernández-Blanco L, Barreira B, Mondéjar-Parreño G, Alfaro-Arnedo E, López IP, Pérez-Rial S, Peces-Barba G, Pichel JG, Peinado VI, Cogolludo Á, Calzada MJ. Cigarette Smoke Directly Promotes Pulmonary Arterial Remodeling and Kv7.4 Channel Dysfunction. Am J Respir Crit Care Med 2021;203:1290-305. [Crossref] [PubMed]
- Wang J, Wang L, Chen X, Liang ML, Wei DH, Cao W, Zhang J. Cigarette smoke extract stimulates human pulmonary artery smooth muscle cell proliferation: Role of inflammation and oxidative stress. Iran J Basic Med Sci 2022;25:755-61. [Crossref] [PubMed]
- Aaron CP, Hoffman EA, Lima JAC, Kawut SM, Bertoni AG, Vogel-Claussen J, et al. Pulmonary vascular volume, impaired left ventricular filling, and dyspnea: The MESA Lung Study. Plos One 2017;12:e0176180. [Crossref] [PubMed]
- Synn AJ, Zhang C, Washko GR, Estépar RSJ, O'Connor GT, Li W, Mittleman MA, Rice MB. Cigarette Smoke Exposure and Radiographic Pulmonary Vascular Morphology in the Framingham Heart Study. Ann Am Thorac Soc 2019;16:698-706. [Crossref] [PubMed]
- Zhao W, Liu H, Leader JK, Wilson D, Meng X, Wang L, Chen LA, Pu J. Computerized identification of the vasculature surrounding a pulmonary nodule. Comput Med Imaging Graph 2019;74:1-9. [Crossref] [PubMed]
- Pu J, Fuhrman C, Good WF, Sciurba FC, Gur D. A differential geometric approach to automated segmentation of human airway tree. IEEE Trans Med Imaging 2011;30:266-78. [Crossref] [PubMed]
- Vij N, Chandramani-Shivalingappa P, Van Westphal C, Hole R, Bodas M. Cigarette smoke-induced autophagy impairment accelerates lung aging, COPD-emphysema exacerbations and pathogenesis. Am J Physiol Cell Physiol 2018;314:C73-87. [Crossref] [PubMed]
- Synn AJ, Li W, San José Estépar R, Zhang C, Washko GR, O'Connor GT, Araki T, Hatabu H, Bankier AA, Mittleman MA, Rice MB. Radiographic pulmonary vessel volume, lung function and airways disease in the Framingham Heart Study. Eur Respir J 2019;54:1900408. [Crossref] [PubMed]
- Estépar RS, Kinney GL, Black-Shinn JL, Bowler RP, Kindlmann GL, Ross JC, et al. Computed tomographic measures of pulmonary vascular morphology in smokers and their clinical implications. Am J Respir Crit Care Med 2013;188:231-9. [Crossref] [PubMed]
- Xing AP, Du YC, Hu XY, Xu JY, Zhang HP, Li Y, Nie X. Cigarette smoke extract stimulates rat pulmonary artery smooth muscle cell proliferation via PKC-PDGFB signaling. J Biomed Biotechnol 2012;2012:534384. [Crossref] [PubMed]
- Sun X, Meng X, Zhang P, Wang L, Ren Y, Xu G, Yang T, Liu M. Quantification of pulmonary vessel volumes on low-dose computed tomography in a healthy male Chinese population: the effects of aging and smoking. Quant Imaging Med Surg 2022;12:406-16. [Crossref] [PubMed]
- Ferrer E, Peinado VI, Castañeda J, Prieto-Lloret J, Olea E, González-Martín MC, Vega-Agapito MV, Díez M, Domínguez-Fandos D, Obeso A, González C, Barberà JA. Effects of cigarette smoke and hypoxia on pulmonary circulation in the guinea pig. Eur Respir J 2011;38:617-27. [Crossref] [PubMed]
- Blanco I, Piccari L, Barberà JA. Pulmonary vasculature in COPD: The silent component. Respirology 2016;21:984-94. [Crossref] [PubMed]
- Gust R, Kozlowski J, Stephenson AH, Schuster DP. Synergistic hemodynamic effects of low-dose endotoxin and acute lung injury. Am J Respir Crit Care Med 1998;157:1919-26. [Crossref] [PubMed]
- Jahn N, Lamberts RR, Busch CJ, Voelker MT, Busch T, Koel-Simmelink MJ, Teunissen CE, Oswald DD, Loer SA, Kaisers UX, Weimann J. Inhaled carbon monoxide protects time-dependently from loss of hypoxic pulmonary vasoconstriction in endotoxemic mice. Respir Res 2015;16:119. [Crossref] [PubMed]
- Thorup C, Jones CL, Gross SS, Moore LC, Goligorsky MS. Carbon monoxide induces vasodilation and nitric oxide release but suppresses endothelial NOS. Am J Physiol 1999;277:F882-9. [Crossref] [PubMed]
- Jou SS, Yagihashi K, Zach JA, Lynch D, Suh YJ. COPDGene Investigators. Relationship between current smoking, visual CT findings and emphysema index in cigarette smokers. Clin Imaging 2019;53:195-9. [Crossref] [PubMed]
- Zach JA, Williams A, Jou SS, Yagihashi K, Everett D, Hokanson JE, Stinson D, Lynch DA. COPDGene Investigators. Current Smoking Status Is Associated With Lower Quantitative CT Measures of Emphysema and Gas Trapping. J Thorac Imaging 2016;31:29-36. [Crossref] [PubMed]